
An official website of the United States government
The .gov means it's official. Federal government websites often end in .gov or .mil. Before sharing sensitive information, make sure you're on a federal government site.
The site is secure. The https:// ensures that you are connecting to the official website and that any information you provide is encrypted and transmitted securely.
- Publications
- Account settings
- Browse Titles
NCBI Bookshelf. A service of the National Library of Medicine, National Institutes of Health.
Institute of Medicine (US) Forum on Drug Discovery, Development, and Translation. Breakthrough Business Models: Drug Development for Rare and Neglected Diseases and Individualized Therapies: Workshop Summary. Washington (DC): National Academies Press (US); 2009.
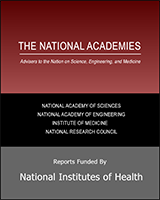

Breakthrough Business Models: Drug Development for Rare and Neglected Diseases and Individualized Therapies: Workshop Summary.
- Hardcopy Version at National Academies Press
2 Current Model for Financing Drug Development: From Concept Through Approval 1
The cost of developing a new drug has been estimated to be more than $1 billion. Development of this scale involves multiple financing mechanisms, as well as the involvement of numerous partners throughout the process. As background for the workshop discussions, Dr. Caskey provided an overview of the current financial landscape at various stages of drug development, including the investors at each stage and the current state of investments, and put forth several suggestions for ways to facilitate drug development.
- INVESTORS IN DRUG DEVELOPMENT
The principal investors in drug development differ at each stage. While basic discovery research is funded primarily by government and by philanthropic organizations, late-stage development is funded mainly by pharmaceutical companies or venture capitalists. The period between discovery and proof of concept, however, is considered extremely risky and therefore has been difficult to fund. Several initiatives discussed below have been undertaken to overcome this funding gap.
Early-and Late-Stage Development
Historically, the largest government investments in basic drug discovery research have been made by the National Institutes of Health (NIH). The Defense Advanced Research Projects Agency (DARPA) has also contributed to the discovery stage by taking on some relatively high-risk biologic projects. Moreover, in part as a result of the public’s impatience with the slow pace of the discovery process, state governments are increasingly taking the initiative in this area. One such example is the California Institute for Regenerative Medicine, a state agency established in 2005 by the California Stem Cell Research and Cures Initiative, which provides grants and loans for stem cell research and facilities at California’s research institutions and universities. Another example is the Texas Cancer Initiative, under which state funds are dedicated to cancer research conducted in Texas. Beyond these public investments, private foundations are also taking a significant financial interest in the discovery process, facilitating progress by funding research in their particular areas of interest.
At the other end of the continuum is late-stage development, which is funded primarily by pharmaceutical companies or venture capitalists with some collaborative support from government sources, such as NIH. Such partnerships are critical in the transition from proof of concept to clinical development.
Translational Research: Discovery Through Proof of Concept
Between basic discovery research and late-stage development lies the critical step of proving the utility of a proposed drug. The funding gap that often occurs in this period has been referred to as the “valley of death.” The risks are great and may be considered as not worth taking for products designed to treat rare and neglected diseases, which may ultimately yield a very limited return on investment. To help fill this funding gap, U.S.-based foundations have increased their investments in discovery and development for new drugs specific to their diseases of interest. In 2007 such groups invested approximately $75 million in biopharmaceutical companies, a 10-fold increase since 2000 ( Gambrill, 2007 ).
At the government level, the Department of Health and Human Services’ Small Business Innovation Research and Small Business Technology Transfer programs provide financial assistance to small companies attempting to advance their initial discoveries to commercial development. In recent years, NIH has significantly increased its focus on translational research. For example, NIH’s National Center for Research Resources administers the Clinical and Translational Science Awards, which fund a national consortium of medical research centers that train physicians in the drug development process. Likewise, the Food and Drug Administration (FDA) encourages the use of Cooperative Research and Development Agreements (CRADAs) to foster public–private partnerships in targeted areas of interest to the agency. Initiatives are taking place at the state level as well. The Texas Emerging Technology Fund, for example, is designed specifically to help companies finance proof-of-concept research. The fund invests in biologic sciences and biotechnology, as well as engineering, materials science, and information technology, and has committed funding to individual companies ranging from $500,000 to $10 million. The aforementioned Texas Cancer Initiative and California Stem Cell Research and Cures Initiative support research through this phase as well. In addition to government programs and philanthropy-and state-funded initiatives, many start-up companies, launched to develop discoveries emerging from academic laboratories, are being supported initially by venture capitalists and “angel investors.”
- CURRENT STATUS OF INVESTMENTS
Inhibitors of Development
Caskey argued that, despite the desire for development of new therapies, several environmental factors negatively affect new investments in drug development. Examples include decreased funding for basic research, regulatory barriers, and problems with drug safety that lead to product withdrawals. Elaborating on these points, Caskey emphasized first that the investment in basic research has been essentially flat in recent years. NIH funding has not increased significantly since its budget doubled from $13 billion in 1998 to $26 billion in 2003, and given inflation, its investment power has actually diminished over the last 5 years, in effect reducing the dollars available for funding new research. Fewer investments in basic research can result in fewer new drug therapy candidates, which in turn can result in fewer investments by private industry to advance promising candidates.
Second, navigating novel products or technologies through the existing regulatory pathways is challenging as scientific advances are made and regulations continue to evolve. In light of the increasing uncertainty of the regulatory process and possible increases in regulatory requirements throughout the development process, investors may shy away from investing in a product before there is clear evidence of its safety and effectiveness. An example is FDA’s initiative to address biomarker evaluation. Although FDA currently allows the use of biomarkers as surrogate end points in some cases, evaluation of biomarkers is difficult, and the agency is working to determine the best approach to the regulatory assessment of biomarker data.
Finally, Caskey argued that lawsuits following product withdrawals greatly affect new investments in development. For example, individual and class-action lawsuits following the withdrawal of Fen-Phen led to settlements of $20–30 billion ( Caskey, 2007 ). This amount of money could potentially have funded the development of 30 to 40 new drugs.
Opportunities to Improve the Financial Landscape
When a program fails in Phase III clinical trials because of either a lack of efficacy or problems with safety, a great amount of money and time that have already been spent go to waste. Thorough identification and validation of drug targets is a critical step in early discovery research that can drastically reduce late-stage drug failures. However, even though a target may be validated and a drug may appear to have an acceptable safety profile, one can never know all of the safety issues that may arise when a new therapeutic is introduced into broad use in the market.
In addition to funding for the discovery and development of drug candidates, funding is needed for research on new technology platforms for validating targets and therapeutics. Recently, venture capitalists have taken an interest in companies developing new platforms. These new technologies can play a significant role in facilitating drug discovery and enhancing drug safety. 2 High-throughput screening platforms that evaluate DNA, RNA, or proteins have already advanced the art of drug discovery. One example is mRNA expression profiling, a powerful micro-array technology platform that was discovered by an academic laboratory, received additional research funding from NIH, and was then commercialized by industry. Profiling of mRNA expression can indicate the phenotype of cells and be used to characterize cancers, but has also been employed successfully in the drug discovery process to identify and validate new targets and measure the responsiveness of a target to a drug. Another platform example is biochemical pathway analysis using mass spectrometry to measure analytes, rather than nucleic acid methods or proteomics. Such a high-throughput method for assessing numerous markers and pathways associated with a disease or drug action can contribute to efficacy and safety analysis prior to clinical use of a drug.
- WAYS TO FACILITATE DRUG DEVELOPMENT
Caskey put forth a number of suggestions for overcoming the impediments to new drug discovery and development:
The academic research community needs to increase investments in technology that can improve target validation and drug safety.
Government research funding aimed at addressing health challenges needs to be more focused on forecast morbidity and the cost of care in the United States.
FDA needs to be adequately funded so it can partner with drug developers and direct the research being performed toward answering important regulatory questions.
Small Business Innovation Research and Small Business Technology Transfer regulations need to be revisited and revised to allow for greater investment.
New incentives for high-risk investors need to be created, perhaps through tax law.
Private disease foundations’ provision of support to the academic community for discovery and to industry for development is beneficial and should be embraced.
Experienced investors need to be brought into the innovation process earlier.
The pharmaceutical industry and academia need to work together to build a stronger U.S. industry.
This chapter is based on the presentation of C. Thomas Caskey, M.D., Director and Chief Executive Officer and Chief Operating Officer of the Brown Foundation Institute of Molecular Medicine for the Prevention of Human Diseases at the University of Texas Health Science Center.
For more detailed discussion of technologies that are improving the efficiency of drug development and the safety of new products, see Caskey, 2007 .
- Cite this Page Institute of Medicine (US) Forum on Drug Discovery, Development, and Translation. Breakthrough Business Models: Drug Development for Rare and Neglected Diseases and Individualized Therapies: Workshop Summary. Washington (DC): National Academies Press (US); 2009. 2, Current Model for Financing Drug Development: From Concept Through Approval.
- PDF version of this title (3.2M)
In this Page
Other titles in this collection.
- The National Academies Collection: Reports funded by National Institutes of Health
Recent Activity
- Current Model for Financing Drug Development: From Concept Through Approval - Br... Current Model for Financing Drug Development: From Concept Through Approval - Breakthrough Business Models
Your browsing activity is empty.
Activity recording is turned off.
Turn recording back on
Connect with NLM
National Library of Medicine 8600 Rockville Pike Bethesda, MD 20894
Web Policies FOIA HHS Vulnerability Disclosure
Help Accessibility Careers
An official website of the United States government
Official websites use .gov A .gov website belongs to an official government organization in the United States.
Secure .gov websites use HTTPS A lock ( Lock Locked padlock icon ) or https:// means you've safely connected to the .gov website. Share sensitive information only on official, secure websites.
- Publications
- Account settings
- Advanced Search
- Journal List

Comparison of Research Spending on New Drug Approvals by the National Institutes of Health vs the Pharmaceutical Industry, 2010-2019
Ekaterina galkina cleary , phd, matthew j jackson , phd, edward w zhou , pharmd, fred d ledley , md.
- Author information
- Article notes
- Copyright and License information
Accepted for Publication: February 18, 2023.
Published: April 28, 2023. doi: 10.1001/jamahealthforum.2023.0511
Open Access: This is an open access article distributed under the terms of the CC-BY License . © 2023 Galkina Cleary E et al. JAMA Health Forum .
Corresponding Author: Fred D. Ledley, MD, Center for Integration of Science and Industry, Bentley University, Jennison Hall 144, 175 Forest St, Waltham, MA 02452 ( [email protected] ).
Author Contributions: Dr Ledley had full access to all of the data in the study and takes responsibility for the integrity of the data and the accuracy of the data analysis.
Concept and design: Ledley.
Acquisition, analysis, or interpretation of data: All authors.
Drafting of the manuscript: Ledley.
Critical revision of the manuscript for important intellectual content: All authors.
Statistical analysis: All authors.
Obtained funding: Ledley.
Administrative, technical, or material support: Jackson.
Supervision: Ledley.
Conflict of Interest Disclosures: Drs Jackson, Galkina Cleary, and Ledley reported grants from the National Biomedical Research Foundation and Institute for New Economic Thinking during the conduct of the study as well as grants from the West Health Policy Center and National Pharmaceutical Council outside the submitted work. Dr Zhou reported grants from the National Biomedical Research Foundation during the conduct of the study as well as a grant from the National Pharmaceutical Council outside the submitted work. No other disclosures were reported.
Funding/Support: This work was supported by grants from the Institute for New Economic Thinking and the National Biomedical Research Foundation.
Role of the Funder/Sponsor: The funding organizations had no role in the design and conduct of the study; collection, management, analysis, and interpretation of the data; preparation, review, or approval of the manuscript; and decision to submit the manuscript for publication.
Data Sharing Statement: See Supplement 2 .
Additional Contributions: We thank Juliana Harrison, MBA, Bentley University for assistance preparing the manuscript; Zoë Folchman-Wagner, PhD, and Vineeta Tanwar, PhD, Bentley University, for contributions to data collection; John Overington, PhD, VP Discovery Informatics, Exscientia and Olivier Wouters, PhD, London School of Economics, for consultation concerning their published works; and Michael Boss, PhD, Nancy Hsiung, PhD, and Bruce Leicher, JD, Bentley University, for their critical analysis. Drs Boss and Hsiung and Mr Leicher received no compensation for work at Bentley University or their role in this study.
Corresponding author.
Received 2022 Aug 5; Accepted 2023 Feb 18; Collection date 2023 Apr.
This is an open access article distributed under the terms of the CC-BY License.
How does National Institutes of Health (NIH) investment in pharmaceutical innovation compare with investment by the pharmaceutical industry?
In this cross-sectional study of 356 drugs approved by the US Food and Drug Administration from 2010 to 2019, the NIH spent $1.44 billion per approval on basic or applied research for products with novel targets or $599 million per approval considering applications of basic research to multiple products. Spending from the NIH was not less than industry spending, with full costs of these investments calculated with comparable accounting.
The results of this cross-sectional study suggest that the relative scale of NIH and industry investment in new drugs may provide a basis for calibrating the balance of social and private returns from these products.
Government and the pharmaceutical industry make substantive contributions to pharmaceutical innovation. This study compared the investments by the National Institutes of Health (NIH) and industry and estimated the cost basis for assessing the balance of social and private returns.
To compare NIH and industry investments in recent drug approvals.
Design, Setting, and Participants
This cross-sectional study of NIH funding associated with drugs approved by the FDA from 2010 to 2019 was conducted from May 2020 to July 2022 and accounted for basic and applied research, failed clinical candidates, and discount rates for government spending compared with analogous estimates of industry investment.
Main Outcomes and Measures
Costs from the NIH for research associated with drug approvals.
Funding from the NIH was contributed to 354 of 356 drugs (99.4%) approved from 2010 to 2019 totaling $187 billion, with a mean (SD) $1344.6 ($1433.1) million per target for basic research on drug targets and $51.8 ($96.8) million per drug for applied research on products. Including costs for failed clinical candidates, mean (SD) NIH costs were $1441.5 ($1372.0) million per approval or $1730.3 ($1657.6) million per approval, estimated with a 3% discount rate. The mean (SD) NIH spending was $2956.0 ($3106.3) million per approval with a 10.5% cost of capital, which estimates the cost savings to industry from NIH spending. Spending and approval by NIH for 81 first-to-target drugs was greater than reported industry spending on 63 drugs approved from 2010 to 2019 (difference, −$1998.4 million; 95% CI, −$3302.1 million to −$694.6 million; P = .003). Spending from the NIH was not less than industry spending considering clinical failures, a 3% discount rate for NIH spending, and a 10.5% cost of capital for the industry (difference, −$1435.3 million; 95% CI, −$3114.6 million to $244.0 million; P = .09) or when industry spending included prehuman research (difference, −$1394.8 million; 95% CI, −$3774.8 million to $985.2 million; P = .25). Accounting for spillovers of NIH-funded basic research on drug targets to multiple products, NIH costs were $711.3 million with a 3% discount rate, which was less than the range of reported industry costs with 10.5% cost of capital.
Conclusions and Relevance
The results of this cross-sectional study found that NIH investment in drugs approved from 2010 to 2019 was not less than investment by the pharmaceutical industry, with comparable accounting for basic and applied research, failed clinical trials, and cost of capital or discount rates. The relative scale of NIH and industry investment may provide a cost basis for calibrating the balance of social and private returns from investments in pharmaceutical innovation.
This cross-sectional study examines National Institutes of Health and pharmaceutical industry investments in recent drug approvals.
Introduction
Private sector investment and returns are classically viewed as the primary driving force for innovation. Evidence also shows that public sector investments in basic and applied biomedical research, including those from the National Institutes of Health (NIH), contribute substantively to the emergence of new drugs 1 , 2 , 3 , 4 , 5 , 6 , 7 and drug-related patents. 2 , 4 , 8 , 9 Recent economic studies have recognized the government’s contributions to pharmaceutical innovation by contextualizing government as an “early-stage investor and government funding for research as an investment.” 10 , 11 , 12 , 13 , 14 , 15 , 16 , 17 As such, these studies argued that there should be an equitable balance of investment risk and return between the public and private sectors 15 , 16 and framed policy regarding the pharmaceutical industry’s drug pricing practices and profits as shaping this balance. 17 , 18
The objective of this study was to compare NIH investment in the products approved by the US Food and Drug Administration (FDA) from 2010 to 2019 with reported levels of investment by the industry. 19 , 20 , 21 This comparison required an accounting for NIH spending comparable with that used to estimate total industry investment. This typically includes not only costs associated with approved products, but also costs associated with products that fail in clinical development and the cost of capital, or opportunity cost, associated with these investments. 19 , 20 , 22 , 23
Funding from the NIH for pharmaceutical innovation has been estimated from total NIH budget allocations 24 or categorical funding from the Research, Condition, and Disease Categories or Research Portfolio Online Reporting Tools (REPORTER). 25 , 26 These methods do not delineate spending associated with individual products. Case study methods have been used to identify NIH contributions associated with specific patents 2 , 8 or products. 5 These methods may not capture funding for basic research, which represents half of NIH funding and is classically undertaken “without specific applications towards processes or products in mind.” 27
Other studies have focused on NIH funding for published research associated with approved drugs or their targets. 6 , 28 , 29 In these studies, the costs of NIH-funded projects (grants) supporting research on a drug or its target were used as a measure of the NIH contribution to that product. In this method, drug-related publications represent applied research, and those associated with the drug’s target, but not the drug, represent basic research. Initial studies using this method identified NIH funding for research underlying each of the 210 drugs approved from 2010 to 2016, with total NIH costs of more than $100 billion and funding for each first-in-class drug of more than $800 million. 28 These studies also demonstrated spillover effects in which NIH spending for basic research in immunology or endocrinology contributed to the development of products for treating cancer. 29
This study extended these methods by developing an accounting for NIH spending that was comparable with reported investments by the industry. Using a data set of drugs approved from 2010 to 2019 (before the COVID-19 pandemic), this analysis estimated the NIH investment in these drugs, including the cost of published basic and applied research associated with these products, cost of phased clinical trials of failed product candidates, and opportunity cost, using discount rates recommended for government spending. 30 , 31 These estimates were used to compare NIH and industry investments in new drug approvals, the cost savings to the industry provided by NIH spending, and the economic efficiencies created through spillovers of NIH-funded basic research on drug targets to multiple products. These results are discussed in the context of policy regarding drug pricing and corporate profit that affects the balance of investment risk and reward between the public and private sectors.
Study Design
This cross-sectional study analyzed NIH-funding for published research related to drugs approved from 2010 to 2019 or their biological targets that was conducted from May 2020 to July 2022. This study did not involve human participants and was not subject to institutional review board review. The study was reported in accordance with the Strengthening the Reporting of Observational Studies in Epidemiology ( STROBE ) reporting guidelines.
Data Sources
The core data collection of PubMed publications, NIH-funded projects and project costs associated with drugs approved from 2010 to 2019 has been previously described. 6 Products approved by the FDA from 2010 to 2019 (new drug application or biologics license application [type 1]), excluding those derived from blood or tissue, diagnostic agents, vaccines, and antimicrobials, and dates of first approval were identified from annual FDA reports. 32 , 33 Drug targets were identified from published literature 34 , 35 or the Therapeutic Targets Database. 36
Publications from 1960 to 2020 were identified in PubMed. Projects funded by the NIH from 2000 to 2020 were identified using the NIH REPORTER application programming interface. Projects were identified by NIH project number comprising the activity code, awarding institute, and number. Data on each project included the start year, end year, and costs for each fiscal year, subproject, or supplemental award. The analysis included phase-specific clinical success rates, 19 average NIH costs for phased clinical trials, 37 average industry investments, 19 , 20 and drug-specific industry costs. 20
Derivation of NIH Costs
Funding from the NIH for publications (PMIDs) associated with study drugs or their targets was identified in NIH REPORTER using methods described previously 6 , 28 (eMethods in Supplement 1 ). Briefly, PMIDs were identified in PubMed using optimized search terms for drugs (eTable 1A in Supplement 1 ) or targets (eTable 1B in Supplement 1 ) as well as automatic term mapping protocols, including medical subject heading terms and Boolean modifiers. The PMIDs were indexed by PubMed Identifier, publication year, and search terms. Projects funded by the NIH that were associated with PMIDs were identified using the REPORTER publication link tables. The PMIDs were further associated with 1 fiscal year of project funding (project year) and total project costs for the year corresponding to the publication year. Project years and costs were not assigned to PMIDs published after the product’s first FDA approval, before the project start year, or more than 4 years after the project end year. Drug-specific costs were calculated from 2000 through the date of first FDA approval. To account for lags between funding and publication, 38 PMIDs with publication dates 1 to 4 years after the project end year were associated with the project end year. The PMIDs identified by drug search were categorized as applied research, which included development. The PMIDs identified by target search, but not a drug search, were categorized as basic research. Project years and costs were categorized as applied research if 1 or more PMIDs associated with that project year were identified by drug search and categorized as basic research if none of the associated PMIDs were identified in drug searches. Duplicate PMIDs, project years, and costs were eliminated independently for each calculation.
The first drug associated with a novel biological target was classified as first to target. 34 , 35 , 39 Applied research costs were estimated from costs identified through the drug search. Basic research costs were estimated from costs identified in searches for targets of first-to-target drugs. Averages were calculated after 95th percentile outlier elimination to account for searches with poor specificity. The average number of drugs per target was recalculated from Santos et al 35 after excluding products derived from blood or tissue, diagnostic agents, vaccines, and antimicrobials. Spending from the NIH on failed clinical trials was estimated from phase transition rates 19 and phase-specific NIH costs. 37 Compounded 3% or 7% discount rates 30 , 31 or a 10.5% cost of capital 19 were calculated from the project year to first FDA approval.
Statistical Methods
Product-specific costs were compared for 81 first-in-class drugs with NIH costs estimated in this analysis and 63 drugs with industry costs described by Wouters 20 using univariate regression in which Cost i = β 0 + β 1 Source i in which Cost i is the estimated NIH cost for research associated with the product or reported industry costs; Source i is an indicator variable with a value of 0 for NIH costs and 1 for industry costs; β 0 estimates the median and 95% CI for NIH spending; and β 1 estimates the median and 95% CI for the difference between NIH and industry spending. Costs were inflation-adjusted to 2018. Analyses were performed in Excel (Microsoft), PostgreSQL (PostgreSQL Global Development Group), or Python. All tests were 2 tailed. A 2-sided P < .05 was considered statistically significant.
Descriptive Data
The FDA approved 356 drugs from 2010 to 2019, including 336 associated with 217 known targets. PubMed searches for drug names identified 229 000 PMIDs, while searches for known drug targets identified 1.9 million publications, of which 21.4% had NIH funding ( Table 1 ). Funding from the NIH funding was identified in 310 of 356 drug searches (87%) and in all 217 target searches ( Table 1 ). Overall, this analysis identified NIH-funded research associated with 354 of 356 products (99.4%) approved from 2010 to 2019. The products without NIH funding were a chelating agent and osmotic laxative.
Table 1. NIH Funding for Basic and Applied Research Associated With 356 NMEs Approved by the FDA, 2010-2019.
Abbreviations: FDA, US Food and Drug Administration; NA, not applicable; NIH, National Institutes of Health; NME, new molecular entity; REPORTER, Research Portfolio Online Reporting Tools.
PubMed search performed with drug name and synonyms.
PubMed search performed with name of biological target.
Total is nonadditive due to publications identified in drug and target searches.
Publications identified in a drug search are classified as applied research. Publications identified in a target search, but not a drug search, are classified as basic research.
Funding from the NIH totaled $187 billion; $31 billion (17%) represented applied research on approved drugs, and $156 billion (83%) represented basic research on drug targets ( Table 1 ). Figure 1 shows annual publications, NIH project (funding) years, and costs leading to first FDA approval.
Figure 1. National Institutes of Health (NIH) Funding for Basic and Applied Research Associated With Drugs Approved From 2010 to 2019 by Year Before First Approval.
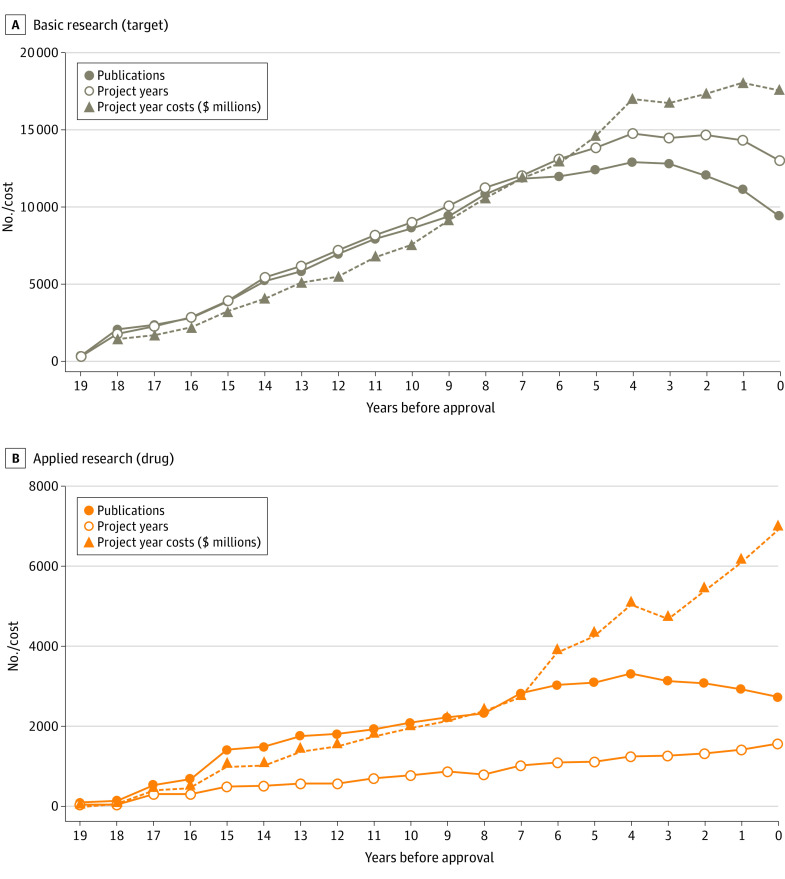
Publications (PMIDs), NIH-funded project years, and project year costs for basic research on 87 novel drug targets with first-to-target drugs (A) and for applied research on 356 drugs (B) approved from 2010 to 2019. Data are shown without applied discount rates.
Research projects and research-related programs, which typically support investigator-initiated research, provided 40.2% of NIH funding, including 43.2% of basic research costs and 24.8% of applied research costs. However, research program projects and centers as well as cooperative agreements (including clinical translational science awards), which typically contribute infrastructure or shared research capabilities, comprised 46.2% of total costs, 42.4% of basic research costs, and 65.6% of applied research costs (eFigure 1 in Supplement 1 ).
NIH Investment in Basic Research on Novel Targets
Of the 356 approvals, 86 (24.2%) were first-to-target products. Figure 1 A shows NIH-funded publications, project years, and NIH costs associated with these targets leading to first-to-target product launch. Funding from the NIH was identified for all 86 targets (eTable 2 in Supplement 1 ).
Figure 2 A shows NIH costs per novel drug target with no discount rate or 3% and 7% discount rates. After 95th percentile outlier elimination, the mean (SD) NIH cost for research on a novel drug target before a first-to-target product approval was $1.34 ($1.43) billion (3% discount, $1.63 [$1.74] billion; 7% discount, $2.15 [$1.66] billion; 10.5% cost of capital, $2.85 [$3.15] billion) ( Table 2 ). Outliers included searches for CD-4, B-cell lymphoma 2, and epidermal growth factor receptor, which returned publications not explicitly associated with the drug target. Calculations without outlier elimination are shown in eTable 3 in Supplement 1 .
Figure 2. National Institutes of Health (NIH) Costs for Basic and Applied Research Associated With Drugs Approved From 2010 to 2019.
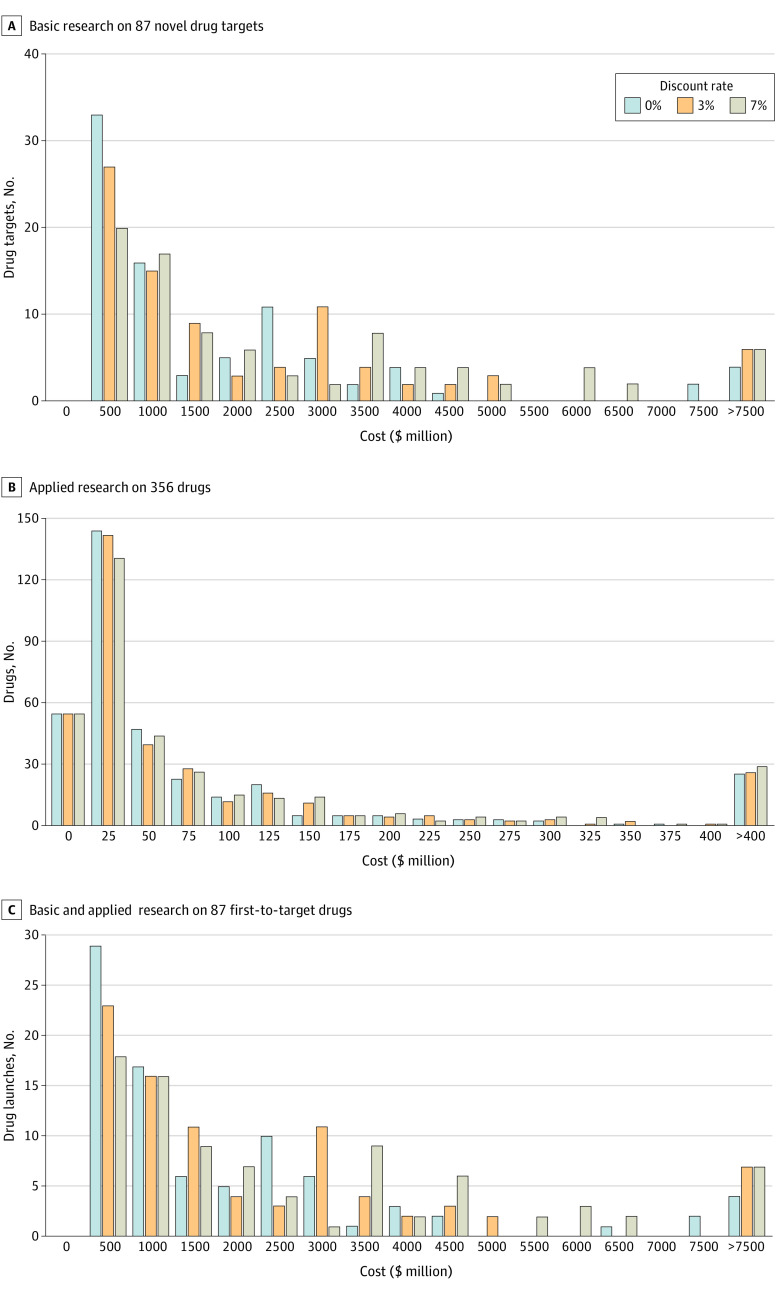
All costs were calculated to the year of drug approval with no discount rate or discount rates of 3% or 7% for years before approval. A, Costs for the NIH for basic research on 87 novel targets before the launch of a first-to-target product identified by searching for a drug target, but not the drug itself. B, Costs for the NIH for published applied research on 356 drugs identified by searching for the drug. C, Costs for the NIH for basic and applied research associated with 87 first-to-target drugs.
Table 2. NIH Costs for Basic and Applied Research Associated With NMEs Approved From 2010 to 2019.
Abbreviations: FDA, US Food and Drug Administration; NA, not applicable; NIH, National Institutes of Health; NME, new molecular entity.
All data are in millions.
Discount rates calculated on years before drug approval. The 3% and 7% discount rates are typically used to assess government investment. The 10.5% cost of capital is typically used to estimate industry costs of drug development.
The first-to-target drug is the first FDA-approved product associated with a novel biological target.
Mean (SD) NIH cost for published basic and applied research for 81 first-to-target drugs calculated after 95th percentile outlier elimination with estimated cost of phased development failures.
Mean (SD) NIH cost for published basic research on novel drug targets (n = 81) to year of first drug approval calculated after 95th percentile outlier elimination.
Mean (SD) cost for published applied research on drugs (n = 356) approved from 2010 to 2019 calculated after 95th percentile outlier elimination.
Funding from the NIH per drug for phased trials of failed drugs.
Spillovers from basic research based on 2.85 drugs associated with each biological target.
NIH Investment in Applied Research on Drug Products
Figure 1 B shows NIH-funded publications, project years, and NIH costs associated with applied research on 356 drugs through the year of approval. Before first approval, 301 of 356 products (84.5%) had NIH research funding (eTable 4 in Supplement 1 ). Figure 2 B shows cumulative NIH costs for applied research with no discount rate or 3% and 7% discount rates. After 95th percentile outlier elimination, the mean (SD) NIH cost for applied research before approval was $51.8 ($96.8) million (3% discount, $58.5 [$111.9] million; 7% discount, $69.4 [$137.8] million; 10.5% cost of capital, $81.4 [$168.3] million) ( Table 2 ). Outliers included searches failing to distinguish applied research on the approved product from basic research on the corresponding natural compound (ie, clotting factors, hormones, α-1 antitrypsin). Results without outlier elimination are shown in eTable 3 in Supplement 1 .

Accounting for NIH Funding for Failed Product Candidates
The NIH costs calculation associated with failed clinical trials is shown in eTable 5 in Supplement 1 . Based on reported phase transition rates, 19 8.53 phase 1 trials, 5.08 phase 2 trials, and 1.79 phase 3 trials were conducted for each product approved. With NIH costs of $5.7 million for phase 1, $7.2 million for phase 2, and $3.9 million for phase 3, 37 estimated NIH costs for clinical trials of failed candidates were $75.4 million for each product approval (3% discount, $80.6 million; 7% discount, $88.6 million; 10.5% cost of capital, $96.8 million) ( Table 2 ).
Total NIH Investment to Launch First Drug Product Associated With Novel Targets
Total NIH costs were calculated for 86 first-to-target products as the sum of NIH costs for basic research on the target, applied research on the drug, and phased clinical trials of failed compounds. The distribution of costs is shown in Figure 2 C. After 95th percentile outlier elimination, mean (SD) NIH costs before a first-to-target product launch was $1.44 ($1.37) billion (3% discount, $1.73 [$1.66] billion; 7% discount, $2.24 [$2.18] billion; 10.5% cost of capital, $2.96 [$3.11] billion) ( Table 2 ). Data without outlier elimination are shown in eTables 2 and 4 in Supplement 1 .
Comparing NIH and Industry Investments
DiMasi et al 19 estimated average industry spending on 106 drugs approved from 1990 to 2010 at $1.5 billion or $2.8 billion with a 10.5% cost of capital (inflation-adjusted to 2018). Using different methods, Wouters et al 20 reported an average industry spending on 63 drugs approved from 2009 to 2018 of $374.1 million, (95% CI, $301.9 million to $464.2 million) or $1.6 billion (95% CI, $1.27 billion to $1.89 billion) with a 10.5% cost of capital.
Spending from the NIH per approval for 81 first-to-target products was significantly greater than reported industry spending on 63 drugs 20 before accounting for clinical failures, cost of capital, or discount rates (difference, −$1998.4 million; 95% CI, −$3302.1 million to −$694.6 million; P = .003) or with accounting for clinical failures (difference, −$1415.8 million; 95% CI, −$2731.4 million to $100.2 million; P = .04) ( Table 3 ). Spending from the NIH was not less than industry spending when industry costs were estimated with clinical failures and a 10.5% cost of capital, and NIH spending was estimated with clinical failures and either a 3% discount rate (difference, −$1435.3 million; 95% CI, −$3114.6 million to $244.0 million; P = .09) or a 7% discount rate (difference, −$2436.3 million; 95% CI, −$4782.1 million to −$90.5 million; P = .04) ( Table 3 ). Investment from the NIH and the industry was not significantly different when industry spending was estimated with clinical failures, prehuman costs 19 (30.8% real costs), and a 10.5% cost of capital, and when NIH costs were estimated with clinical failures and either a 3% discount rate (difference, −$393.8 million; 95% CI, −$2120.5 million to $1332.9 million; P = .65) or 7% discount rate (difference, −$1394.8 million; 95% CI, −$3774.8 million to $985.2 million; P = .25) ( Table 3 ).
Table 3. Comparison of the NIH Investment in Basic and Applied Research on FDA-Approved Drugs and Estimated Industry Investment in Development Accounting for Clinical Failures and the Time-Value of Investments.
Abbreviations: FDA, US Food and Drug Administration; NIH, National Institutes of Health; OMB, US Office of Management and Business.
Clinical failures are estimated as the average NIH costs associated with each phase of clinical development and published phase transition rates. Spending from the NIH on clinical failures was calculated using phase transition rates described by DiMasi et al. 19 Industry spending on clinical failures was calculated using phase transition rates described by Wong et al. 23
Investment by the NIH in preclinical research was included in applied research. Industry costs reported by Wouters et al 20 included preclinical research with phase 1. In a separate sensitivity analysis, Wouters et al 20 estimated preclinical costs to be 43% of clinical costs based on data reported by DiMasi et al. 19
Univariate linear regression performed with NIH funding associated with drugs approved from 2010 to 2019 with novel drug targets (n = 81) or reported industry spending (n = 63) with an indicator variable for industry of 1 or NIH of 0.
Investment by the NIH was calculated with no discount rate or the 3% or 7% discount rates for government spending recommended by OMB.
Industry investment was calculated with a 10.5% cost of capital based on DiMasi et al 19 and Wouters et al. 20
Intercept of linear regression model estimates average NIH spending on basic and applied research, which included preclinical research and phased clinical trials.
Coefficient of linear regression model estimates the difference between NIH spending and industry spending. Negative values indicated NIH spending greater than industry spending.
Includes prehuman studies as 30.8% of total costs or 42.9% of costs calculated with 10.5% cost of capital as described by DiMasi et al. 19
Spillover Effects From Basic Research on Drug Targets
Santos et al 40 cataloged 893 biological targets for FDA-approved products (1578) through June 2015, of which 1467 (93.0%) met inclusion criteria for this study. These products were associated with 515 biological targets, an average of 2.85 products per target (eFigure 2 in Supplement 1 ).
Accounting for spillovers of basic research on novel drug targets to 2.85 product approvals, the NIH cost for basic research per approval was $471.8 million (3% discount, $572.2 million; 7% discount, $753.4 million; 10.5% cost of capital, $1.0 billion) ( Table 2 ). Accounting for spillover effects from basic research on drug targets, costs of applied research, product failures, and discount rates or cost of capital, the estimated NIH investment per approval was $599.0 million (3% discount, $711.3 million; 7% discount, $911.4 million; 10.5% cost of capital, $1179 million). Estimated NIH spending was lower than the reported average industry spending 19 but within the 95% CI of per drug spending. 20
In this cross-sectional study, evidence suggests the public sector makes substantial contributions to the foundational knowledge on which drug approvals are based, 1 , 2 , 4 , 6 , 7 , 8 , 41 , 42 but less to patents 6 , 9 or development. 2 , 3 , 37 , 43 Conversely, the industry is primarily responsible for product development and sponsored more than 99% of the product launches in this data set. 6
The objective of this work was to compare NIH investments in recent drug approvals with reported investment by the industry. This required an accounting for NIH spending with costs for basic research on the targets for these drugs, applied research on the approved products, phased clinical trials of failed products, and the recommended discount rates for government spending. 30 , 31 This accounting adheres closely to methods used to estimate industry investment, 19 , 20 while also recognizing fundamental differences in the nature of public and private sector investment in prevailing economic theories. 10
These analyses suggest that NIH project costs for basic or applied research associated with the products approved from 2010 to 2019 were significantly greater than reported industry spending. Costs for the NIH were also higher than industry costs when both included spending on failed clinical trials of candidate products. Including clinical failures, NIH investment (calculated with either a 3% or 7% discount rate) was not less than industry investment calculated with a 10.5% cost of capital. Investment from the NIH calculated with clinical failures and a 3% or 7% discount rate was also not less than industry investment calculated with clinical failures, additional costs of prehuman research, and 10.5% cost of capital. These results suggest that NIH investments in pharmaceutical innovation are comparable with those made by industry.
While including the cost of capital in estimates of the industry’s investment in pharmaceutical innovation is controversial 44 and estimates of this rate vary, 45 , 46 consideration of the cost of capital is normative in finance theory and practice. These calculations are also consistent with prevailing economic theories that view private sector investment as inherently productive in that it typically generates a return on investment. In this context, the cost of capital represents the opportunity cost or financial risk that long-term capital investments in drug discovery and development may not achieve normal returns on investment.
There is no theoretical basis for applying an equivalent cost of capital to government spending. Prevailing economic theories treat government funding as nonproductive in that it is not expected to provide a return on investment. The 3% and 7% discount rates recommended by the US Office of Management and Budget for government spending 30 , 31 have distinct theoretical foundations. The 3% discount rate on federal spending approximates the historical cost of government borrowing and, consequently, the full cost of government spending. 31 , 47 The 7% discount rate represents the average productivity of private sector investments and is interpreted as a measure of the opportunity cost to the economy if public sector spending crowds out and reduces private sector investment. 30 , 48 Given evidence that NIH funding for biomedical research stimulates, rather than reduces, private sector investment, 49 estimating NIH investment with the 3% discount rate may be most consistent with prevailing economic principles.
This analysis did include an NIH spending calculation with the 10.5% cost of capital. This value provides an estimate of the additional costs that the industry would incur in the absence of NIH spending. Comparing these estimated cost savings with those of DiMasi et al 19 or Wouters et al 20 of industry investment suggests that industry costs would be more than double in the absence of the NIH contributions.
This work also recognizes that economic efficiencies may arise through spillover of knowledge or capabilities gained from NIH-funded basic research to applications by multiple firms or multiple products. 8 , 29 , 35 , 43 , 50 Such spillovers would reduce the estimated NIH cost per approval. Considering only potential spillovers from NIH-funded basic research on drug targets to multiple products directed at the same targets, NIH spending per drug was within the range of actual industry spending. 20 Spending from the NIH was estimated with either a 3% or 7% discount rate was lower than industry spending calculated with the 10.5% cost of capital.
Policy Implications
Science and innovation policy remains grounded in a model in which government investments in basic research generate scientific capital that can be commercialized by industry for social and economic benefit. This model is exemplified by NIH spending for basic biomedical science, which plays an enabling role in pharmaceutical innovation. 1 , 3 , 6 , 7 , 28 , 49 Emerging economic theory formalizes this model by contextualizing government funding for research as an early-stage investment in innovation. 10 , 11 , 12 , 13 , 15 , 16 , 17 These theories further posit that, as early-stage investors, government or the public sector it represents could expect social or economic returns commensurate with those of comparable investments by the private sector. 10 , 15 , 16
The present study was predicated on this concept that NIH spending represents an investment that can be meaningfully compared with investment by the industry. In this context, the finding that the magnitude of NIH investment in new drugs is comparable with that of the industry suggests that returns to the public and private sector should also be comparable. To achieve this, public policy associated with drug pricing, 51 corporate profit, 52 and commercial applications of government-funded invention 53 should be calibrated to provide an equitable distribution of returns between the public and private sectors. 10 , 15 , 16 The present results may provide a cost basis for considering not only the private rate of return to industry or the economy, but also the social return on investments, 40 including the multiplex elements associated with health. 54 , 55
Limitations
First, this analysis is limited by the sensitivity and specificity of PubMed searches, right censoring of the data collection, and reported false-positive and false-negative associations between PMIDs and NIH projects in REPORTER. 38 Search terms may not identify NIH funding for research tools, pharmaceutical modalities, or process development, which may underestimate total NIH costs.
Second, NIH costs for each publication were estimated as 1 fiscal year of project funding. This is consistent with evidence that 5-year NIH grants produce a median of 5 publications 56 but may underestimate NIH costs for studies spanning multiple years.
Third, NIH funding in REPORTER represents a fraction of public sector funding for biomedical research and does not include funding from other agencies or governments, nongovernment organizations, academic institutions, or nonprofit organizations. This analysis also did not include contract funding, research and development tax credits, or vouchers. This would underestimate the public sector contribution to pharmaceutical innovation.
Fourth, this study considered only spillovers from basic research on drug targets. Spillovers may also emerge from NIH funding for research training, infrastructure, or capabilities. This would not affect the total NIH costs but would underestimate the gain from economic efficiencies.
Conclusions
This cross-sectional study found that NIH investment in drugs approved from 2010 to 2019 was not less than investment by industry, with comparable accounting for basic and applied research, failed clinical trials, and cost of capital or discount rates. The relative scale of NIH and industry investment may provide a cost basis for calibrating the balance of social and private returns from investments in pharmaceutical innovation.
eTable 1. PubMed drug and target search terms
eTable 2. NIH costs associated with 86 novel drug targets with quartile and 95% percentile values
eTable 3. NIH costs for basic and applied research related to NMEs approved 2010-2019 without outlier elimination
eTable 4. NIH costs associated with 356 drugs approved 2010-2019 with quartile and 95% percentile values
eTable 5. Calculation of the NIH contribution to phased clinical trials of failed clinical compounds for each product approval
eFigure 1. NIH funding for basic and applied research related to drugs approved 2010-2019 by Project Activity Code
eFigure 2. Number of approved FDA drugs (through June 2015) associated with 515 drug targets
eReferences.
Data sharing statement
- 1. Toole AA. The impact of public basic research on industrial innovation: evidence from the pharmaceutical industry. Res Policy. 2012;41(1):1-12. doi: 10.1016/j.respol.2011.06.004 [ DOI ] [ Google Scholar ]
- 2. Sampat BN, Lichtenberg FR. What are the respective roles of the public and private sectors in pharmaceutical innovation? Health Aff (Millwood). 2011;30(2):332-339. doi: 10.1377/hlthaff.2009.0917 [ DOI ] [ PubMed ] [ Google Scholar ]
- 3. Chakravarthy R, Cotter K, DiMasi J, Milne C-P, Wendel N. Public- and private-sector contributions to the research and development of the most transformational drugs in the past 25 years: from theory to therapy. Ther Innov Regul Sci. 2016;50(6):759-768. doi: 10.1177/2168479016648730 [ DOI ] [ PubMed ] [ Google Scholar ]
- 4. Stevens AJ, Jensen JJ, Wyller K, Kilgore PC, Chatterjee S, Rohrbaugh ML. The role of public-sector research in the discovery of drugs and vaccines. N Engl J Med. 2011;364(6):535-541. doi: 10.1056/NEJMsa1008268 [ DOI ] [ PubMed ] [ Google Scholar ]
- 5. Nayak RK, Avorn J, Kesselheim AS. Public sector financial support for late stage discovery of new drugs in the United States: cohort study. BMJ. 2019;367:l5766. doi: 10.1136/bmj.l5766 [ DOI ] [ PMC free article ] [ PubMed ] [ Google Scholar ]
- 6. Cleary E, Jackson MJ, Ledley F. Government as the first investor in biopharmaceutical innovation: evidence from new drug approvals, 2010-2019. Accessed March 30, 2023. doi: 10.36687/inetwp133 [ DOI ]
- 7. McNamee LM, Walsh MJ, Ledley FD. Timelines of translational science: from technology initiation to FDA approval. PLoS One. 2017;12(5):e0177371. doi: 10.1371/journal.pone.0177371 [ DOI ] [ PMC free article ] [ PubMed ] [ Google Scholar ]
- 8. Azoulay P, Li D, Zivin JSG, Sampat BN. Public R&D investments and private-sector patenting: evidence from NIH funding rules. Rev Econ Stud. 2019;86(1):117-152. doi: 10.1093/restud/rdy034 [ DOI ] [ PMC free article ] [ PubMed ] [ Google Scholar ]
- 9. Li D, Azoulay P, Sampat BN. The applied value of public investments in biomedical research. Science. 2017;356(6333):78-81. doi: 10.1126/science.aal0010 [ DOI ] [ PubMed ] [ Google Scholar ]
- 10. Mazzucato M, Li H. The Entrepreneurial State: Socializing Both Risks and Rewards. Real-World Economics Review; 2018:84. [ Google Scholar ]
- 11. Mazzucato M. An entrepreneurial society needs an entrepreneurial state. Harv Bus Rev. 2016;1-4. https://hbr.org/2016/10/an-entrepreneurial-society-needs-an-entrepreneurial-state [ Google Scholar ]
- 12. Mazzucato M. From market fixing to market-creating: a new framework for innovation policy. Ind Innov. 2016;23(2):140-156. doi: 10.1080/13662716.2016.1146124 [ DOI ] [ Google Scholar ]
- 13. Block FL, Keller MR. State of Innovation: the US Government’s Role in Technology Development. Routledge; 2015. doi: 10.4324/9781315631905 [ DOI ] [ Google Scholar ]
- 14. Hopkins M, Lazonick W. Who invests in the high-tech knowledge base? Accessed March 30, 2023. doi: 10.2139/ssrn.2638091 [ DOI ]
- 15. Lazonick W, Mazzucato M. The risk-reward nexus in the innovation-inequality relationship: who takes the risks? who gets the rewards? Ind Corp Change. 2013;22(4):1093-1128. doi: 10.1093/icc/dtt019 [ DOI ] [ Google Scholar ]
- 16. Laplane A, Mazzucato M. Socializing the risks and rewards of public investments: economic, policy, and legal issues. Res Policy. 2020;49:100008. doi: 10.1016/j.repolx.2020.100008 [ DOI ] [ Google Scholar ]
- 17. Lazonick W, Hopkins M, Jacobson K, Sakinç M, Tulum ÖUS. Pharma's financialized business model. Accessed March 30, 2023. doi: 10.2139/ssrn.3035529 [ DOI ]
- 18. Mazzucato M, Li HL. A market shaping approach for the biopharmaceutical industry: governing innovation towards the public interest. J Law Med Ethics. 2021;49(1):39-49. doi: 10.1017/jme.2021.8 [ DOI ] [ PubMed ] [ Google Scholar ]
- 19. DiMasi JA, Grabowski HG, Hansen RW. Innovation in the pharmaceutical industry: new estimates of R&D costs. J Health Econ. 2016;47:20-33. doi: 10.1016/j.jhealeco.2016.01.012 [ DOI ] [ PubMed ] [ Google Scholar ]
- 20. Wouters OJ, McKee M, Luyten J. Estimated research and development investment needed to bring a new medicine to market, 2009-2018. JAMA. 2020;323(9):844-853. doi: 10.1001/jama.2020.1166 [ DOI ] [ PMC free article ] [ PubMed ] [ Google Scholar ]
- 21. Rennane S, Baker L, Mulcahy A. Estimating the cost of industry investment in drug research and development: a review of methods and results. Inquiry. 2021;58:469580211059731. [ DOI ] [ PMC free article ] [ PubMed ] [ Google Scholar ]
- 22. DiMasi J. Causes of clinical failures vary widely by therapeutic class, phase of study. Tufts CSDD Impact Report. 2013;15(5):1-4. [ Google Scholar ]
- 23. Wong CH, Siah KW, Lo AW. Estimation of clinical trial success rates and related parameters. Biostatistics. 2019;20(2):273-286. doi: 10.1093/biostatistics/kxx069 [ DOI ] [ PMC free article ] [ PubMed ] [ Google Scholar ]
- 24. Moses H III, Matheson DH, Cairns-Smith S, George BP, Palisch C, Dorsey ER. The anatomy of medical research: US and international comparisons. JAMA. 2015;313(2):174-189. doi: 10.1001/jama.2014.15939 [ DOI ] [ PubMed ] [ Google Scholar ]
- 25. Sampat BN, Buterbaugh K, Perl M. New evidence on the allocation of NIH funds across diseases. Milbank Q. 2013;91(1):163-185. doi: 10.1111/milq.12005 [ DOI ] [ PMC free article ] [ PubMed ] [ Google Scholar ]
- 26. Ballreich JM, Gross CP, Powe NR, Anderson GF. Allocation of National Institutes of Health funding by disease category in 2008 and 2019. JAMA Netw Open. 2021;4(1):e2034890-e2034890. doi: 10.1001/jamanetworkopen.2020.34890 [ DOI ] [ PMC free article ] [ PubMed ] [ Google Scholar ]
- 27. National Science Foundation . Definitions of research and development: an annotated compilation of official sources. Accessed July 27, 2022. https://ncses.nsf.gov/pubs/ncses22209 [ Google Scholar ]
- 28. Galkina Cleary E, Beierlein JM, Khanuja NS, McNamee LM, Ledley FD. Contribution of NIH funding to new drug approvals 2010-2016. Proc Natl Acad Sci U S A. 2018;115(10):2329-2334. doi: 10.1073/pnas.1715368115 [ DOI ] [ PMC free article ] [ PubMed ] [ Google Scholar ]
- 29. Cleary EG, Ledley FD. NIH funding for research underlying new cancer therapies. Lancet Oncol. 2020;21(6):755-757. doi: 10.1016/S1470-2045(20)30235-7 [ DOI ] [ PubMed ] [ Google Scholar ]
- 30. Office of Management and Budget . M-03-21, OMB circular No. A-4, "regulatory analysis". Accessed July 27, 2022. https://www.whitehouse.gov/wp-content/uploads/2017/11/2003-M-03-21-OMB-Circular-No.-A-4-Regulatory-Analysis.pdf
- 31. National Archives . Circular A-94: guidelines and discount rates for benefit-cost analysis of federal programs. Accessed July 27, 2022. https://obamawhitehouse.archives.gov/sites/default/files/omb/assets/a94/a094.pdf
- 32. US Food and Drug Administration . New drugs at FDA: CDER’s new molecular entities and new therapeutic biological products. Accessed March 29, 2023. https://www.fda.gov/drugs/development-approval-process-drugs/new-drugs-fda-cders-new-molecular-entities-and-new-therapeutic-biological-products
- 33. US Food and Drug Administration . 2022 Biological approvals. Accessed March 29, 2023. https://www.fda.gov/vaccines-blood-biologics/development-approval-process-cber/2022-biological-approvals
- 34. Eder J, Sedrani R, Wiesmann C. The discovery of first-in-class drugs: origins and evolution. Nat Rev Drug Discov. 2014;13(8):577-587. doi: 10.1038/nrd4336 [ DOI ] [ PubMed ] [ Google Scholar ]
- 35. Santos R, Ursu O, Gaulton A, et al. A comprehensive map of molecular drug targets. Nat Rev Drug Discov. 2017;16(1):19-34. doi: 10.1038/nrd.2016.230 [ DOI ] [ PMC free article ] [ PubMed ] [ Google Scholar ]
- 36. Zhu F, Han B, Kumar P, et al. Update of TTD: therapeutic target database. Nucleic Acids Res. 2010;38(Database issue)(suppl 1):D787-D791. doi: 10.1093/nar/gkp1014 [ DOI ] [ PMC free article ] [ PubMed ] [ Google Scholar ]
- 37. Zhou E, Jackson MJ, Ledley FD. NIH contribution to phased clinical development of drugs approved from 2010-2019: tech note. Accessed February 17, 2023.
- 38. Boyack KW, Jordan P. Metrics associated with NIH funding: a high-level view. J Am Med Inform Assoc. 2011;18(4):423-431. doi: 10.1136/amiajnl-2011-000213 [ DOI ] [ PMC free article ] [ PubMed ] [ Google Scholar ]
- 39. Lanthier M, Miller KL, Nardinelli C, Woodcock J. An improved approach to measuring drug innovation finds steady rates of first-in-class pharmaceuticals, 1987-2011. Health Aff (Millwood). 2013;32(8):1433-1439. doi: 10.1377/hlthaff.2012.0541 [ DOI ] [ PubMed ] [ Google Scholar ]
- 40. Lingane A, Olsen S. Guidelines for social return on investment. Calif Manage Rev. 2004;46(3):116-135. doi: 10.2307/41166224 [ DOI ] [ Google Scholar ]
- 41. Sampat BN. Academic patents and access to medicines in developing countries. Am J Public Health. 2009;99(1):9-17. doi: 10.2105/AJPH.2007.128769 [ DOI ] [ PMC free article ] [ PubMed ] [ Google Scholar ]
- 42. Beierlein JM, McNamee LM, Walsh MJ, Kaitin KI, DiMasi JA, Ledley FD. Landscape of innovation for cardiovascular pharmaceuticals: from basic science to new molecular entities. Clin Ther. 2017;39(7):1409-1425.e20. doi: 10.1016/j.clinthera.2017.06.001 [ DOI ] [ PubMed ] [ Google Scholar ]
- 43. Cockburn IM, Henderson RM. Publicly funded science and the productivity of the pharmaceutical industry. Innov Policy Econ. 2000;1:1-34. doi: 10.1086/ipe.1.25056140 [ DOI ] [ Google Scholar ]
- 44. Chit A, Chit A, Papadimitropoulos M, Krahn M, Parker J, Grootendorst P. The opportunity cost of capital: development of new pharmaceuticals. Inquiry. 2015;52:0046958015584641. [ DOI ] [ PMC free article ] [ PubMed ] [ Google Scholar ]
- 45. Baras AI, Baras AS, Schulman KA. Drug development risk and the cost of capital. Nat Rev Drug Discov. 2012;11(5):347-348. doi: 10.1038/nrd3722 [ DOI ] [ PubMed ] [ Google Scholar ]
- 46. Harrington SE. Cost of capital for pharmaceutical, biotechnology, and medical device firms. The Oxford Handbook of the Economics of the Biopharmaceutical Industry. eds, Danzon PM, Nicholson S. 2012:75-99. doi: 10.1093/oxfordhb/9780199742998.013.0004 [ DOI ] [ Google Scholar ]
- 47. Council of Economic Advisors . Discounting for public policy: theory and recent evidence on the merits of updating the discount rate. Accessed July 27, 2022. https://obamawhitehouse.archives.gov/sites/default/files/page/files/201701_cea_discounting_issue_brief.pdf
- 48. David PA, Hall BH, Toole AA. Is public R&D a complement or substitute for private R&D? a review of the econometric evidence. Res Policy. 2000;29(4-5):497-529. doi: 10.1016/S0048-7333(99)00087-6 [ DOI ] [ Google Scholar ]
- 49. Toole AA. Does public scientific research complement private investment in research and development in the pharmaceutical industry? J Law Econ. 2007;50(1):81-104. doi: 10.1086/508314 [ DOI ] [ Google Scholar ]
- 50. DiMasi JA, Paquette C. The economics of follow-on drug research and development: trends in entry rates and the timing of development. Pharmacoeconomics. 2004;22(2)(suppl 2):1-14. doi: 10.2165/00019053-200422002-00002 [ DOI ] [ PubMed ] [ Google Scholar ]
- 51. Wouters OJ, Berenbrok LA, He M, Li Y, Hernandez I. Association of research and development investments with treatment costs for new drugs approved from 2009 to 2018. JAMA Netw Open. 2022;5(9):e2218623-e2218623. doi: 10.1001/jamanetworkopen.2022.18623 [ DOI ] [ PMC free article ] [ PubMed ] [ Google Scholar ]
- 52. Ledley FD, McCoy SS, Vaughan G, Cleary EG. Profitability of large pharmaceutical companies compared with other large public companies. JAMA. 2020;323(9):834-843. doi: 10.1001/jama.2020.0442 [ DOI ] [ PMC free article ] [ PubMed ] [ Google Scholar ]
- 53. Markel H. Patents, profits, and the American people—the Bayh-Dole Act of 1980. N Engl J Med. 2013;369(9):794-796. doi: 10.1056/NEJMp1306553 [ DOI ] [ PubMed ] [ Google Scholar ]
- 54. Lakdawalla DN, Doshi JA, Garrison LP Jr, Phelps CE, Basu A, Danzon PM. Defining elements of value in health care-a health economics approach: an ISPOR Special Task Force report [3]. Value Health. 2018;21(2):131-139. doi: 10.1016/j.jval.2017.12.007 [ DOI ] [ PubMed ] [ Google Scholar ]
- 55. Stiglitz JE. Measuring What Counts: The Global Movement for Well-Being. The New Press; 2019. [ Google Scholar ]
- 56. Li D, Agha L. Research funding: big names or big ideas: do peer-review panels select the best science proposals? Science. 2015;348(6233):434-438. doi: 10.1126/science.aaa0185 [ DOI ] [ PubMed ] [ Google Scholar ]
Associated Data
This section collects any data citations, data availability statements, or supplementary materials included in this article.
Supplementary Materials
- View on publisher site
- Collections
Similar articles
Cited by other articles, links to ncbi databases.
- Download .nbib .nbib
- Format: AMA APA MLA NLM
Add to Collections
- U.S. Department of Health & Human Services

- Virtual Tour
- Staff Directory
- En Español
You are here
News releases.
News Release
Tuesday, June 12, 2012
Five more pharmaceutical companies join NIH initiative to speed therapeutic discovery
Nearly 60 compounds from industry collaborators now publicly available.
Five additional pharmaceutical companies have joined a National Institutes of Health-led effort to help scientists research promising new treatments for patients. Funding and molecular compound information is available now for the initial phase of the recently unveiled Discovering New Therapeutic Uses for Existing Molecules program. This NIH-industry collaboration will match researchers with 58 compounds to test ideas for new therapeutic uses. Since the launch of the program last month, the total number of compounds the companies are making available has more than doubled.
Abbott, Bristol-Myers Squibb Company, GlaxoSmithKline, Janssen Pharmaceutical Research & Development, L.L.C., and Sanofi have joined Pfizer, AstraZeneca, and Eli Lilly and Company in this innovative approach to research.
The NIH's new National Center for Advancing Translational Sciences (NCATS) created the Therapeutics Discovery program to help re-engineer the research pipeline. By crowdsourcing compounds that already have cleared several key steps in the development process, including safety testing in humans, scientists nationwide have the opportunity to contribute their expertise to advancing these resources for new disease therapies.
The eight participating companies will provide their compounds and related data, which were determined by the NIH to meet specific eligibility criteria. For example, each compound must have advanced to clinical studies but been unsuccessful in its original therapeutic indication or not pursued for business reasons. Preliminary information about the compounds, including mechanism of action, route of administration, and any limitations in use based on safety and tolerability, are available at http://ncats.nih.gov/therapeutics-directory.html .
"Each company participating in this innovative collaboration has made substantial research and development investments to advance these compounds to the point where they can be used in clinical studies," said Kathy L. Hudson, Ph.D., NCATS acting deputy director. "If researchers funded through this effort can demonstrate new uses for the compounds, they could significantly reduce the amount of time it takes to get a treatment to patients in need."
For the pilot phase of the program, in fiscal year 2013, NCATS will provide up to $20 million to fund two- to three-year staged, cooperative agreement research grants. If specific milestones are met, funded researchers will conduct pre-clinical validation and clinical feasibility studies in the first stage, and proof-of-concept clinical trials in the second stage, to test whether one of the compounds may be effective against a previously unexplored disease target. The pilot phase also is intended to test the utility of the newly created template agreements by reducing the negotiation time that otherwise could delay the research.
Researchers who are interested in NCATS’ Therapeutics Discovery funding must submit a pre-application in response to the NIH Funding Opportunity Announcement at http://grants.nih.gov/grants/guide/pa-files/PAR-12-203.html by Aug. 14, 2012. Applicants must include details about how they would explore specific hypotheses related to one of the compounds that might be useful in a specific disease area. The pre-applications will undergo review by external experts, and while no funding will be provided at this stage, successful applicants will be notified to submit a full application for fiscal year 2013 funding.
For more information, visit ncats.nih.gov/therapeutics.html .
The National Center for Advancing Translational Sciences (NCATS) aims to catalyze the generation of innovative methods and technologies that will enhance the development, testing and implementation of diagnostics and therapeutics across a wide range of human diseases and conditions. For more information about NCATS, visit ncats.nih.gov .
About the National Institutes of Health (NIH): NIH, the nation's medical research agency, includes 27 Institutes and Centers and is a component of the U.S. Department of Health and Human Services. NIH is the primary federal agency conducting and supporting basic, clinical, and translational medical research, and is investigating the causes, treatments, and cures for both common and rare diseases. For more information about NIH and its programs, visit www.nih.gov .
NIH…Turning Discovery Into Health ®
Connect with Us
- More Social Media from NIH
Redirect Notice
Funding categories.
At any given time, NIH has over 1,000 active funding opportunities that use various funding instruments and announce a broad range of programs. Explore these categories for insight into the funding landscape and discover programs that may address your needs.
Research Training and Career Development
Training and career support for scientists, fellows, and trainees at the undergraduate, predoctoral, postdoctoral, early career, and mid-career levels.
Research and Development
NIH supports a wide variety of research and development programs, including capacity building, conferences and meetings, research projects (small, large, exploratory, pilots), and resource programs.
Construction and Modernization
NIH enhances biomedical research through support for construction, renovation, and modernization of new or existing physical infrastructure, e.g., buildings, facilities, laboratories, and fixed equipment.
Small Business
Support for U.S. small businesses (including minority-, women-, veteran-owned and HUBZone companies) to stimulate technological innovation, pursue research and development, and commercialize biomedical products in NIH areas of science.
Supplemental Funding
For currently funded NIH grants, supplemental funding may be available through competing revisions to expand a project's scope and administrative supplements to meet unanticipated costs, promote workforce diversity, or support career re-entry without a change of scope.
Loan Repayment Programs
To retain and recruit professionals in biomedical or biobehavioral careers, NIH repays educational debt in return for a commitment to engage in research relevant to our scientific mission.
NIH uses legally binding contracts to acquire goods and services (e.g., research and development work) to support research and research-related activities.
Other Transactions
Though statutory authority, NIH may fund unique legal agreements for a specific function, initiative, program, project, or research. These transactions are not grants, contracts, or cooperative agreements.
Challenges and Prizes
To address special problems and challenges in biomedical research, NIH competitions and prizes solicit, showcase, and support participants’ transformative solutions and achievements.
Extramural Diversity
Learn how diversity supports our mission, find opportunities to participate in diversity programs, and more.
Full list of Activity Codes

IMAGES