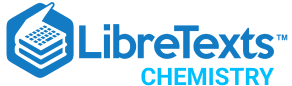
- school Campus Bookshelves
- menu_book Bookshelves
- perm_media Learning Objects
- login Login
- how_to_reg Request Instructor Account
- hub Instructor Commons

Margin Size
- Download Page (PDF)
- Download Full Book (PDF)
- Periodic Table
- Physics Constants
- Scientific Calculator
- Reference & Cite
- Tools expand_more
- Readability
selected template will load here
This action is not available.
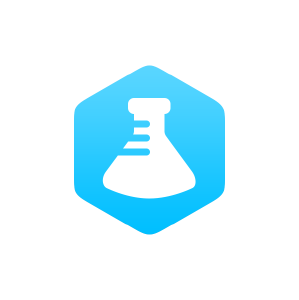
1: Chemical Kinetics - The Method of Initial Rates (Experiment)
- Last updated
- Save as PDF
- Page ID 95999
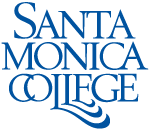
- Santa Monica College
\( \newcommand{\vecs}[1]{\overset { \scriptstyle \rightharpoonup} {\mathbf{#1}} } \)
\( \newcommand{\vecd}[1]{\overset{-\!-\!\rightharpoonup}{\vphantom{a}\smash {#1}}} \)
\( \newcommand{\id}{\mathrm{id}}\) \( \newcommand{\Span}{\mathrm{span}}\)
( \newcommand{\kernel}{\mathrm{null}\,}\) \( \newcommand{\range}{\mathrm{range}\,}\)
\( \newcommand{\RealPart}{\mathrm{Re}}\) \( \newcommand{\ImaginaryPart}{\mathrm{Im}}\)
\( \newcommand{\Argument}{\mathrm{Arg}}\) \( \newcommand{\norm}[1]{\| #1 \|}\)
\( \newcommand{\inner}[2]{\langle #1, #2 \rangle}\)
\( \newcommand{\Span}{\mathrm{span}}\)
\( \newcommand{\id}{\mathrm{id}}\)
\( \newcommand{\kernel}{\mathrm{null}\,}\)
\( \newcommand{\range}{\mathrm{range}\,}\)
\( \newcommand{\RealPart}{\mathrm{Re}}\)
\( \newcommand{\ImaginaryPart}{\mathrm{Im}}\)
\( \newcommand{\Argument}{\mathrm{Arg}}\)
\( \newcommand{\norm}[1]{\| #1 \|}\)
\( \newcommand{\Span}{\mathrm{span}}\) \( \newcommand{\AA}{\unicode[.8,0]{x212B}}\)
\( \newcommand{\vectorA}[1]{\vec{#1}} % arrow\)
\( \newcommand{\vectorAt}[1]{\vec{\text{#1}}} % arrow\)
\( \newcommand{\vectorB}[1]{\overset { \scriptstyle \rightharpoonup} {\mathbf{#1}} } \)
\( \newcommand{\vectorC}[1]{\textbf{#1}} \)
\( \newcommand{\vectorD}[1]{\overrightarrow{#1}} \)
\( \newcommand{\vectorDt}[1]{\overrightarrow{\text{#1}}} \)
\( \newcommand{\vectE}[1]{\overset{-\!-\!\rightharpoonup}{\vphantom{a}\smash{\mathbf {#1}}}} \)
- To determine the rate law of a chemical reaction using the Method of Initial Rates.
- To determine the activation energy of the reaction by finding the value of the rate constant, \(k\), at several temperatures.
- To observe the effect of a catalyst on the reaction rate.
Part A: Finding the Rate Law Using the Method of Initial Rates
The rate law of a chemical reaction is a mathematical equation that describes how the reaction rate depends upon the concentration of each reactant. Two methods are commonly used in the experimental determination of the rate law: the method of initial rates and the graphical method . In this experiment, we shall use the method of initial rates to determine the rate law of a reaction. You should review the sections on determining rate laws via this method in the chapter on chemical kinetics in your textbook before proceeding with this experiment.
The reaction to be studied in this experiment is represented by the following balanced chemical equation:
\[\ce{6I^{-}( aq ) + BrO3^{-}( aq ) + 6H^{+}( aq )->3I2( aq ) + Br^{-}( aq ) + 3H2O( l )} \label{1}\]
This reaction proceeds relatively slowly. The rate law for this reaction is of the form:
\[\text{Rate} = k [\ce{I^{-}}]^{x}[\ce{BrO3^{-}}]^{y}[\ce{H^{+}}]^{z} \label{2}\]
where the value of the rate constant, \(k\), is dependent upon the temperature at which the reaction is run. The values of \(x\), \(y\), \(z\) and \(k\) must be found for this reaction in order to specify the rate law completely. The values of the reaction orders, \(x\), \(y\), and \(z\), are usually, though not always, small integers.
The method of initial rates allows the values of these reaction orders to be found by running the reaction multiple times under controlled conditions and measuring the rate of the reaction in each case. All variables are held constant from one run to the next, except for the concentration of one reactant. The order of that reactant concentration in the rate law can be determined by observing how the reaction rate varies as the concentration of that one reactant is varied. This method is repeated for each reactant until all the orders are determined. At that point, the rate law can be used to find the value of \(k\) for each trial. If the temperatures are the same for each trial, then the values of \(k\) should be the same too.
The rate of the reaction can be defined as the rate of decrease of the concentration of \( \ce{BrO3^{-}}\):
\[\text{Rate of Reaction} = - \frac{ \Delta \ce{[BrO3^{-}]}}{\Delta t} \label{3}\]
Note that this is actually the average rate of the reaction over the time interval \(\Delta t\) since the concentration of \( \ce{BrO3^{-}}\) and therefore the rate of the reaction is continuously decreasing during \(\Delta t\). In this experiment we can assume that \(\Delta \ce{[BrO3^{-}]}\) is negligible compared to \( [\ce{BrO3^{-}}]\) thus allowing us to approximate our measured average rate as being equal to the initial, instantaneous rate.
The rate of the reaction will be measured indirectly by running a second reaction, known as a clock reaction, simultaneously along with the reaction of interest. The clock reaction must be inherently fast relative to the reaction of interest and it must consume at least one of the products of the reaction of interest. In this case the parallel reactions are the following.
\[ \ce{6I^{-}( aq ) + BrO3^{-}( aq ) + 6H^{+}( aq )->3I2( aq ) + Br^{-}( aq ) + 3H2O( l )}\quad\quad \text{slow} \label{4}\]
\[ \text{(clock reaction)}\quad\quad \ce{3I2(aq) + 6S2O3^{2-}(aq)-> 6I^{-}(aq) + 3S4O2^{-}(aq)}\quad\quad \text{fast} \label{5}\]
Note that since the clock reaction is relatively fast, the \(\ce{I2(aq)}\) will be consumed by the clock reaction as quickly as it can be produced by the reaction of interest thus holding the \(\ce{I2}\) concentration at a very low value close to zero. Only after the \(\ce{S2O3^{2-}} \) has been entirely consumed will the concentration of \(\ce{I2}\) start to increase. After the \(\ce{S2O3^{2-}} \) has been consumed the concentration of \(\ce{I2}\) will increase rapidly allowing the \(\ce{I2}\) to react with a starch indicator resulting in the solution turning to a deep blue color.
In this experiment you will measure the time required for the solution to turn blue. This is essentially the amount of time required for all of the \(\ce{S2O3^{2-}} \) to be reacted. Inspection of Table 1 shows that the number of moles of \(\ce{S2O3^{2-}} \) is the same for all reaction mixtures and is relatively small compared to the number of moles of each reactant. Furthermore a stoichiometric calculation can be used to determine the number of moles of \( \ce{BrO3^{-}}\) reacted at the point in time when all of the \(\ce{S2O3^{2-}} \) has been consumed. By dividing the number of moles of \( \ce{BrO3^{-}}\) reacted by the total volume of the reaction mixture we can obtain the change in the \( \ce{BrO3^{-}}\) concentration. We can obtain the reaction rate by dividing this change in concentration by the amount of time required for the blue color to appear (\(\Delta t\)).
Part B: Determination of the Activation Energy of the Reaction
The value of the rate constant, \(k\), measured in Part A is dependent upon the temperature at which the reaction occurs according to the Arrhenius Equation :
\[k =Ae^{-E_{a} /RT}\label{6}\]
where \(A\) is the frequency factor and is related to the number of properly aligned collisions that occur per second between reactant molecules; \(E_{a}\) is the activation energy of the reaction and is the minimum energy that must be present in a collision for a reaction to occur; and \(R\) is the universal gas constant with a value of \(8.3145 \times 10^{-3} kJ·mol^{–1}\).
Taking the natural logarithm of both sides of Equation \ref{6} gives:
\[\ln k= -\dfrac{E_{a}}{R} \left( \frac{1}{T} \right) + \ln A \label{7}\]
Note that this equation is that of a straight line of the form \(y= mx + b\) where:
\[y = \ln k\]
\[x= \dfrac{1}{T}\]
\[m =-\dfrac{E_{a}}{R}\]
\[b= \ln A\]
Thus, if the rate constant, \(k\), is measured at several temperature and \(\ln k\) is plotted as a function of \(1/T\), the slope of the resulting line will allow the value of \(E_{a}\) for the reaction to be determined.
Part C: The Effect of a Catalyst upon Reaction Rate
Catalysts increase the rate of a chemical reaction by providing an alternate pathway or mechanism through which a reaction can proceed. Since the alternate mechanism has a lower activation energy than that of the uncatalyzed reaction the effect of the catalyst is to increase the rate of the reaction. To demonstrate the effect of a catalyst on the reaction rate you will repeat one of the trials from Part A in the presence of an ammonium molybdate, \( \ce{(NH4)2MoO4}\) ( aq ), catalyst .
Materials and Equipment
You will need the following additional items for this experiment: stopwatch (or digital timer), hot-water baths set at different temperatures (available in lab room) ,ice-water bath (obtain a bucket of ice from the stockroom), four 10-mL graduated cylinders (these must be shared with other groups; the stockroom does not have extra 10-mL cylinders to lend)
General Safety
Students must wear safety goggles at all times. The hot-water baths used in Part B of this experiment can become hot enough to burn your skin. Use caution when working with them.
PERSONAL PROTECTIVE EQUIPMENT (PPE)
- Lab coat, goggles, closed shoes and gloves required.
- Gloves needed when handling hydrochloric acid, iodine, and ammonium molybdate
CHEMICAL HANDLING: The ammonium molybdate catalyst used in Part C is known to be toxic and harmful to the environment. Care should be taken in handling this chemical and proper disposal is required.
WASTE DISPOSAL: Except for solutions containing the ammonium molybdate catalyst used in Part C, all other solutions used in this lab may be disposed of in the sink. All waste solutions from Part C containing the ammonium molybdate catalyst must be disposed of in an appropriate chemical waste container.
Experimental Set-up and Procedure: Preparation of Glassware
Because soap residue and other chemicals can interfere with the reaction we are observing it is critical that all glassware used in this experiment be rinsed several times using deionized water (and not soap!) prior to performing the experiment. Also, because the production of deionized water is very energy intensive glassware should be rinsed using a squirt bottle in order to minimize waste; never rinse glassware directly under the deionized water tap. In general there is no need to dry glassware after rinsing because most solutions that we use are aqueous.
In this part of the experiment, you will perform four trials. In each trial you will vary the initial concentration of one reagent in the reaction mixture. Table 1 below summarizes the volume of each reagent that should be used in each reaction mixture.
We see from Table 1 that two reaction flasks are used for each trial. This is because we shall measure the time that the clock reaction takes to turn blue from the moment we mix the contents of the two flasks. Observe that the total volume for each trial listed in Table 1 is the same. For example, 10.0 milliliters of water have been added to the mixture in Trial 1 so that its volume is the same as those for other trials. Also observe that the same quantity of sodium thiosulfate has been added to each reaction mixture. Why are these two conditions important? (There are two questions at the end of the experiment concerning this; you may wish to answer them now).
0.010 M \(\ce{KI}\) | 0.0010 M \(\ce{Na2S2O3}\) | \(\ce{H2O}\) | 0.040 M \(\ce{KBrO3}\) | 0.10 M \(\ce{HCl}\) | Starch | |
1 | 10.0 | 10.0 | 10.0 | 10.0 | 10.0 | 3-4 drops |
2 | 20.0 | 10.0 | -- | 10.0 | 10.0 | 3-4 drops |
3 | 10.0 | 10.0 | -- | 20.0 | 10.0 | 3-4 drops |
4 | 10.0 | 10.0 | -- | 10.0 | 20.0 | 3-4 drops |
- Rinse four 250-mL beakers, four 10-mL graduated cylinders, a thermometer, a 125- mL Erlenmeyer flask, and a 250-mL Erlenmeyer flask using deionized water as described in the preparation of glassware section above. There is no need to dry these after rinsing, but shake them gently several times to remove almost all the water.
- Using the four 250-mL beakers, you will collect about 100 mL of each of the four reagents needed to prepare the mixtures listed in Table 1. Rinse each beaker with about 5 mL of the particular reagent solution you will store in it first, pouring this rinse into the sink, then fill the beaker with the 100 mL you will need. In this way the beakers do not need to be dried before use. Label each beaker appropriately.
- Using one of the clean rinsed 10-mL graduated cylinders, measure 10.0 mL of deionized water and transfer it into the clean 250-mL Erlynmeyer flask (Flask I).
- Now place one of the four clean rinsed 10-mL graduated cylinders by each of the 250-mL beakers containing the reagents. Rinse each 10-mL graduated cylinder with about 2-3 mL of the reagent in the beaker next to it, pouring this rinse into the sink. You should now use these cylinders for measuring only the reagent in the beaker they are paired with. Label each of these graduated cylinders appropriately.
- We have already begun to prepare the half of Reaction Mixture 1 listed in Table 1 for Flask I. Into the 250-mL Erlenmeyer flask containing the 10.0 mL of deionized water, add 10.0 mL of the 0.010 M \(\ce{KI}\) reagent solution and 10.0 mL of the 0.0010 M \(\ce{Na2S2O3}\) reagent solution from your beakers using the appropriate 10-mL graduated cylinders.
- Now prepare the half of Reaction Mixture 1 listed in Table 1 for Flask II in a similar manner by combining 10.0 mL of the 0.040 M \(\ce{KBrO3}\) reagent solution, 10.0 mL of the 0.10 M \(\ce{HCl}\) solution, and 3 to 4 drops of starch indicator in the clean rinsed 125- mL Erlenmeyer flask.
- The next step requires two experimenters: one to operate the stopwatch or timer and another to mix and swirl the contents of the two flasks. Be certain that the person using the stopwatch or timer knows how to operate it by testing it once or twice before proceeding.
- Pour the contents of Flask II into Flask I rapidly and then swirl the solution to mix thoroughly. Start your stopwatch or timer the moment the two solutions are combined. Set the flask containing the solution down on a sheet of white paper and watch carefully for the blue color of the starch-iodine complex to appear. It should take about one to three minutes. Stop the timer the instant that the blue color appears. Record the elapsed time on your data sheet.
- Using your thermometer, measure the temperature of the reaction mixture immediately following the reaction to the nearest tenth of a degree and record this value on your data sheet.
- Dispose of the contents of the flask in the sink. Rinse both Erlenmeyer flasks and your thermometer as described in the preparation of glassware section.
- Repeat this procedure once more, performing a second trial for Mixture 1. Use the 10-mL graduated cylinder from the \(\ce{Na2S2O3}\) solution to measure the deionized water. Be sure to rinse this cylinder appropriately before using it again with the \(\ce{Na2S2O3}\) solution. If the time you measure for this second trial differs by more than ten percent from that of your first trial, repeat the procedure again. If after three trials you still unable to obtain two trials with reaction times that differ by less than ten percent, see your instructor.
- Now repeat this procedure for the other three mixtures listed in Table 1. You need only perform one trial for each of these mixtures. Compare the times you obtain for each of these mixtures with those obtained by other teams. Repeat any trials where the reaction time differs significantly from those obtained by other teams. Record these data on your data sheet. Do not forget to measure the temperature immediately following each trial.
- Prepare Flasks I and II as in Part A using the quantities given for Mixture 1 in Table 1.
- Prepare an ice-water bath by mixing ice and just enough water to fill the spaces between the pieces of ice in the small ice bucket obtained from the stockroom. Insert your thermometer into the 250-mL Erlenmeyer flask. (Do not move the thermometer to the other flask or you may inadvertently mix some of the reagents). Place both flasks carefully into the ice-water bath and wait for the temperature of the contents of the 250-mL flask to reach about 3°C.
- Remove the flasks from the ice-water bath and pour the contents of Flask II into Flask I rapidly. Swirl the solution to mix thoroughly. Start your timer the moment you combine the two solutions. Keep the flask containing the mixed solution in the ice bath and watch carefully for the blue color to appear. This should occur in about three to eight minutes. For the cold solution a faint blue color may appear initially and then grow darker. Stop the timer the instant that this faint blue color first appears. Record the elapsed time and the final temperature of this mixture on your data sheet.
- Perform two more trials for Part B at elevated temperatures using the hot-water bath at the side of the laboratory room in place of the ice-water bath. Each trial should be performed using the quantities given for Mixture 1 in Table 1. Heat both flasks in the hot-water bath until the temperature in the 250-mL flask reaches approximately 30°C (±5°C) in the first elevated-temperature trial, and about 40°C (±5°C) in the second elevated-temperature trial. You may need to hold or clamp the flasks in place to keep their contents from spilling while they are heating in the hot-water bath. When the appropriate temperature has been reached, remove the flasks, mix the contents, and record the elapsed time for the blue color to appear. Record the final temperature of the reaction mixture as before.
- Dispose of the contents of the flasks in the sink. Rinse the flasks and thermometer as before.
Part C: The Effect of a Catalyst Upon Reaction Rate
- Prepare Flasks I and II as in Part A above using the quantities given for reaction Mixture 1 in Table 1, but this time in addition to the starch indicator add one drop of 0.5 M ammonium molybdate, \(\ce{(NH4)2MoO4}\), to the reagents in Flask II.
- Pour the contents of Flask II into Flask I rapidly and then swirl the solution to mix thoroughly. Start your timer the moment you combine the two solutions. Set the flask containing the solution down on a sheet of white paper and watch for the blue color of the starch-iodine complex to appear. This should occur very rapidly in this case. Record the elapsed time and the final temperature of this mixture on your data sheet.
- Pour the contents of this flask into the appropriate chemical waste container. The contents of the flask containing the catalyst should NOT be poured down the sink. Rinse the flask you added the catalyst to well.
Lab Report: Chemical Kinetics- The Method of Initial Rates
Name: ____________________________ Lab Partner: ________________________
Date: ________________________ Lab Section: __________________
Part A – Finding the Rate Law Using the Method of Initial Rates
Data for Reaction Mixture 1 (to confirm constancy between trials):
1 | |||
2 | |||
3 (if required) | |||
Data for Mixtures 1 through 4:
1 (average from above) | |||
2 | |||
3 | |||
4 |
Initial Concentrations of Reagents after Mixing: (Use \(M_{1}V_{1} = M_{2}V_{2}\) to calculate values)
1 | ||||
2 | ||||
3 | ||||
4 |
- Show a sample calculation below illustrating how you arrived at the value entered in the first cell:
- Based on the stoichiometry of Equations \ref{1} and \ref{4}:
_______ mole(s) of \(\ce{S2O3^{2–}}\) are consumed for every _______ mole(s) of \(\ce{BrO3^{–}}\) that react.
- Therefore, the values of \(a\) and \(b\) in the relative rate expression:
\(-\frac{1}{a} \frac{\Delta [\ce{BrO3^{-}}]}{\Delta t} = -\frac{1}{b} \frac{\Delta [\ce{S2O3^{2-}}]}{\Delta t} \) are \(a\) = ______ and \(b\) = ______
Use your concentration and time data from the previous page to calculate the rate that \(\ce{S2O3^{2–}}\) is consumed in each of your four mixtures. Then use the values of \(a\) and \(b\) in the relative rate expression above to determine the corresponding initial rate of reaction in terms of the change in concentration of \(\ce{BrO3^{–}}\) reacted.
Relative Rates of Reaction:
) | (M⋅s ) | |
---|---|---|
1 | ||
2 | ||
3 | ||
4 |
- Show sample calculations below illustrating how you arrived at the values for Reaction Mixture 1:
Summary of Results for Use in the Method of Initial Rates: (copy the appropriate values from the tables on the previous two pages)
(M⋅s ) | ||||
---|---|---|---|---|
1 | ||||
2 | ||||
3 | ||||
4 |
- Use the method of initial rates and the relevant data in the table above to determine the order of each reactant as given by Equation \ref{2} and state the experimentally determined rate law below. Clearly show all your calculations, including which mixtures the data you used came from.
- The reaction order with respect to \(\ce{I^{-}}\): \(x\) = ______________
- The reaction order with respect to \(\ce{BrO3^{–}}\): \(y\) =______________
- The reaction order with respect to \(\ce{H^{+}}\): \(z\) =______________
- The Rate Law:
Using your experimentally determined rate law from the previous page determine the value of the rate constant, \(k\), for each of the four reaction mixtures and the average value of \(k\).
Value and Units of the Rate Constant, \(k\) :
Value of \(k\) |
- Show sample calculations below illustrating how you determined the value of \(k\) for Mixture 1:
- Average value of \(k\): ______________
- Units of \(k\):______________
Part B – The Effect of Temperature on the Reaction Rate
In this part we will use the temperature data collected in Parts A and B for Mixture 1 to determine the value of the activation energy, \(E_{a}\), for the reaction.
Complete the following table using your experimental data and calculated values from Parts A and B of the experiment. Attach your additional calculations on separate sheets of paper to the back of your laboratory report. Be sure to determine the value of the rate constant, \(k\), for each of the different temperatures below and give the appropriate units (the average value of \(k\) that you determined in Part A is valid only for the room temperature entry below).
Summary of Results for Use in Determining the Activation Energy of the Reaction:
Temp of Mixture, \(T\) (°C) | ||||
Temp of Mixture, \(T\) (K) | ||||
Elapsed Time (s) | ||||
\(-\frac{\Delta [\ce{S2O3^{2-}}]}{\Delta t}\) (M⋅s ) | ||||
\(-\frac{\Delta [\ce{BrO3^{-}}]}{\Delta t}\) (M⋅s ) | ||||
Rate Constant & Units, \(k\) | ||||
\(\ln k\) (no units) | ||||
\(1/T\) (K ) |
*For Room Temp use your data for Mixture 1 from Part A.
- Use Excel to create a graph of “\(\text{ln} k\) versus \(1/T\)”. Your graph should have an appropriate title and labeled axes with an appropriate scale. Using the Excel trendline function, add a best-fit line to your plotted data and have Excel display the equation of this line and its R 2 value on your graph. Submit this graph with your report.
- Use this graph to determine the value of the activation energy, \(E_{a}\), and the frequency factor, \(A\), for this reaction (be certain to include the proper units for each!). Show your calculations.
Calculations:
Value of \(E_{a}\):_________________ Units of \(E_{a}\):_________________
Value of \(A\):_________________ Units of \(A\):_________________

Part C – The Effect of a Catalyst Upon Reaction Rate
Reaction Time of Normal and Catalyzed Reaction for Mixture 1:
without catalyst* | |||
with catalyst |
*Use the data from Part A for Mixture 1.
- The volume of each the reagents listed in Table 1 are varied one at a time in turn except for the volume of sodium thiosulfate, which is the same in each trial. Why do we keep the volume of sodium thiosulfate the same in each trial?
- In Part A, even though the concentrations of the reactants are changed in each trial, the experimentally determined values of the rate constant, \(k\), for each trial should be fairly similar. Why is this?
- How do your results from Part B also support your answer to the previous question?
- What is the qualitative relationship between reaction rate and temperature obtained from your results in Part B? Can you give a physical explanation to explain this? What is occurring on the molecular level?
- Based on your results for Part C, how does the presence of the catalyst effect the value of the activation energy of this reaction?
- What additional experiment could you perform to determine the value of the activation energy for the catalyzed reaction?
- What is one difficulty would you might face in performing the experiment that you described in your answer to the previous question? How might you attempt to overcome this difficulty?
Pre-laboratory Assignment: Chemical Kinetics- The Method of Initial Rates
- Suppose a student prepares reaction mixture 2 (see Table 1 in Part A). When the contents of the reaction flasks 1 and 2 are mixed together it takes 75 seconds for the blue color to appear.
- Calculate the initial concentrations of the reactants after mixing. For example, the concentration of \(\ce{S2O3^{2-}}\) is calculated as follows.
\[ [\ce{S2O3^{2-}}] = \frac{(0.010 L) (0.0010 \frac{mol}{L})}{(0.050 L)} = 2.0 \times 10^{-4} M \]
\([\ce{I^{-}}]\) =
\([\ce{BrO3^{-}}]\) =
\([\ce{H^{+}}]\) =
- Calculate the initial rate of the reaction (\(-\frac{\Delta [\ce{BrO3^{-}}]}{\Delta t} \)) in units of \(\frac{mol \ce{BrO3^{-}}}{L\cdot s}\).
- Suppose that the amount of time needed for reaction mixture 1 to turn blue is 145 seconds. Calculate the initial concentrations of the reactants after mixing for reaction mixture 1 and use the method of initial rates to determine the reaction order, \(x\), with respect to \([\ce{I^{-}}]\).
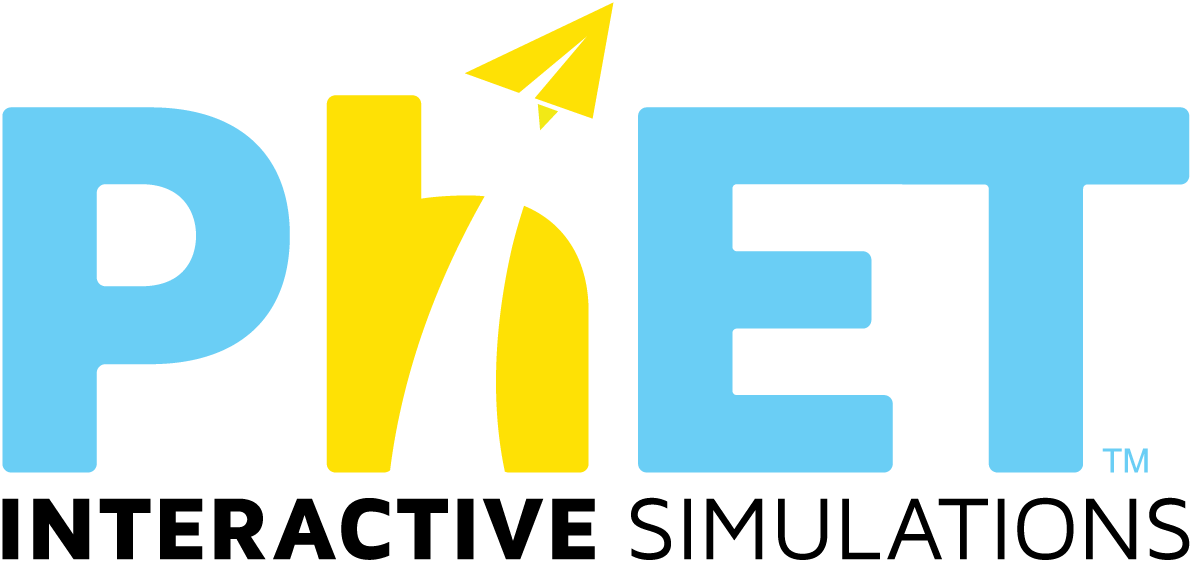
- Sign in / Register
- Administration
- Edit profile
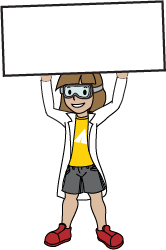
The PhET website does not support your browser. We recommend using the latest version of Chrome, Firefox, Safari, or Edge.

Lab Procedure: Iodine Clock Reaction

Core Concepts
In this lab tutorial, we learn about the iodine clock reaction, including its procedure, underlying chemistry, and data analysis.
Topics Covered in Other Articles
- Lab Safety Rules
- Recrystallization
- Thin Layer Chromatography
- Distillation
- Integrated Rate Laws
What is the Iodine Clock Reaction?
The Iodine Clock Reaction is a classic chemistry experiment that demonstrates many basic principles of kinetics and redox chemistry . For this, the reaction persists as a staple of general chemistry lab demonstrations.
In this experiment, you prepare two simple, transparent solutions. Once the solutions combine, however, the mixture gradually turns from clear to dark blue to near-black. This color change corresponds to the progress of the reaction, which allows you to visually witness the kinetics in a way that most reactions do not provide.
Interestingly, some chemists colloquially call this reaction the “Egyptian Night” experiment. In Egypt, the darkness of nighttime often arrives rather suddenly, similar to rapid dark color change in this reaction.

Iodine Clock Procedure
To perform the Iodine Clock Reaction, you need an iodine salt, a reductant, an oxidant, an acid , starch, and water as a solvent. As mentioned before, these components become allocated between three different solutions according to these specifications:
- First Solution: Starch, Water.
- Second Solution: Iodine Salt, Reductant, Water.
- Third Solution: Oxidant, Acid, Water.
Once the solutions mix, the reaction begins.
The most common variant of the Iodine Clock Reaction uses sodium thiosulfate (Na 2 S 2 O 3 ) as the reductant and hydrogen peroxide (H 2 O 2 ) as the oxidant. Potassium iodide (KI) serves as the salt, while sulfuric acid (H 2 SO 4 ) provides the required acidity. Importantly, gloves, safety goggles, and caution should be observed when using sulfuric acid and hydrogen peroxide to prevent chemical burns.
As we’ll find out in a later section, the kinetics of the reaction depends on the concentrations of acid, iodide, and oxidant. Thus, most lab procedures studying reaction kinetics will vary the concentrations of one or more of these species. Aside from that, the reductant concentration tends to be kept low, as very little is required, while the starch tends to be in excess.
The Chemistry of the Iodine Clock
Iodine clock redox and kinetics.
Before the three solutions mix into one, each ionic species dissociates into their respective ions:
KI → K + + I –
Na 2 S 2 O 3 → 2Na + + S 2 O 3 2-
H 2 SO 4 → H + + HSO 4 –
During the reaction, K + , Na + , and HSO 4 – do not participate, remaining as spectator ions. Once the solutions mix, the hydrogen peroxide oxidizes the iodide into diatomic iodine:
2H + + H 2 O 2 + 2I – → I 2 + 2H 2 O
Importantly, as the reaction produces diatomic iodine, the thiosulfate re-reduces the iodine back to iodide:
2S 2 O 3 2- + I 2 → 2I – + S 4 O 6 2-
This back and forth between iodide and iodine continues until all thiosulfate oxidizes away. Afterward, significant quantities of iodide and iodine exist at the same time. They react with one another to form the triiodide ion:
I 2 + I – → I 3 –
This triiodide ion then forms a complex with the starch. This complex is responsible for the increasing dark blue of the reaction vessel. As a side note, due to the striking dark blue of the complex, a mixture of iodine and iodide called Lugol’s iodine is used to test for trace amounts of starch .

Iodine Clock Kinetics
The first reaction, the oxidation, occurs much slower than the reduction, making it the rate-determining step during that first phase of the reaction. Additionally, once the reduction ceases, the oxidation continues to serve as the rate-determining step, as both the formation of the triiodide and the starch complex occur relatively quickly. Thus, for the entirety of the experiment, oxidation determines the progress of the dark blue hue. This is true even though the starch complex is not directly generated from the oxidation.
Aside from the qualitative observation of the increasingly blue reaction vessel, you can periodically measure the starch concentration through spectrophotometry . The resulting data then allows you to quantify the reaction kinetics.
Kinetic Data Analysis
First, you need to do multiple trials of the Iodine Clock with different concentrations of potassium iodide. Then, you quickly place these samples into a spectrophotometer that records concentrations at consistent time intervals. You’d want to set the spectrophotometer to a frequency similar to 600nm to pick up the dark blue of the starch complex.

Next, you graph your data. You should find that the absorbance of each graph increases linearly with time. This makes sense since chemical reactions always initially proceed at linear rates.

Finally, to determine the reaction order with respect to KI, you take the logarithms of the initial concentrations and reaction rates and generate a log/log graph.

The slope of the resulting slope corresponds to the reaction order in our rate law , due to the properties of logarithms.
RxnRate = k’[KI] n
log(RxnRate) = log(k’[KI] n ) = nlog([KI]) + log(k’)
k’: Relative rate constant (s -1 )
n: Reaction Order of KI
The graph then generates a trendline of y = x – 1.2883, indicating that the Iodine Clock Reaction is first order with respect to KI (n = 1).
- Berkeley Lab
- Energy Technologies Area
- Energy Storage & Distributed Resources
- Energy Conversion Group
A Better Model for Converting Carbon Dioxide into Fuels and Products
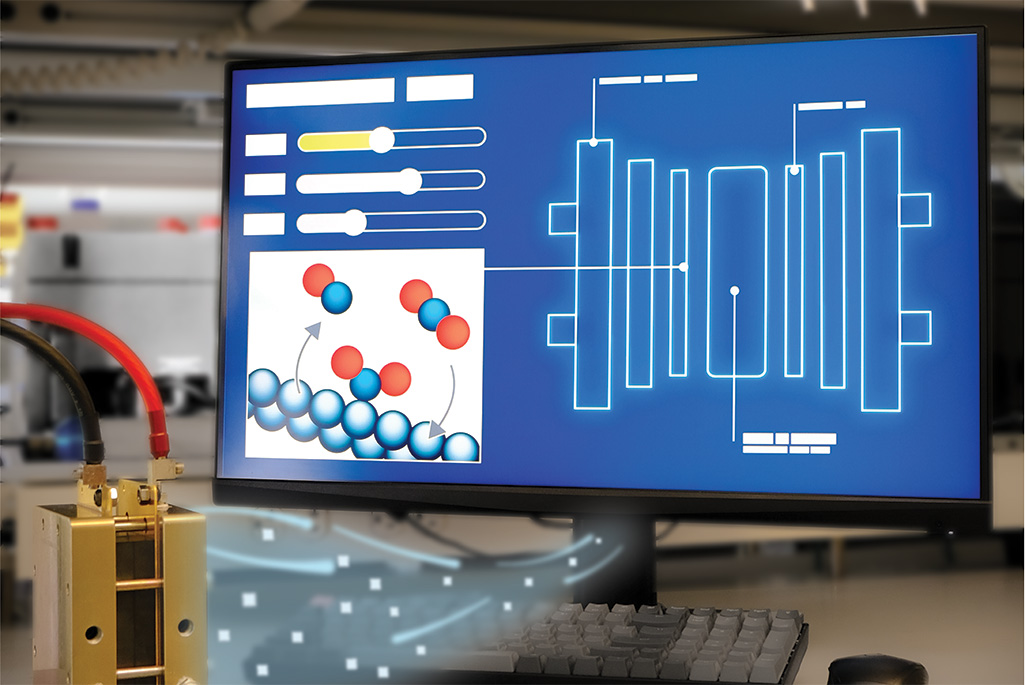
Some parts of the world have been so successful in making inexpensive renewable electricity that we occasionally have too much of it. One possible use for that low-cost energy: Converting carbon dioxide into fuel and other products using a device called a membrane-electrode assembly.
A team of scientists from Lawrence Berkeley National Laboratory (Berkeley Lab) and the University of California Berkeley have developed a new approach to understanding this promising technology via physics modeling. The paper, which was recently published in the journal Nature Chemical Engineering , could help scientists learn how to improve membrane-electrode assembly efficiency.
Carbon dioxide can be transformed into valuable feedstocks such as carbon monoxide and ethylene, which manufacturers use to make products including chemicals and packaging. One way to do this is with membrane-electrode assemblies, which are devices that consist of two electrodes separated by a membrane. Also used in fuel cells that turn inputs like hydrogen into electricity, membrane-electrode assemblies hold promise for being able to use surplus renewable power to run reaction sequences that catalyze carbon dioxide into other chemicals. But these devices have problems with efficiency, and their workings are not yet fully understood.
“Membrane-electrode assemblies are complicated systems with multiple layers. Each layer holds different chemical species, additives, and particles,” said Adam Weber, a senior scientist at Berkeley Lab and corresponding author of the study. “Often, we don’t really know why experiments with membrane-electrode assemblies produce certain products, or why they fail to convert a larger percentage of a given amount of carbon dioxide.”
Computer modeling can help predict which device parameters will produce the best results, but they tend to be less accurate at anticipating issues such as crossover, which is when carbon dioxide moves across the membrane instead of reacting. To improve model accuracy, the researchers turned to Marcus–Hush–Chidsey kinetics, a theory that previously had not been integrated into membrane-electrode assembly modeling and is shown to be critical for understanding the reaction mechanism.
The researchers validated their model against experimental data, finding that it did a better job of predicting real-world outcomes than previous models. Among other advantages, the use of Marcus–Hush–Chidsey kinetics made it possible to account for the role of water orientation.
The team then ran virtual experiments with its model to explore how different membrane-electrode assembly designs performed in terms of carbon-dioxide utilization and selectivity for desired products. “With this work, we have shown how you can leverage chemical engineering principles toward these advanced technologies that are coming online,” he said. “That gives us insights and ideas for optimizing these cell designs and materials so we can go forward and make them.”
Some of the variables the team tested virtually included catalyst-layer thickness and catalyst-specific surface area. They also uncovered design rules around the importance of coupled ion and water transport, as well as tradeoffs between transport phenomena and reaction and buffer kinetics. All of these change the overall energy efficiency, products obtained, and amount of carbon dioxide converted.
“Having a digital twin of a system allows you to probe a much larger parameter space much more rapidly than in experiments, which are typically complex and require special equipment,” Weber said, adding, “We can’t see where every molecule is in an experiment. But in a model, we can.”
Weber said the next step in the research is to increase the model’s complexity to be able to look at performance over a membrane-electrode assembly’s lifetime, among other variables.
This research was supported in part by the Department of Energy’s Bioenergy Technologies Office and Berkeley Lab’s Laboratory Directed Research and Development Grant.
This article originally appeared at the Berkeley Lab News Center .

IMAGES
VIDEO
COMMENTS
This page titled 1: Chemical Kinetics - The Method of Initial Rates (Experiment) is shared under a CC BY-NC license and was authored, remixed, and/or curated by Santa Monica College. The objectives of this experiment are to determine the rate law of a chemical reaction using the Method of Initial Rates, to determine the activation energy of the ...
laws via this method in the chapter on chemical kinetics in your textbook before proceeding with this experiment. The reaction to be studied in this experiment is represented by the following balanced chemical equation: 6 € I− (aq)+BrO 3 − (aq)+6 H+(aq)→3 I 2 Br − 3 2Ol (1) This reaction proceeds relatively slowly.
We recommend using the latest version of Chrome, Firefox, Safari, or Edge. Explore what makes a reaction happen by colliding atoms and molecules. Design experiments with different reactions, concentrations, and temperatures. When are reactions reversible? What affects the rate of a reaction?
Chemistry M01B Laboratory Manual Page 15 In this experiment, we will study the kinetics of the reaction between iodine and acetone in aqueous solution: H 3 C C C H 3 O + I 2 H 3 C C C H 2 I O +H+ I-H+ The rate of this reaction is expected to depend on the concentration of hydrogen ion in the solution, a catalyst, as well as the concentrations ...
The Iodine Clock Reaction is a classic chemistry experiment that demonstrates many basic principles of kinetics and redox chemistry. For this, the reaction persists as a staple of general chemistry lab demonstrations. In this experiment, you prepare two simple, transparent solutions.
Experiment 3 Chemical Kinetics - Determining the Rate Equation Introduction: In order to be in a position to manipulate any operation, one has to know all the fine details of the system ... This lab is very dependent on dispensing the exact quantities. 3. Into a 15cm test tube place the required amount of KMnO4. Use the same precaution as above.
Chemistry M01B Laboratory Manual Page 24 Experiment #5 - Chemical Kinetics: Iodine Clock Reaction In the previous experiment, we discussed the factors that influence the rate of a chemical reaction and presented the terminology used in quantitative relations in studies of the kinetics of chemical reactions.
Chemical kinetics deals with how fast chemical reactions happen and with how these rates are dependent upon factors such as concentration, temperature, or the presence of a catalyst. In this experiment we will examine the effect of the change of initial concentrations on the reaction rate of a colored dye, crystal violet, and hydroxide ion: [(H ...
5.310 Laboratory Chemistry . EXPERIMENT # 5. 1 . A Study of the Kinetics of the Enzyme Catalase and its Reaction . With H. 2 O 2. 2 ... One of the main goals of chemical kinetics is to understand the steps by which a reaction takes place and how reaction conditions such as temperature and concentration
The kinetics of the reaction between iodide (I−) and peroxydisulfate (S2O82-) will be explored in this experiment. The reaction is also referred to as the "Iodine Clock Reaction". The chemical reaction between these two substances is shown in Equation 8 below. 3I−(aq) + S2O82-(aq) ⇔ I3−(aq) + 2SO42-(aq) Equation 8.
Lab 11 - Chemical Kinetics ... The experiment will be set up such that the amount of thiosulfate (S 2 O 3 2-) is only about one percent of the amounts of iodide and persulfate (S 2 O 8 2-) ions. During the first percent or so of Reaction 6, the elemental iodine produced is consumed by thiosulfate in Reaction 7. No iodine is left to react with ...
CHEM 126 LAB. February 10th 2021. Experiment 4: Kinetics Introduction: The rates of chemical reaction and the ability to control these reactions are important aspects in life. Chemical kinetics is the study of the rates at which chemical reactions occur, the factors affecting the rate of reactions and the mechanisms [order] in which they occur.
Experiment 3: Chemical Kinetics Report Sheet/Post Lab Questions. Measure Solution 1 Solution 2 Solution 3 ↑ Temperature Volume H 2 O 2 (mL) Volume H 2 O (mL) Volume KI (mL) Slope. Show your calculation for the slope. m= n= Write the Rate Law for this reaction. What is the order of this reaction? Did the rate for the higher temperature ...
Chemical kinetics determine the rate of the reaction and the order of each reactant that can be only done by experiment. In this experiment, blue solution and NaOCL solution are used to investigate the rate of reaction or to see how long it takes to reach the 100 percent of transmittance by using Spectronic 20.
Fill a cuvette about 3/4 full with distilled water, or the solvent being used in the experiment, to serve as the blank. After the spectrophotometer has warmed up, place the blank cuvette in the spectrophotometer. Align the cuvette so a clear side of the cuvette is facing the light source.
Chemical Kinetics: Determining Rate Laws for Chemical Reactions v010615 INTRODUCTION It is thought that the birth of chemical kinetics occurred in 1850 when a German chemist, Ludwig ... In this experiment we will examine the effect of the change of initial concentrations on the reaction rate of the food dye, FD&C Blue #1 (792.85 g/mol), and ...
Among other advantages, the use of Marcus-Hush-Chidsey kinetics made it possible to account for the role of water orientation. The team then ran virtual experiments with its model to explore how different membrane-electrode assembly designs performed in terms of carbon-dioxide utilization and selectivity for desired products.
Experiment 7: Chemical Kinetics. Procedure. See my pre-lab for a general overview of the experimental procedure. The laboratory. experiment I conducted follows the same procedures stated in my pre-lab, no changes. were made to my experiment. In my experiment the solutions I used include: 0 M. Ascorbic acid, 0 M Hydrochloric acid, and2. 09 × 10 ...