
- Science Notes Posts
- Contact Science Notes
- Todd Helmenstine Biography
- Anne Helmenstine Biography
- Free Printable Periodic Tables (PDF and PNG)
- Periodic Table Wallpapers
- Interactive Periodic Table
- Periodic Table Posters
- Science Experiments for Kids
- How to Grow Crystals
- Chemistry Projects
- Fire and Flames Projects
- Holiday Science
- Chemistry Problems With Answers
- Physics Problems
- Unit Conversion Example Problems
- Chemistry Worksheets
- Biology Worksheets
- Periodic Table Worksheets
- Physical Science Worksheets
- Science Lab Worksheets
- My Amazon Books

Capillary Action – What It Is and How It Works

Capillary action is fluid flow through a narrow tube or space from surface tension, cohesion, and adhesion . For example, if you place a thin tube into water, the water flows up the the tube. Other names for the phenomenon are capillarity, capillary motion, and wicking. Capillary action does not require the force of gravity. In fact, liquids often rise in narrow tube in opposition to gravity.
Forces in Capillary Action – How It Works
The three forces largely responsible for capillary action are surface tension, cohesion, and adhesion.
- Cohesion occurs when liquid molecules stick to each other. In the case of water molecules, cohesion is high because of hydrogen bonding between the molecules.
- Adhesion describes how well liquid molecules stick to surfaces. Water molecules stick to glass and plastic surfaces. In contrast, mercury atoms stick well to each other, but are not as attracted to a container surface.
- Surface tension is the tension on a liquid at its interface with air that minimizes the liquid surface area. Water and mercury both have high surface tension. In a narrow tube, the meniscus formed by these two liquids is curved. However, water curves toward the container wall, while mercury forms a rounded shape in the center of a capillary.
- Gravity affects how far a liquid rises within a capillary, as it exerts a downward pull on the liquid in a vertical tube.
Many liquids act like water and rise in a capillary tube. However, liquids like mercury rise to a level that is lower than that of the liquid surrounding the tube.
A convex meniscus forms when molecules are more attracted to each other than they are to the container. For example, mercury forms a convex meniscus in glass. A concave meniscus forms when molecules are more attracted to the container than they are to each other. For example, water forms a concave meniscus in glass. The meniscus shape depends on both the composition of the liquid and that of the container. For example, the meniscus of water in plastic is nearly flat.
Capillary Action Examples
There are many familiar examples of capillary action in everyday life:
- When you place a straw in a glass of water, the liquid level within the straw is higher than the height of the water in the glass.
- Capillary action causes the rise of damp in concrete and drywall.
- The lacrimal ducts (tear ducts) of the eyes continuously drain tears from the eye surface.
- A candle wick absorbs liquid wax that constantly supplies a candle flame. Paint brushes and lamp wicks pick up liquid the same way.
- Paper towels wick up water using capillary action.
- Fabrics that wick away perspiration also use capillary action.
The movement of water up the stems and trunks of plants (transpiration) involves capillary action, but it also relies on evaporation from leaves and osmotic pressure from roots.
Capillary Action Uses
Capillary action has several uses. For example:
- Fountain pens draw up ink using capillary action
- Thin layer and paper chromatography apply capillary action.
- Capillary tubes are thin tubes in science and medicine that draw small samples, such as blood.
Formula for the Height of the Meniscus
Be sure you measure the liquid level in a capillary tube, test tube, or buret at the meniscus line . There is a formula for calculating the height of the meniscus in a liquid column that accounts for capillary action:
h = 2γcosθ / ρgr
- h is the height of the meniscus in the column of liquid
- γ is the surface tension in the liquid-air environment
- θ is the angle of contact between the liquid and the column wall
- ρ is the liquid density
- g is the acceleration due to gravity
- r is the tube internal radius
Using this formula, note the effect of the radius of the tube. The thinner the tube, the smaller the radius, and the further the liquid travels from capillary action.
Formula for Volume of Liquid Transport
A dry porous medium, such as a paper towel, absorbs liquid at a rate that slows over time. There is a formula for calculating the volume absorbed over time:
- V is the volume of liquid
- S is the sorptivity or the medium’s capacity for absorption via capillary action
- A is the cross-section of the wet area
The rate of liquid absorption depends on several factors, including temperature, permeability, and humidity.
- Batchelor, G.K. (1967). An Introduction to Fluid Dynamics . Cambridge University Press. ISBN 0-521-66396-2.
- de Gennes, Pierre-Gilles; Brochard-Wyart, Françoise; Quéré, David (2004). Capillarity and Wetting Phenomena . Springer New York. ISBN 978-1-4419-1833-8. doi: 10.1007/978-0-387-21656-0
- Freeman, Scott (2014). Biological Sciences . United States of America: Pearson. ISBN 978-0-321-74367-1.
- Liu, M.; et al. (2016). “Evaporation limited radial capillary penetration in porous media”. Langmuir . 32 (38): 9899–9904. doi: 10.1021/acs.langmuir.6b02404
Related Posts

- Why Does Water Expand When It Freezes
- Gold Foil Experiment
- Faraday Cage
- Oil Drop Experiment
- Magnetic Monopole
- Why Do Fireflies Light Up
- Types of Blood Cells With Their Structure, and Functions
- The Main Parts of a Plant With Their Functions
- Parts of a Flower With Their Structure and Functions
- Parts of a Leaf With Their Structure and Functions
- Why Does Ice Float on Water
- Why Does Oil Float on Water
- How Do Clouds Form
- What Causes Lightning
- How are Diamonds Made
- Types of Meteorites
- Types of Volcanoes
- Types of Rocks
Capillary Action
Capillary action is a phenomenon in which liquid flows through a narrow tube without the assistance of any force. It refers to the ascension of liquid through a capillary tube due to forces of adhesion and cohesion and, typically, against gravity. Capillary action occurs when the force of adhesion is greater than the force of cohesion.
For instance, capillary action is observed when placing a thin straw into a cup of water. Water goes into the straw and rises higher than the surrounding water in the cup. The water inside the straw works against gravity, which would questionably pull it down.
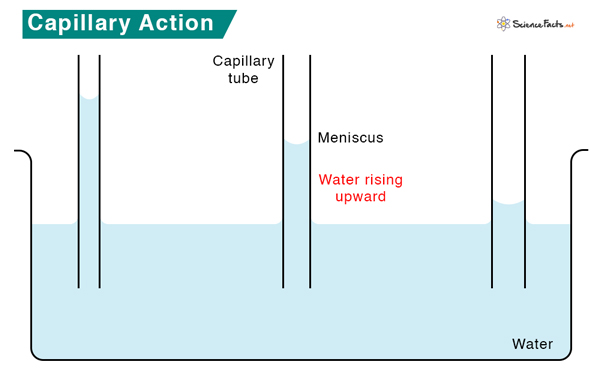
How Does Capillary Action Work
The two properties that make capillary action possible are:
Cohesive Force – Force due to intermolecular attractions between molecules of the same kind, that is, liquid molecules. Cohesive force leads to surface tension in which the surface molecules bond and form an impenetrable layer.
Adhesive Force – Force due to intermolecular attractions between molecules of different kinds, that is, between the liquid and the material of the capillary tube.
The combination of cohesive and adhesive forces causes capillary action. However, it does not occur in all liquids. For instance, mercury will not rise in a narrow tube because its cohesive force is stronger than the adhesive force. At 20 o C, mercury’s surface tension is 486 dyne/cm, much higher than water’s (72.8 dyne/cm).
Capillary action is observed in real life through various examples.
- Plaster and paper towels uptake water since both are porous materials with a strong affinity toward water.
- When a candle burns, the heat from the flame melts the wax. The melted wax traverses up the wick, supplying the fuel necessary to sustain the burning.
- A phenomenon known as thin-layer chromatography separates dyes used in the food industry using capillary action.
- Capillary action occurs naturally in our eyes. It pumps out the tear fluid from our eyes and removes dirt and dust particles.
Capillary Action of Water
Capillary action is a property of water. Water molecules are both cohesive and adhesive because of their polarity. This cohesive property makes water molecules bond firmly, producing high surface tension. The adhesive property allows the molecules to adhere to the walls of the tube, typically made of glass. Adhesion of water to glass causes an upward force on it at the edge, raising the water level in the tube and resulting in an upward meniscus, as shown in the image above.
Capillary Action in Plants
In biology, plants use capillary action to bring water from the soil and distribute it to their various parts. Water contains essential nutrients that are responsible for plants’ growth. As we have seen that capillary action occurs in a capillary tube, plants have narrow tubes called xylem tissue.
As they exit the root system, the water molecules stick together and to the side walls of the xylem tissue. This process is known as transpiration pull. Transpiration is responsible for tall towering trees to supply water to the top leaves from the roots through stems and branches.
Plants extract water exclusively from their roots and transport it to its entirety. Without capillary action, they will not be able to survive.
Height of Liquid Column
A liquid rises inside a thin cylindrical tube due to capillary action. It is possible to determine the height, h, of the liquid column. The following formula gives the height to which the liquid rises.
\( \gamma \) is the surface tension of the liquid-air interface
θ is the contact angle
ρ is the density of the liquid
g is the acceleration due to gravity
r is the radius of the tube in which the liquid is confined
We can use the values at 20 0 C and reduce the above equation. For water confined to a glass tube of radius r, we have
ρ = 998 kg/m 3
g = 9.8 m/s 2
Plugging in these values, we get
Therefore, the tube needs to be sufficiently small in diameter for capillary action to occur.
- Capillary Action and Water – Usgs.gov
- Capillary Action – Chem.libretexts.org
- What is Capillary Action? – Study.com
- Cohesion and Adhesion in Liquids: Surface Tension and Capillary Action – Pressbooks.uiowa.edu
- Capillary Action – Chemdemos.uoregon.edu
- Capillary Action – What It Is and How It Works – Sciencenotes.org
Article was last reviewed on Friday, July 28, 2023
Related articles

Leave a Reply Cancel reply
Your email address will not be published. Required fields are marked *
Save my name, email, and website in this browser for the next time I comment.
Popular Articles

Join our Newsletter
Fill your E-mail Address
Related Worksheets
- Privacy Policy
© 2024 ( Science Facts ). All rights reserved. Reproduction in whole or in part without permission is prohibited.
Capillary Action: Definition and Examples
A spontaneous flow of liquid that doesn't require gravity to work
- Chemical Laws
- Periodic Table
- Projects & Experiments
- Scientific Method
- Biochemistry
- Physical Chemistry
- Medical Chemistry
- Chemistry In Everyday Life
- Famous Chemists
- Activities for Kids
- Abbreviations & Acronyms
- Weather & Climate
- Ph.D., Biomedical Sciences, University of Tennessee at Knoxville
- B.A., Physics and Mathematics, Hastings College
Capillary action is defined as the spontaneous flow of a liquid into a narrow tube or porous material. This movement does not require the force of gravity to occur. In fact, it often acts in opposition to gravity. Capillary action is sometimes called capillary motion, capillarity, or wicking.
Capillary action is caused by the combination of cohesive forces of the liquid and the adhesive forces between the liquid and tube material. Cohesion and adhesion are two types of intermolecular forces . These forces pull the liquid into the tube. In order for wicking to occur, a tube needs to be sufficiently small in diameter.
Examples of capillary action include the uptake of water in paper and plaster (two porous materials), the wicking of paint between the hairs of a paintbrush, and the movement of water through sand.
Fast Facts: History of Capillary Action Study
- Capillary action was first recorded by Leonardo da Vinci .
- Robert Boyle performed experiments on capillary action in 1660, noting a partial vacuum had no effect on the height a liquid could obtain via wicking.
- A mathematical model of the phenomenon was presented by Thomas Young and Pierre-Simon Laplace in 1805.
- Albert Einstein 's first scientific paper in 1900 was written on the subject of capillarity.
See Capillary Action Yourself
An excellent and easy demonstration of capillary action is done by placing a celery stalk in water. Color the water with food coloring and observe the progress of the dye up the celery stalk.
The same process may be used to color white carnations . Trim the bottom of a carnation stem to make sure it can absorb water. Place the flower in dyed water. The color will migrate via capillary action all the way to the flower petals.
A less dramatic but more familiar example of capillary action is the wicking behavior of a paper towel used to wipe up a spill.
- Cohesion Definition in Chemistry
- Surface Tension - Definition and Experiments
- How to Make Colored Flowers
- Surface Tension Definition and Causes
- Decantation Definition in Chemistry
- Water Properties and Facts You Should Know
- How to Make a Rainbow Rose
- Precipitate Definition and Example in Chemistry
- How to Do Chromatography with Candy and Coffee Filters
- Hygroscopic Definition in Chemistry
- Things to Do with Liquid Nitrogen
- Hydrometer Definition in Science
- What Happens to Candle Wax When a Candle Burns
- 4 Simple Chemical Tests for Food
- Centrifugation: What It is and Why It's Used
- Filtration Definition and Processes (Chemistry)
- Utility Menu

Harvard Natural Sciences Lecture Demonstrations
1 Oxford St Cambridge MA 02138 Science Center B-08A (617) 495-5824
- Key to Catalog
enter search criteria into the search box
Capillary action, what it shows:.

How it works:
Setting it up:.
Clamp the tubes to a lab stand and lower them into a petri dish. It is best to mix the ink and water before setting down the tubes. Be generous with the ink (black, blue or red); the setup needs to be illuminated from behind with a light box, and the level in the thinnest tube is especially difficult to see. Use a CCD camera with close-up lens looking as horizontally as possible; the watch glass should sit on a lab jack so a suitable view can be obtained.
As already mentioned, it can be difficult to see the water levels in the capillaries because of the thin optical depth. Play around with the camera angle to get the best contrast on the monitor.
Demo Subjects
Newtonian Mechanics Fluid Mechanics Oscillations and Waves Electricity and Magnetism Light and Optics Quantum Physics and Relativity Thermal Physics Condensed Matter Astronomy and Astrophysics Geophysics Chemical Behavior of Matter Mathematical Topics
Key to Catalog Listings
Size : from small [S] (benchtop) to extra large [XL] (most of the hall) Setup Time : <10 min [t], 10-15 min [t+], >15 min [t++] /span> Rating : from good [★] to wow! [★★★★] or not rated [—]
Complete key to listings

An official website of the United States government
Here's how you know
Official websites use .gov A .gov website belongs to an official government organization in the United States.
Secure .gov websites use HTTPS A lock ( ) or https:// means you’ve safely connected to the .gov website. Share sensitive information only on official, secure websites.
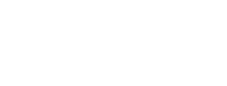
- Digg
Latest Earthquakes | Chat Share Social Media
Capillary Action and Water
- Publications
Plants and trees couldn't thrive without capillary action. Capillary action helps bring water up into the roots. With the help of adhesion and cohesion, water can work its way all the way up to the branches and leaves. Read on to learn more about how this movement of water takes place.
• Water Science School HOME • Water Properties topics •
Capillary Action
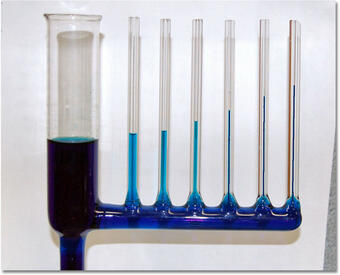
Even if you've never heard of capillary action, it is still important in your life. Capillary action is important for moving water (and all of the things that are dissolved in it) around. It is defined as the movement of water within the spaces of a porous material due to the forces of adhesion, cohesion , and surface tension .
Capillary action occurs because water is sticky, thanks to the forces of cohesion (water molecules like to stay close together) and adhesion (water molecules are attracted and stick to other substances). Adhesion of water to the walls of a vessel will cause an upward force on the liquid at the edges and result in a meniscus which turns upward. The surface tension acts to hold the surface intact. Capillary action occurs when the adhesion to the walls is stronger than the cohesive forces between the liquid molecules. The height to which capillary action will take water in a uniform circular tube (picture to right) is limited by surface tension and, of course, gravity.
Not only does water tend to stick together in a drop, it sticks to glass, cloth, organic tissues, soil, and, luckily, to the fibers in a paper towel. Dip a paper towel into a glass of water and the water will "climb" onto the paper towel. In fact, it will keep going up the towel until the pull of gravity is too much for it to overcome.
Capillary action is all around us every day
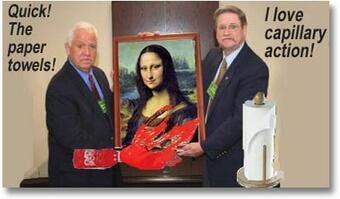
- If you dip a paper towel in water, you will see it "magically" climb up the towel, appearing to ignore gravity. You are seeing capillary action in action, and "climbing up" is about right - the water molecules climb up the towel and drag other water molecules along. (Obviously, Mona Lisa is a big fan of capillary action!)
- Plants and trees couldn't thrive without capillary action. Plants put down roots into the soil which are capable of carrying water from the soil up into the plant. Water, which contains dissolved nutrients, gets inside the roots and starts climbing up the plant tissue. Capillary action helps bring water up into the roots. But capillary action can only "pull" water up a small distance, after which it cannot overcome gravity. To get water up to all the branches and leaves, the forces of adhesion and cohesion go to work in the plant's xylem to move water to the furthest leaf.
- Capillary action is also essential for the drainage of constantly produced tear fluid from the eye. Two tiny-diameter tubes, the lacrimal ducts, are present in the inner corner of the eyelid; these ducts secrete tears into the eye. (Source: Wikipedia )
- Maybe you've used a fountain pen .... or maybe your parents or grandparents did. The ink moves from a reservoir in the body of the pen down to the tip and into the paper (which is composed of tiny paper fibers and air spaces between them), and not just turning into a blob. Of course gravity is responsible for the ink moving "downhill" to the pen tip, but capillary action is needed to keep the ink flowing onto the paper.
The proof is in the pudding ... I mean, in the celery
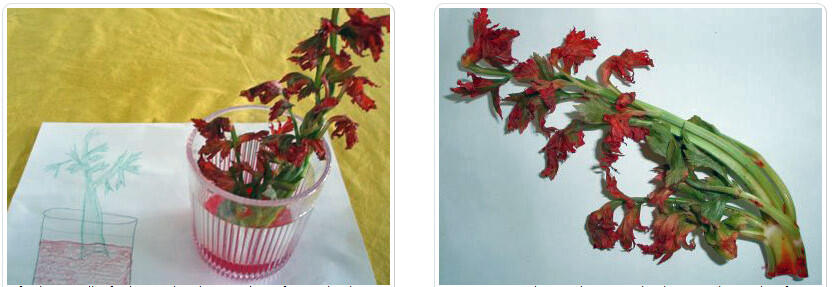
You can see capillary action in action (although slowly) by doing an experiment where you place the bottom of a celery stalk in a glass of water with food coloring and watch for the movement of the color to the top leaves of the celery. You might want to use a piece of celery that has begun to whither, as it is in need of a quick drink. It can take a few days, but, as these pictures show, the colored water is "drawn" upward, against the pull of gravity. This effect happens because, in plants, water molecules move through narrow tubes that are called capillaries (or xylem).
Do you think you know a lot about water properties? Take our interactive water-properties true/false quiz and test your water knowledge.
Sources and more information
- Water Properties: Environment and Climate Change Canada
Learn more about capillary action and related water properties.
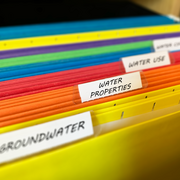
Water Properties Information by Topic
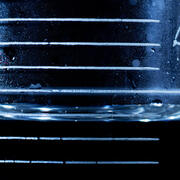
Water Meniscus
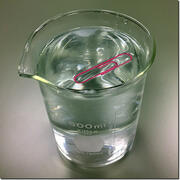
Surface Tension and Water
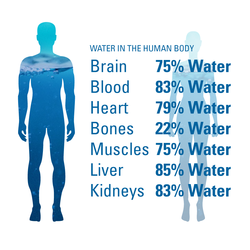
The Water in You: Water and the Human Body
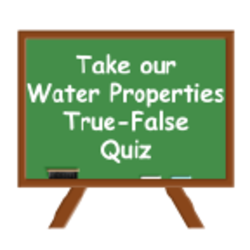
Water Properties True/False Quiz
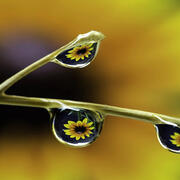
Adhesion and Cohesion of Water
Below are multimedia items associated with capillary action and related water properties.
Below are publications associated with capillary action and related water properties.
Soil water retention and maximum capillary drive from saturation to oven dryness
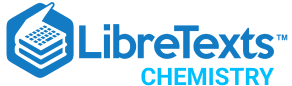
- school Campus Bookshelves
- menu_book Bookshelves
- perm_media Learning Objects
- login Login
- how_to_reg Request Instructor Account
- hub Instructor Commons
Margin Size
- Download Page (PDF)
- Download Full Book (PDF)
- Periodic Table
- Physics Constants
- Scientific Calculator
- Reference & Cite
- Tools expand_more
- Readability
selected template will load here
This action is not available.
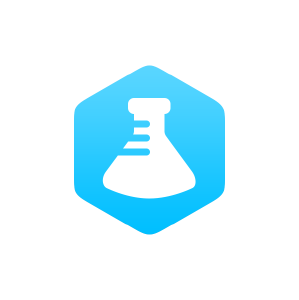
3.4: Capillary Action
- Last updated
- Save as PDF
- Page ID 387404
\( \newcommand{\vecs}[1]{\overset { \scriptstyle \rightharpoonup} {\mathbf{#1}} } \)
\( \newcommand{\vecd}[1]{\overset{-\!-\!\rightharpoonup}{\vphantom{a}\smash {#1}}} \)
\( \newcommand{\id}{\mathrm{id}}\) \( \newcommand{\Span}{\mathrm{span}}\)
( \newcommand{\kernel}{\mathrm{null}\,}\) \( \newcommand{\range}{\mathrm{range}\,}\)
\( \newcommand{\RealPart}{\mathrm{Re}}\) \( \newcommand{\ImaginaryPart}{\mathrm{Im}}\)
\( \newcommand{\Argument}{\mathrm{Arg}}\) \( \newcommand{\norm}[1]{\| #1 \|}\)
\( \newcommand{\inner}[2]{\langle #1, #2 \rangle}\)
\( \newcommand{\Span}{\mathrm{span}}\)
\( \newcommand{\id}{\mathrm{id}}\)
\( \newcommand{\kernel}{\mathrm{null}\,}\)
\( \newcommand{\range}{\mathrm{range}\,}\)
\( \newcommand{\RealPart}{\mathrm{Re}}\)
\( \newcommand{\ImaginaryPart}{\mathrm{Im}}\)
\( \newcommand{\Argument}{\mathrm{Arg}}\)
\( \newcommand{\norm}[1]{\| #1 \|}\)
\( \newcommand{\Span}{\mathrm{span}}\) \( \newcommand{\AA}{\unicode[.8,0]{x212B}}\)
\( \newcommand{\vectorA}[1]{\vec{#1}} % arrow\)
\( \newcommand{\vectorAt}[1]{\vec{\text{#1}}} % arrow\)
\( \newcommand{\vectorB}[1]{\overset { \scriptstyle \rightharpoonup} {\mathbf{#1}} } \)
\( \newcommand{\vectorC}[1]{\textbf{#1}} \)
\( \newcommand{\vectorD}[1]{\overrightarrow{#1}} \)
\( \newcommand{\vectorDt}[1]{\overrightarrow{\text{#1}}} \)
\( \newcommand{\vectE}[1]{\overset{-\!-\!\rightharpoonup}{\vphantom{a}\smash{\mathbf {#1}}}} \)
Capillary action can be defined as the ascension of liquids through slim tube, cylinder or permeable substance due to adhesive and cohesive forces interacting between the liquid and the surface. When intermolecular bonding of a liquid itself is substantially inferior to a substances’ surface it is interacting, capillarity occurs . Also, the diameter of the container as well as the gravitational forces will determine amount of liquid raised. While, water possesses this unique property, a liquid like mercury will not display the same attributes due to the fact that it has higher cohesive force than adhesive force.
Forces in Capillary Action
Three main variables that determine whether a liquid possesses capillary action are:
- Cohesive force : It is the intermolecular bonding of a substance where its mutual attractiveness forces them to maintain a certain shape of the liquid.
- Surface tension : This occurs as a result of like molecules, cohesive forces, banding together to form a somewhat impenetrable surface on the body of water. The surface tension is measured in milliNewton/meter (mN/m).
- Adhesive force : When forces of attraction between unlike molecules occur, it is called adhesive forces.
Capillary action only occurs when the adhesive forces are stronger than the cohesive forces, which invariably becomes surface tension , in the liquid.
.jpg?revision=1&size=bestfit&width=487&height=276)
A good way to remember the difference between adhesive and cohesive forces is that with ad hesive forces you add another set of molecules, the molecules of the surface, for the liquid to bond with. With co hesive forces, the molecules of the liquid will only co operate with their own kind. For water and clean glass, the adhesive forces (water/glass interface) are larger than the cohesive forces in water (hydrogen bonding) and the water wets the glass. For water on dirty glass, the adhesive forces (water/hydrocarbons (covering the glass surface)) can be smaller (depending on how dirty the glass surface is) than the cohesive forces in water resulting in droplets/beads of water on the glass surface. Larger adhesive than cohesive forces results in a rise up the capillary tube.
Applications
Practical use of capillary action is evident in all forms of our daily lives. It makes performing our tasks efficiently and effectively. Some applications of this unique property include:
- The fundamental properties are used to absorb water by using paper towels. The cohesive and adhesive properties draw the fluid into the paper towel. The liquid flows into the paper towel at a certain rate.
- A technique called thin layer chromatography uses capillary action in which a layer of liquid is used to separate mixtures from substances.
- Capillary action helps us naturally by pumping out tear fluid in the eye. This process cleanses the eye and clears all of the dust and particles that are around the ducts of the eye.
- To generate energy: A possible use for capillary action is as a source of renewable energy. By allowing water to climb through capillaries, evaporate once it reaches the top, the condensate and drop back down to the bottom spinning a turbine on its way to create the energy, capillary action can make electricity! Although this idea is still in the works, it goes to show the potential that capillary action holds and how important it is.

When measuring the level of liquid of a test tube or buret, it is imperative to measure at the meniscus line for an accurate reading. It is possible to measure the height (represented by h) of a test tube, buret, or other liquid column using the formula:
\[ h = \dfrac{2\gamma \cos\theta}{\rho\;g\;r} \]
In this formula,
- γ represents the surface tension in a liquid-air environment,
- θ is the angle of contact or the degree of contact,
- ρ is the density of the liquid in the representative column,
- g is the acceleration due to the force of gravity and
- r is the radius of the tube in which the liquid is presented in.
By rearranging the equation and measuring the height of the capillary rise, h, surface tension can be determined.
\[ \gamma = \dfrac{h \rho\;r}{2 cos\theta} \]
- Name one way to increase capillary action, and one way to decrease it.
- If cohesion is greater than adhesion, will the meniscus be convex or concave?
- What would be the height of water in a glass tube with a radius of .6mm? Assume the glass is clean (the contact angle between water and glass is 0°).
- Increase capillary action: Increase temperature, decrease capillary tube diameter, perform any number of actions to decrease surface tension, etc…! Decrease capillary action: The opposite of the steps you would take to increase, also, increasing the density of the liquid you're working with.
- The meniscus will result in a convex formation.
- The height of the water in the glass tube would be ~25mm high.
- Petrucci, Ralph, and William Harwood. F. Geoffrey Herring. Jeffry Madura. General Chemistry: Principles and Modern Applications. 9th ed. Upper Saddle River, NJ: Pearson, 2007.
- Cyclical Gravity Greenhouse Liquid Capillary Action Energy Generator, R Smith, 9.11.2005 14:38,
- Capillary Action, Wikipedia
- Capillary Action, from USGS Water Science for Schools." USGS Georgia Water Science Center - Home Page . Web. 04 June 2011.
Contributors and Attributions
- Achille Peiris, Becky Stein
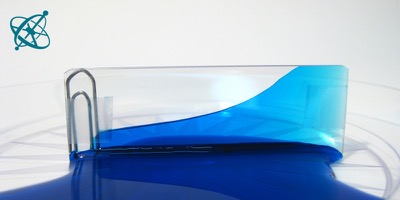
What makes this water rise?
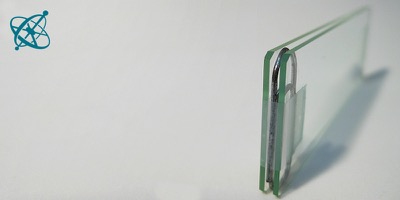
Build a wedge from two microscope slides…
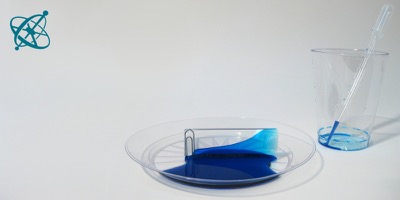
…and place it into the liquid.
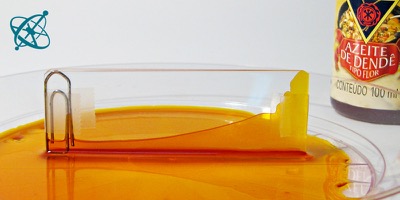
Try different liquids!

Walking Water Rainbow Science Experiment
Let’s make a walking water rainbow! There’s no better way for little scientists to learn about capillary action and color mixing than by making water walk (yes – walk!) in this colorful rainbow science experiment. This science experiment is a favorite of ours because it’s so easy to set up and the results are almost immediate.
Check out the simple step-by-step below and then gra b 30 more jaw-dropping (but easy prep!) science experiments kids will love from our shop!
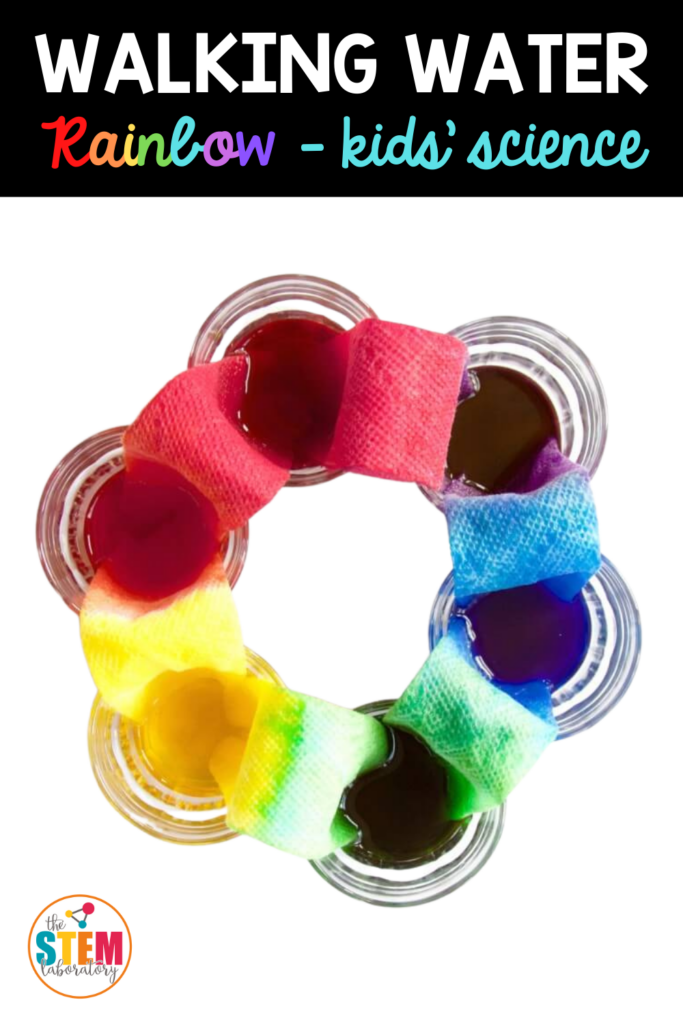
Getting Ready
To prep, I gathered our supplies:
- 6 wide-mouth glasses or jars
- Paper towels (use the kind where you can select a size)
- Food dye or liquid water colors (red, yellow, and blue)
I grabbed the six small glasses first . We’ve had success using wide-mouth drinking cups and canning jars, too. Even though they all worked, just remember that bigger glasses will need more food coloring.
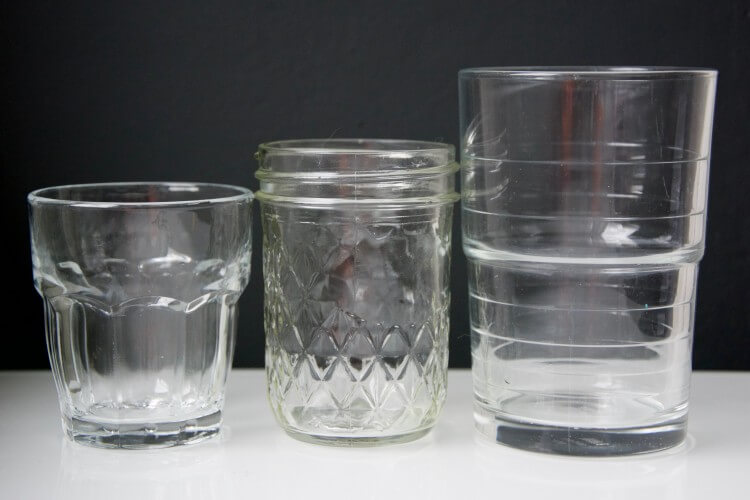
I ripped off six sheets of paper towel and folded each sheet in thirds, lengthwise.
We were using pretty small glasses, so I cut a few inches off the folded paper towel so it would fit in the glasses.
It’s a good idea to test your paper towel strip to make sure they fit properly in your glasses. They should be able to go from the bottom of one jar to the next without sticking up in the air too much. The paper towel on the left shows the just-right height. It’s important to set up this rainbow science experiment for success!

Making a Rainbow
This colorful rainbow science experiment is so simple and quick, it’s perfect for even the youngest little scientists. My 3 year old, Q, couldn’t wait to get started.
First, I had him line up the glasses and fill the first one with a good squirt of red watercolor , the third with yellow, and the fifth glass with blue. We left the other glasses empty.
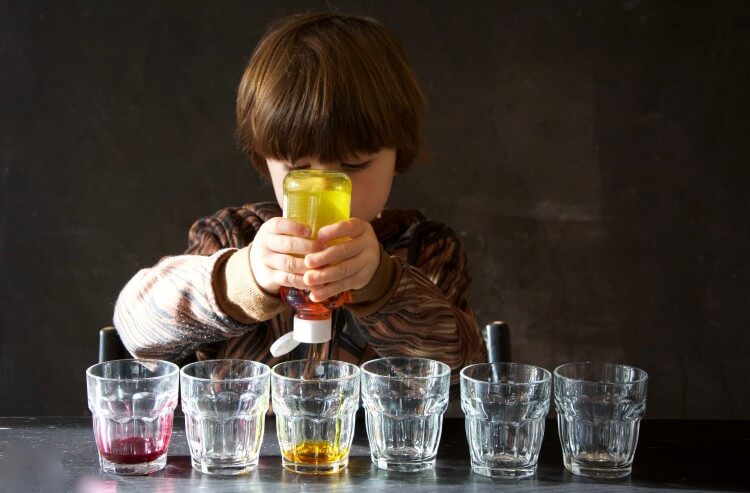
Next, I helped Q add water to the glasses with color until the colored water almost reached the top.
We moved the glasses into a circle and added the paper towels . Starting with the red, we added one end of the paper towel and then put the other end in the empty glass next to it.
We continued around until the last paper towel was placed into the red glass.
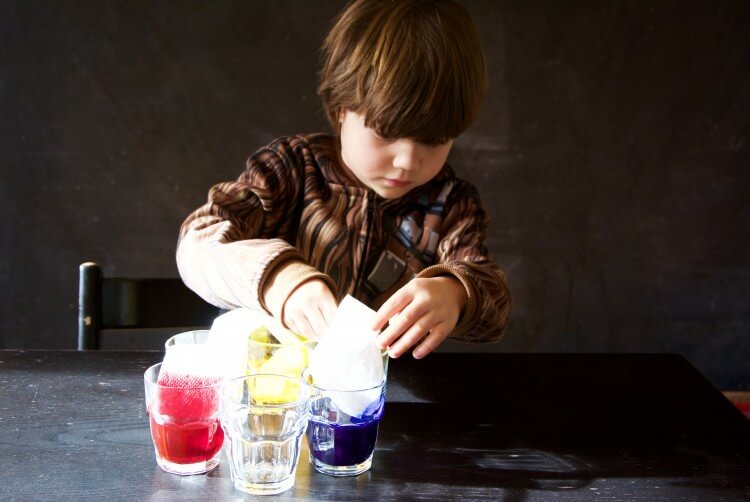
We saw the color wick up the paper towel right away. This rainbow science experiment doesn’t take long to get going!
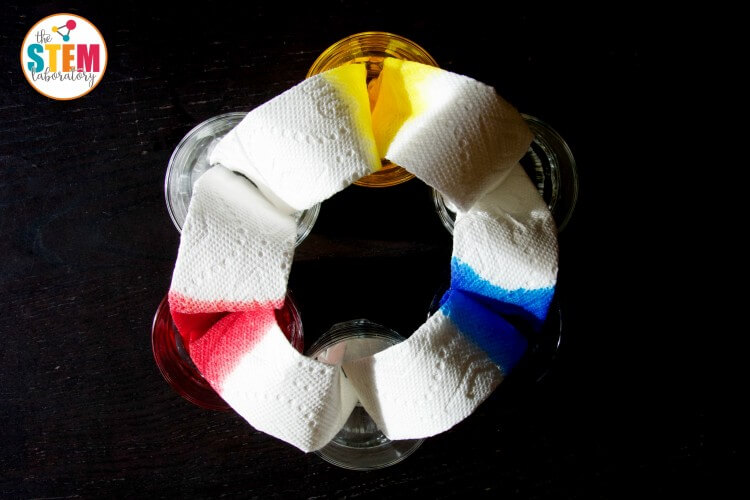
After another several minutes, the colored water had almost travelled the whole length of each paper towel.
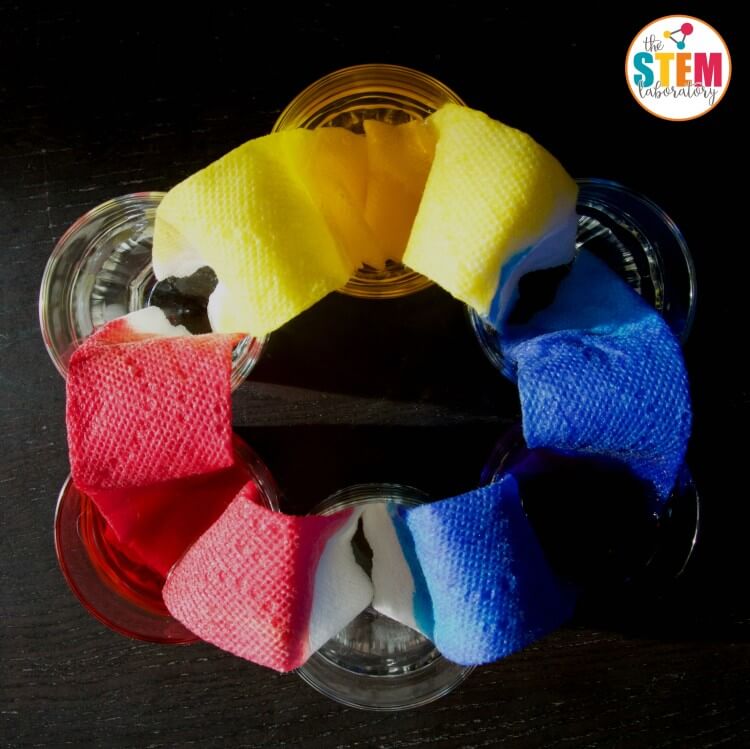
Five minutes later, the water had traveled all the way up and then down the paper towel and was dripping into the empty glass.
The yellow and red water dripped into the empty cup to make orange! It made for a good lesson on color mixing.
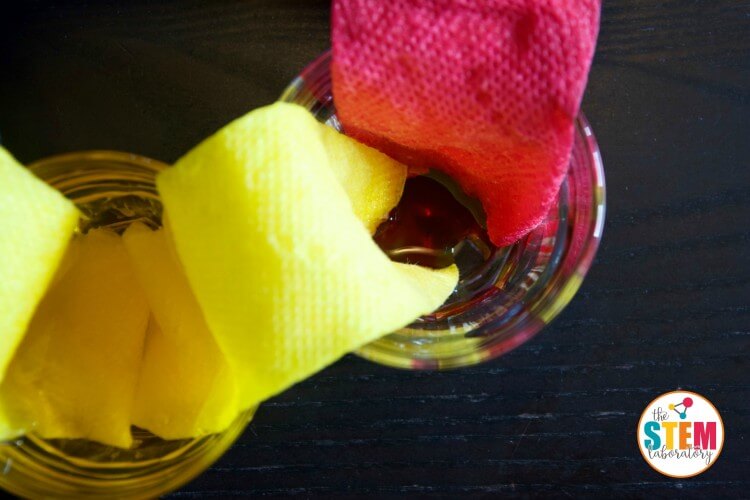
After another five minutes, we could see the water level had dropped in the red, yellow, and blue glasses and rose in the once empty glasses as the water continued to travel from the more full glasses to the less full glasses.
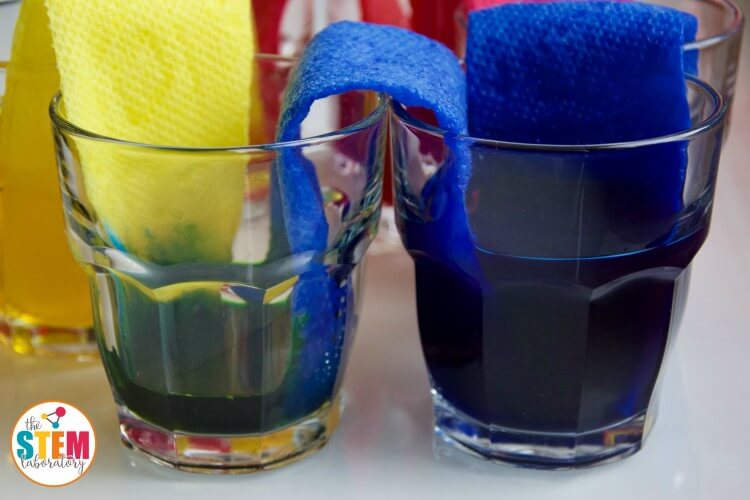
We grabbed a snack and watched our beautiful rainbow science experiment during the next 20 minutes. The water continued to walk from the primary colored glasses to fill the secondary-colored glasses until all the jars were filled equally.

Not Working?
If you aren’t seeing much movement within a few minutes, it may be that you need to add more water to your colored water glasses. It really needs to be almost at the top for the water to walk quickly. So try topping off those glasses and seeing if that gets things moving.
If you see the water moving up the paper towel but it seems like it’s taking forever , it may be the type of paper towel you are using. You want a paper towel that will really hold a lot of water. We have used Bounty Select-a-Size and Target’s Up and Up Brand Select-a-Size with success.
It really is worth the extra effort of trying different cups and paper towels to get this activity to work. And once you have had success, don’t throw out those beautifully-colored paper towels or the colored water! We gently squeezed out our paper towels and let them dry in a heap on a baking sheet. We ended up with gorgeous tie-dyed looking paper towels to use for crafts and we used the leftover water as watercolors for painting with later.
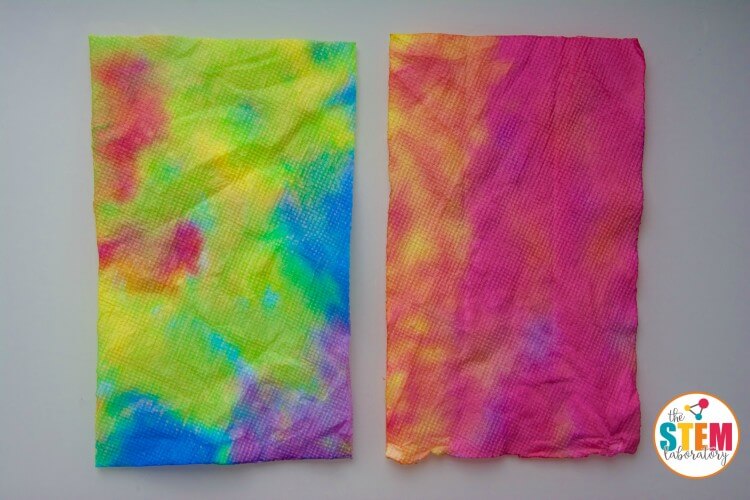
The Science Behind It
This rainbow science experiment is as magic as the science behind it. The colored water travels up the paper towel by a process called capillary action . Capillary action is the ability of a liquid to flow upward, against gravity, in narrow spaces. This is the same thing that helps water climb from a plant’s roots to the leaves in the tree tops.
Paper towels, and all paper products, are made from fibers found in plants called cellulose . In this demonstration, the water flowed upwards through the tiny gaps between the cellulose fibers. The gaps in the towel acted like capillary tubes, pulling the water upwards.
The water is able to defy gravity as it travels upward due to the attractive forces between the water and the cellulose fibers.
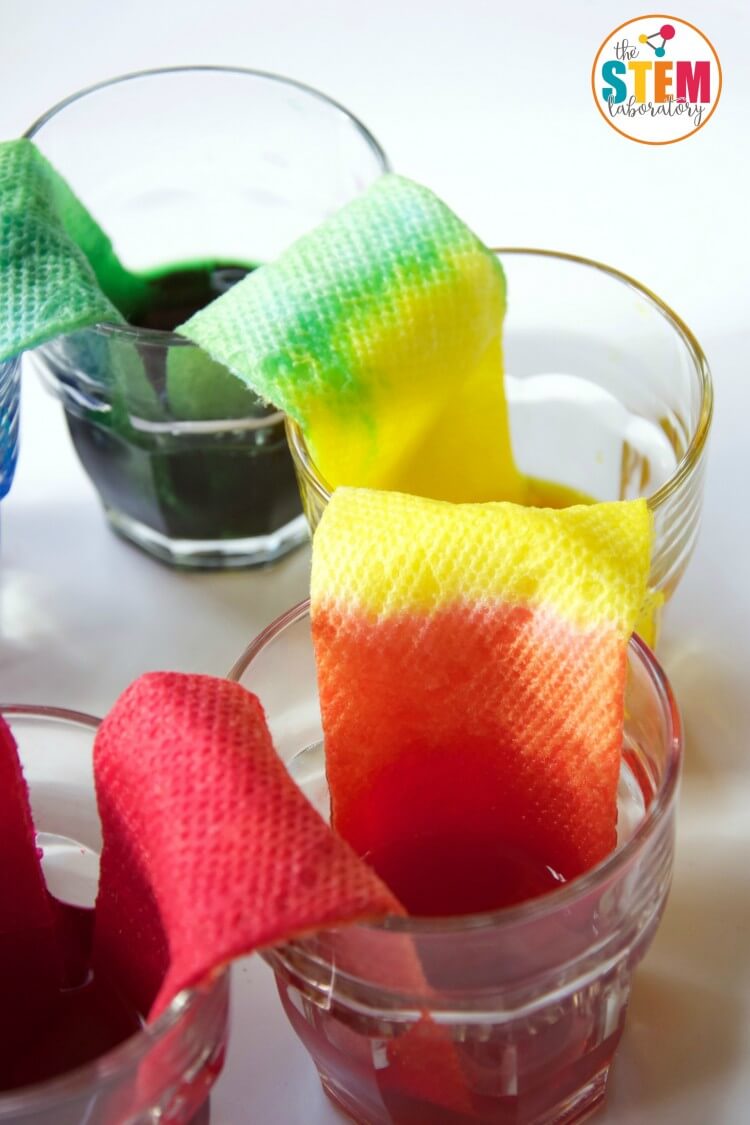
The water molecules tend to cling to the cellulose fibers in the paper towel. This is called adhesion .
The water molecules are also attracted to each other and stick close together, a process called cohesion . So, as the water slowly moves up the tiny gaps in the paper towel fibers, the cohesive forces help to draw more water upwards.
At some point, the adhesive forces between the water and cellulose and the cohesive forces between the water molecules will be overcome by the gravitational forces on the weight of the water in the paper towel.
When that happens, the water will not travel up the paper towel anymore. That is why it helps to shorten the length that colored water has to travel by making sure your paper towel isn’t too tall and making sure you fill your colored liquid to the top of the glass.
Rainbow Science Activity Extensions
Turn this demonstration into a true experiment by varying the water level (volume) you start with and seeing how long it takes the water to reach the empty glass.
Or start with the same volume of colored water and change the brand, type (single vs double ply, quilted vs not) or length of paper towel to see how long it takes for the water to “walk” to the empty glass.
You could even use the same volume of water, same length and brand of paper towel but vary the height of the filled glass , by raising them up on books, to see how that affects the speed of the water as it “walks” to the empty glass.
Have you had enough fun with the paper towels? Try using other paper products to see how the type of paper effects the results. Try toilet paper, printer paper, newspaper or a page from a glossy magazine. What do you predict will happen?
Grab a Record Sheet
Help kids keep track of their results by grabbing our free record sheet! Then grab 30 more jaw-dropping (but easy prep!) science experiments kids will love from our shop!
Similar Posts
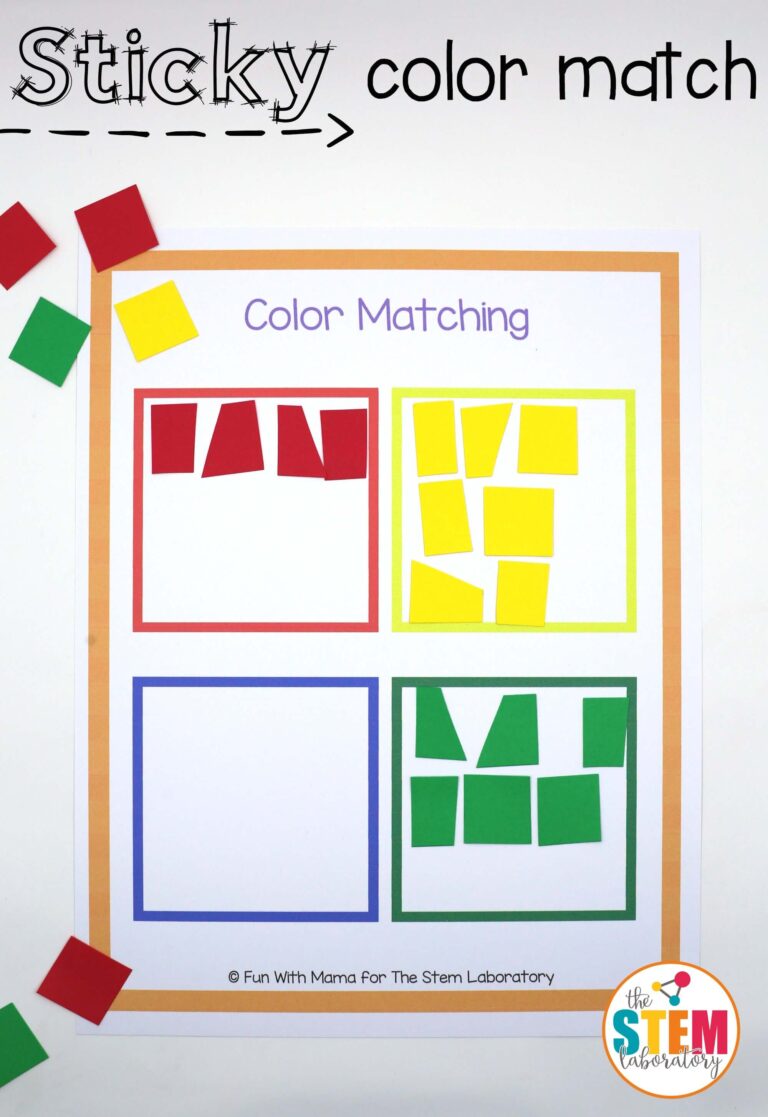
Sticky Color Matching
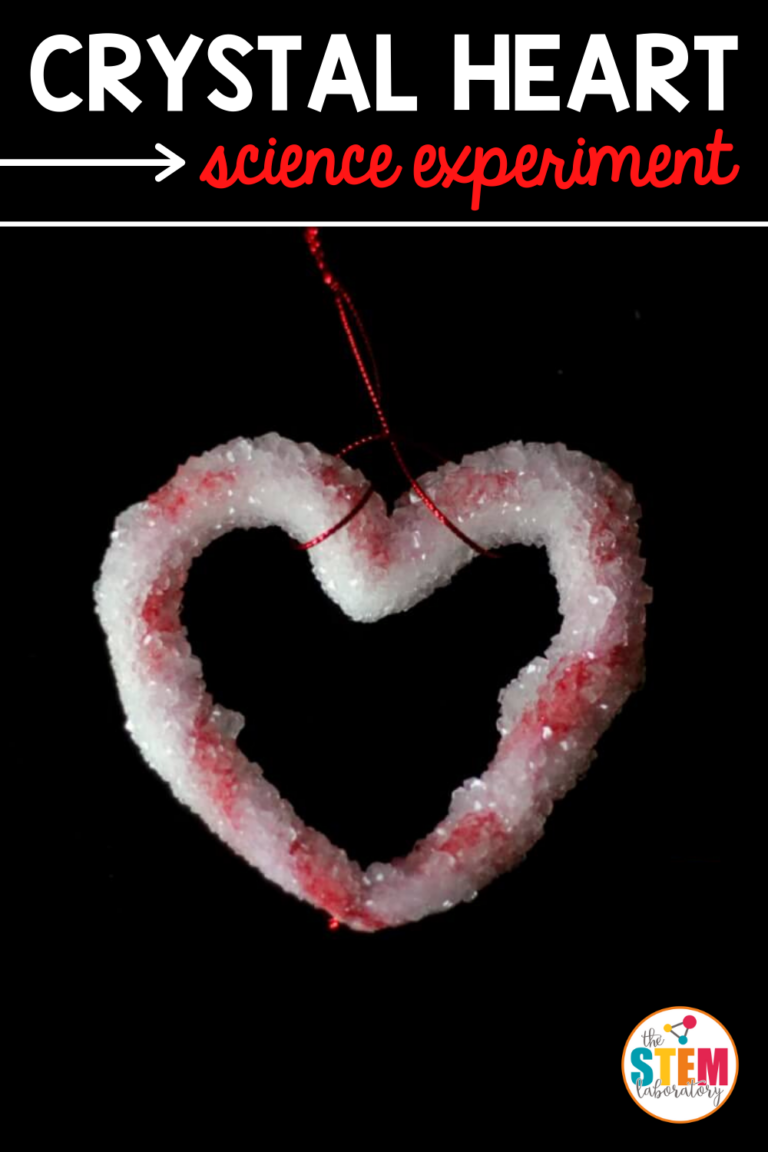
Crystal Heart Valentine’s Science Experiment
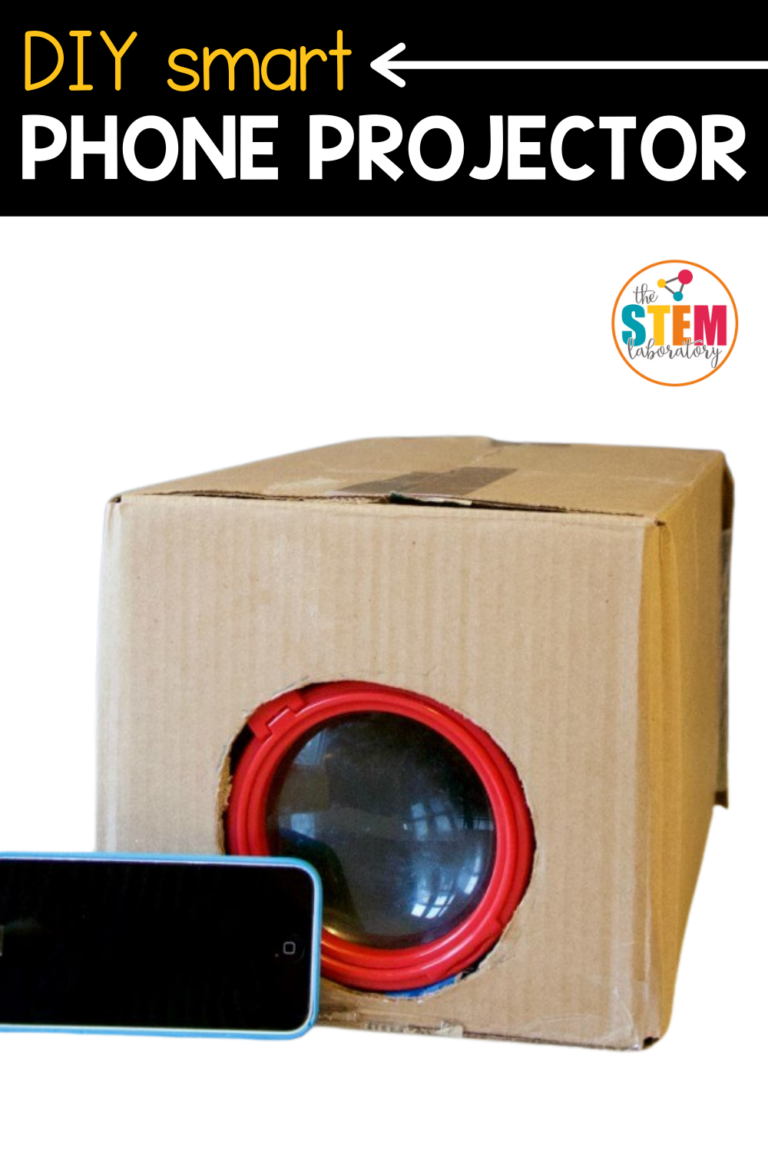
DIY Smart Phone Projector

Kindergarten STEM Activities
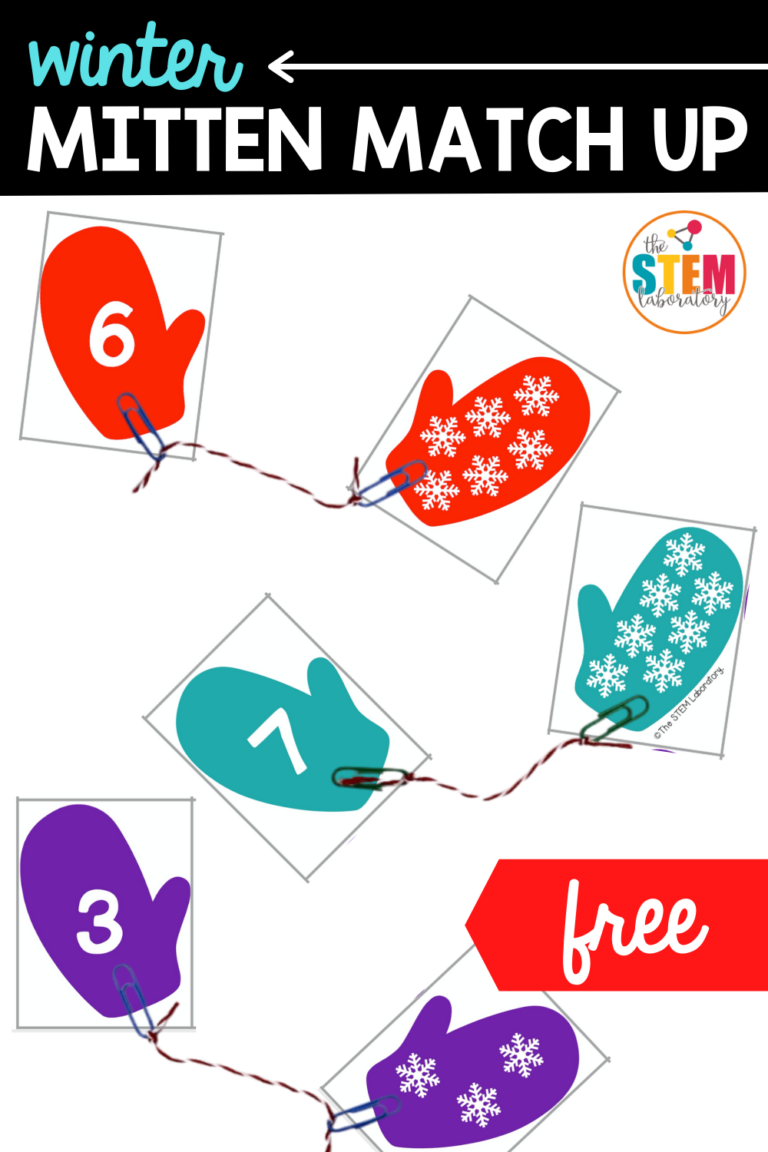

Winter Mitten Number Match Up
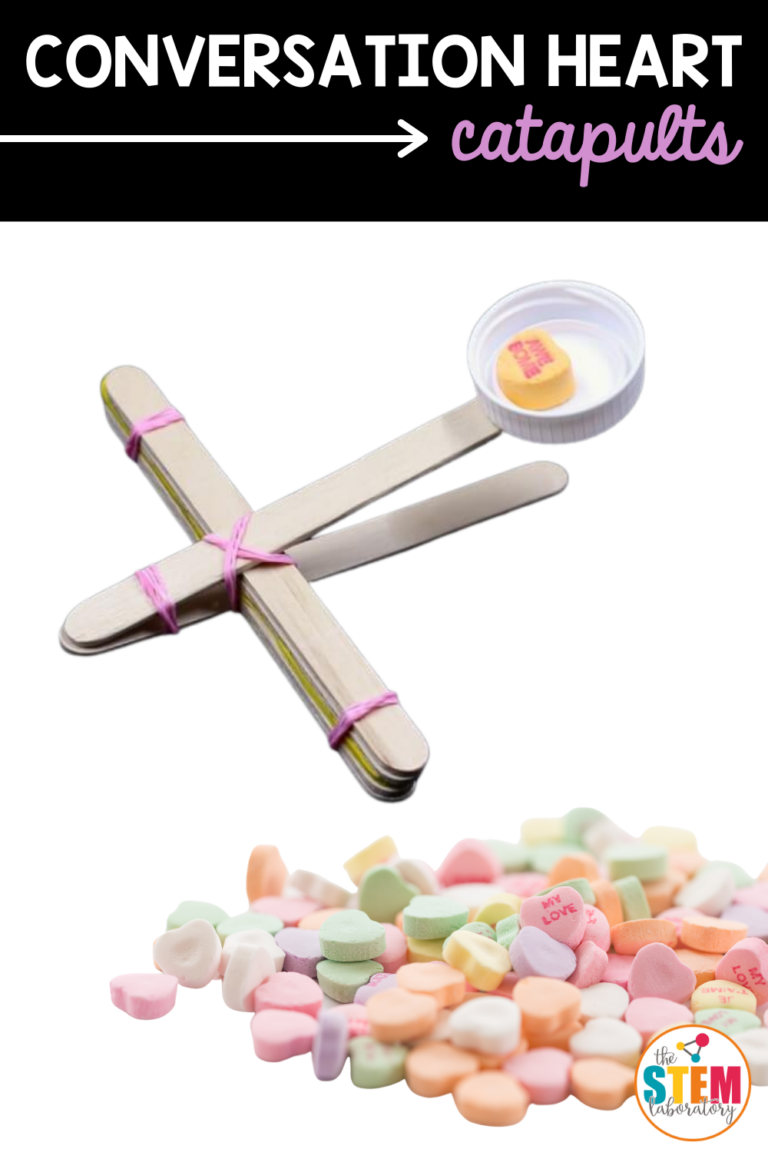
Conversation Heart Popsicle Stick Catapult
11 comments.
- Pingback: Ice Color Mixing - The Stem Laboratory
- Pingback: Color Matching Fish - Teach Me Mommy
- Pingback: EXPLODING PAINT ROCKETS STEAM ACTIVITY
- Pingback: FREE Rainbow Train Preschool Counting Game - Stay At Home Educator
- Pingback: Coloring Sheets - Playdough To Plato
- Pingback: Matching Colors and Numbers 1-6 | Liz's Early Learning Spot
- Pingback: Color Hunt Around the Room - Mrs. Jones' Creation Station
- Pingback: Colors Sorting Mats Game
- Pingback: Ultimate Boredom Buster: 101 Things To Do When Kids Are Bored
- Pingback: 15 Color Activities | Happy Days in First Grade
- Pingback: 5 Easy Science Experiments for Kids [With Video] – Baba Blast!
Leave a Reply Cancel reply
Your email address will not be published. Required fields are marked *
Capillary Forces in Microassembly
Modeling, Simulation, Experiments, and Case Study
- © 2007
- Pierre Lambert 0
Université Libre de Bruxelles (ULB), Bruxelles, Belgium
You can also search for this author in PubMed Google Scholar
- Covers an interdisciplinary field, between the surface sciences fundamental aspects and more production and assembly oriented issues
- The developed methodology includes an exhaustive literature review, models and simulations, experimental validation of the models and of the proposed gripping principle, within the framework of a watch bearing assembly case study
- Provides an exhaustive basis to understand, model (analytically and numerically), and design grippers based on capillary forces
- Includes supplementary material: sn.pub/extras
Part of the book series: Microtechnology and MEMS (MEMS)
36k Accesses
65 Citations
This is a preview of subscription content, log in via an institution to check access.
Access this book
- Available as PDF
- Read on any device
- Instant download
- Own it forever
- Compact, lightweight edition
- Dispatched in 3 to 5 business days
- Free shipping worldwide - see info
- Durable hardcover edition
Tax calculation will be finalised at checkout
Other ways to access
Licence this eBook for your library
Institutional subscriptions
Table of contents (26 chapters)
Front matter, microassembly specificities, from conventional assembly to microassembly, classification of forces acting in the microworld, handling principles for microassembly, conclusions, modeling and simulation of capillary forces, introduction, first set of parameters, state of the art on the capillary force models at equilibrium, static simulation at constant volume of liquid, comparisons between the capillary force models, example 1: application to the modeling of a microgripper for watch bearings, second set of parameters, limits of the static simulation, approaching contact distance, rupture criteria, and volume repartition after separation, example 2: numerical implementation of the proposed models, conclusions of the theoretical study of capillary forces, experimental aspects.
- Surface science
- capillary forces
- microassembly
- micromanipulation case study
- surface tension
About this book
From the reviews:
Authors and Affiliations
Pierre Lambert
Bibliographic Information
Book Title : Capillary Forces in Microassembly
Book Subtitle : Modeling, Simulation, Experiments, and Case Study
Authors : Pierre Lambert
Series Title : Microtechnology and MEMS
DOI : https://doi.org/10.1007/978-0-387-71089-1
Publisher : Springer New York, NY
eBook Packages : Chemistry and Materials Science , Chemistry and Material Science (R0)
Copyright Information : Springer-Verlag US 2007
Hardcover ISBN : 978-0-387-71088-4 Published: 02 October 2007
Softcover ISBN : 978-1-4419-4382-8 Published: 24 November 2010
eBook ISBN : 978-0-387-71089-1 Published: 29 August 2007
Series ISSN : 1615-8326
Series E-ISSN : 2365-0680
Edition Number : 1
Number of Pages : XXII, 263
Topics : Surfaces and Interfaces, Thin Films , Theoretical and Applied Mechanics , Classical and Continuum Physics , Engineering Fluid Dynamics , Nanotechnology , Manufacturing, Machines, Tools, Processes
- Publish with us
Policies and ethics
- Find a journal
- Track your research
Open Access is an initiative that aims to make scientific research freely available to all. To date our community has made over 100 million downloads. It’s based on principles of collaboration, unobstructed discovery, and, most importantly, scientific progression. As PhD students, we found it difficult to access the research we needed, so we decided to create a new Open Access publisher that levels the playing field for scientists across the world. How? By making research easy to access, and puts the academic needs of the researchers before the business interests of publishers.
We are a community of more than 103,000 authors and editors from 3,291 institutions spanning 160 countries, including Nobel Prize winners and some of the world’s most-cited researchers. Publishing on IntechOpen allows authors to earn citations and find new collaborators, meaning more people see your work not only from your own field of study, but from other related fields too.
Brief introduction to this section that descibes Open Access especially from an IntechOpen perspective
Want to get in touch? Contact our London head office or media team here
Our team is growing all the time, so we’re always on the lookout for smart people who want to help us reshape the world of scientific publishing.
Home > Books > Preparation of Space Experiments
Capillary Driven Flows under Microgravity Conditions: From Parabolic Flights to Space Experiment
Submitted: 09 April 2020 Published: 02 September 2020
DOI: 10.5772/intechopen.93467
Cite this chapter
There are two ways to cite this chapter:
From the Edited Volume
Preparation of Space Experiments
Edited by Vladimir Pletser
To purchase hard copies of this book, please contact the representative in India: CBS Publishers & Distributors Pvt. Ltd. www.cbspd.com | [email protected]
Chapter metrics overview
670 Chapter Downloads
Impact of this chapter
Total Chapter Downloads on intechopen.com

Total Chapter Views on intechopen.com
The chapter investigates imbibition into a porous medium under microgravity condition under the action of capillary forces. The study of capillary effects in terrestrial conditions is often difficult due to the influence of gravity, which makes it necessary to conduct experiments in microgravity. The chapter describes the features of experiments and the processing of experimental data in two types of microgravity experiments: during parabolic flights and at space stations in terrestrial orbit. During parabolic flights, a highly permeable artificial porous medium consisting of glass balls of the same size was considered (such a medium makes it easy to visualize experimental results and simulate media with different permeability using balls of different diameters). In experiments in orbit, the flow of fluids with various physical properties in natural sands was considered. The chapter also describes the mathematical modeling of such processes and presents the results of numerical simulations and their comparison with experimental data. The possibility of determining rheological relationships for capillary forces being functions of governing parameters in porous medium on the basis of data obtained from experiments in microgravity is demonstrated.
- microgravity
- parabolic flights
- porous medium
- capillary effects
Author Information
Nikolay smirnov.
- Moscow M.V. Lomonosov State University, Russia
- Scientific Research Institute for System Analysis of the Russian Academy of Sciences, Russia
Valeriy Nikitin
Evgeniya kolenkina (skryleva) *.
*Address all correspondence to: [email protected]
1. Introduction
Investigation of capillary driven seepage under microgravity conditions is of great interest for space applications and terrestrial engineering and technology. On space platforms, capillary transport of liquids is observed in various devices/processes such as purification filtration systems, heat pipes, and fuel transport from tanks in weightlessness. Capillary forces turn to be the major mechanism driving the feeding fluid to the plant’s roots in hydroponics plant growth systems in space.
On the other hand, microgravity investigation of seepage processes gives a deeper insight in the fundamentals of this nonequilibrium phenomenon, thus providing a better understanding of seepage processes for terrestrial applications, such as oil recovery. Microgravity conditions allow us to study capillary effects in large pores, which are impossible in terrestrial conditions due to the influence of gravity, which distorts the shape of the interface and changes effects.
Under terrestrial conditions, the capillary forces are partly shielded by gravity effects, but, nevertheless, are still present. Accurate investigation of wettability effects in immiscible fluids filtration requires abandoning all other effects as far as possible. Determination of some rheological relationships for accounting the influence of capillary forces in porous media is possible by performing experiments under reduced gravity conditions. Such relationships can then be introduced into already existing models, integrating heat and mass transfer through porous media.
The process of imbibition of viscous fluids into a porous medium depends essentially on capillary effects and instability, which may develop on the displacement front in case of multiphase flow. Accounting for capillary forces is critical for the description of the motion of liquids in porous media. The study of the capillary effects under ordinary conditions is difficult because of the significant effect of gravity on the seepage process in big pores, wherein visual registration is possible. Therefore, in this chapter, we consider the flow of liquids in a porous medium under microgravity conditions during parabolic flights. The problem of multiphase seepage in porous media is very well developed. There is a lot of different models based on the modified Darcy law [ 1 , 2 , 3 , 4 , 5 , 6 ]. Experimental and theoretical studies of the flow of fluids in porous media and natural sands under microgravity conditions have been carried out, and mathematical models describing these processes have been developed [ 7 , 8 , 9 , 10 , 11 ]. Another important factor, which influences the displacement, is the instability developing at the front. Initially flat interface of the liquids is broken; some “fingers” of the liquid break through, which causes liquid being entrapped inside the porous medium. The unstable liquid displacement is well studied [ 12 , 13 ]. Experiments on the flow of liquids through a porous medium under microgravity conditions under the action of capillary forces are described in [ 9 ]. The motivation for the present study is investigating the flow of fluid in an artificial porous medium containing heterogeneities under the action of capillary forces. Professor Jean-Claude Legros ( Figure 1 ) and his student Eric Istasse were engaged in the determination of capillary characteristics in porous media, and they conducted experiments on imbibition of fluids into porous media during parabolic flights. In this chapter, the results of these experiments are reviewed and compared with the results of numerical simulation. In the case of a porous medium formed by relatively large particles, it becomes important to take into account additional inner terms in the Darcy equation. The corresponding mathematical model is described here. Models without inertia effects, described in detail in [ 2 , 3 , 4 ], describe fairly accurately slow flows in classical low-permeable porous media, but they are not suitable for processes discussed here.

Professor Jean Claude Legros (left) with Professor Nikolay Smirnov (right).
In this chapter, we focus on seepage flows in porous media with inhomogeneity. The presence of heterogeneity brings to the nonuniformity of the seepage flow and the displacement front, which can lead to nonlinear physical results. Such nonlinear physical results are described in detail in [ 14 ], where experiments on the imbibition of liquids into a natural porous medium under microgravity conditions are considered. Results showed that a point located higher along the length of a sample can be reached by a liquid faster than a lower one. In a natural porous medium, we can only make hypothesis about the reasons of such a behavior; while using an artificial porous medium assembled of transparent glass balls, one can visually register the effect of heterogeneity on the capillary driven seepage flow.
2. Experimental investigations imbibition into artificial porous medium
Parabolic flight campaigns were organized (and funded) by the European Space Agency. The parabolic flights are achieved using the French Airbus A300-ZeroG, an aircraft especially transformed to a rather big microgravity laboratory. Detailed description of experimental procedures and techniques can be found in [ 15 ]. Below we provide a shortened version of the description.
Microgravity conditions encountered during the parabolic flight exercise are very special ones, and anybody will certainly understand that they are far from usual ground-based laboratory conditions. The design of a dedicated experimental payload must thus integrate the specific aspects of reduced gravity environment. For example, flight procedures should be simplified as much as possible, rendering in-flight operations easy to perform. Mass and overall dimensions of the experimental setup are also limiting factors that have to be carefully considered during the payload design.
A typical parabolic flight campaign is usually scheduled around three successive flights days, preceded by 10 days of experiment integration and eventual “last minute” modifications. The short period of time between the flight opportunities may impact the intrinsic conception of the experimental devices, e.g., experimental cells, payload maintenance tasks, and so on. Furthermore, the aircraft safety rules are very strict, and any boarding experiment must first pass a detailed control procedure, performed by the safety crewmembers.
All these aspects are part of a weightlessness flight program and have to be kept in mind during the preparation of a flight opportunity.
2.1 Instrumental setup description
We consider porous samples made of glass spheres having various granulometric size distributions. The regular shape of the glass spheres will eliminate potential anisotropy of the porous sample, simplifying thus the investigation of the observed flows. Another advantage is the translucent character of the glass spheres that enables us to visualize in some way internal fluid motions that are usually inaccessible by direct visualization. Finally, choosing spherical constituting solid particles makes it possible to realize a lot of different samples with various well-known intrinsic properties, i.e., porosity, permeability, and mean characteristic pore radius. Overall dimensions of the porous samples (75 × 50 × 200 mm 3 ) have been determined taking into account the available duration of microgravity.
We investigated several fluid systems. The simplified two-phase flow model, presented in the previous section, is experimentally investigated in the case of a water-air system and in the case of a water-alkane system. We choose the iso-octane, also known as 2,2,4-trimethylpentane, for the hydrocarbon, because it is less dense than water, completely immiscible with it. Iso-octane is less wetting the porous matrix as compared with water. This liquid-liquid system investigation enables us to vary the surface tension parameter without modifying the wettability properties of the porous medium. Furthermore, the viscosity of iso-octane is less than the water one. Hence, we limited the appearing of viscous fingering phenomenon that tends to greatly destabilize the moving liquid-liquid interface. The stable shape of the interface is indeed a necessary condition to neglect the dispersion fluxes appearing in our mathematical model.
The boundary conditions mentioned for the mathematical model, namely no hydrostatic pressure difference between bottom and top of the porous sample, were satisfied. We have also seen that it is necessary to avoid as far as possible the capillary effects at the level of the fluid reservoir. This was achieved by saturating completely the reservoir with the wetting fluid. Note that this reservoir must be able to deform when the capillary flow occurred in microgravity, otherwise we would have observed the formation of an air bubble, which, if growing, would have been able to block the entrance of the porous sample for the fluid. Hence, the reservoir consisted of a deformable plastic bag that remained full of liquid during flight conditions.
During the parabola, the transition period between the pull-up phase and the microgravity phase induced undesired effects that greatly influenced the flow through the porous sample. Capillary driven filtration started indeed immediately at the beginning of the transition period. At this particular moment, we did not have clear initial conditions for the subsequent creeping under reduced gravity. Hence, it was very important for the filtration modeling to restrict as much as possible the transient flow occurring during this transition phase. It was preferable to wait for acceptable reduced gravity conditions to start up the flow. The experimental setup incorporated simple technical solutions to control these undesired effects. The hydraulic scheme of the experiment indeed included electromagnetic valves that allowed us to close hermetically the porous medium. Consequently, if a capillary flow was starting during the transition phase, it would be rapidly damped by the air pressure increase, which was resulting from the decrease of the volume accessible for the gaseous phase inside the cell. Once in real microgravity, we simply opened the valves to allow the capillary driven flow to invade the solid matrix.
The effect of temperature on surface tension and viscosity properties of the fluids was not considered in the present study. The aircraft air-conditioning system maintained the cabin temperature around 25°C during flight operations.
2.2 Payload description
The experimental payload has been completely designed and developed by the scientific and technical teams of the Physical Chemistry Department of the Free University of Brussels. It incorporated all the necessary devices to study various capillary driven flows under reduced gravity conditions, and its basic features will be now briefly detailed.
An electrical panel, mandatory to interface the experiment with the aircraft power supplies.
A video system, including black and white video camera and high-quality video recorder.
A hydraulic scheme, i.e., a network of valves and tubes necessary to handle the eventual fluid feeding of the experimental cells.
A temperature and pressure acquisition system was loaded and allowed to work autonomously during the flight. Data were simply downloaded after landing.
A residual g-level data acquisition system, coupled with the video recording system, enabling video incrustation of residual g-level directly through the video signal.
The main rack measured 880 × 650 × 1440 mm 3 (length × width × height) and integrated in fact two experiments, with a total mass of approximately 300 kg.
The experimental rack was designed to investigate two experimental cells simultaneously. This was a very convenient feature that made it possible to directly correlate two porous samples having different properties under the same microgravity conditions. As the flight planning was organized in 6 sets of 5 successive parabolas, we investigated the experimental cells by pair, and we video recorded 5 successive capillary creeping for them. Longer breaks between two successive sets of parabolas were used to perform the cells exchange.
Following this scientific strategy, we were able to study the influence of the preimbibition of the porous sample. Starting from a completely dry porous medium, the capillary creeping occurred during the first parabola, followed directly by a drainage process during the pull-out phase. The amount of liquid being trapped inside the porous matrix was depending on the surface tension properties of the fluid-fluid pair under investigation. We observed experimentally that the seepage «creepability» parameter Ψ [ 7 , 15 ] was significantly influenced by the preimbibition. But, once the porous matrix has been imbibed, following successive imbibition was not modifying essentially its microscopic surface state. Successive capillary seepage during a set of parabolas would invade the porous matrix higher and higher, simply because the fluid encountered less resistance in the already preimbibitted zone and the latter tended to increase during successive capillary driven imbibition. The number of operations that experimenters had to perform during the parabola itself was intentionally small. The parabolic flight maneuver, with its succession of hypergravity and low gravity periods, revealed to be physiologically tiring. In these conditions, the experimental procedures should be as simple as possible to allow the experimenters to anticipate these repetitive gravity variations. For the capillary creeping experiment, the most difficult tasks were the cells exchange, and the fluid management to adjust correctly the initial fluid levels inside for the porous samples. These tasks were executed before the pull-up phases. As we continuously video recorded the experimental cells, the experimenters had only to wait for the optimal reduced gravity level to switch on the electromagnetic valves, thus enabling the flows through the samples.
2.3 Experiment details and results
We concentrate in this section on the peculiarities of seepage flow in a media containing permeability inhomogeneity. The zones of different permeability were arranged in the experimental cells ( Figure 2 ) using glass balls of different diameters. Porous samples were hermetically confined inside the experimental cell, whose overall dimensions were 74 × 50 × 315 mm 3 . Each cell had a separate fluid reservoir made of a deformable plastic bag. The reservoir fluid capacity was sufficient to fill in totally the porous sample. The experimental cell was connected at its bottom side to a cylindrical connector. This connector was 70 mm long and served as a hydraulic junction between the porous sample and the reservoir, filled in with a water-dye mixture. The diameter of the channel zone has been chosen large enough, i.e., 37 mm, to allow reasonable fluid feeding to the porous medium during microgravity periods. During the flight, the capillary driven filtration was video recorded, using a high-quality video equipment. The back-light system allowed to visualize the phase interface separating water and air.

The experimental cell for artificial porous medium.
Three experiments are reported here. In all cases, we considered the imbibition of fluid into the porous media that has been prewetted throughout previous parabolas. Zones with different permeability were formed by spheres with diameters of 2 and 6 mm. The artificial porous medium within each zone was composed of glass balls of the same diameter. Thus, the permeability of the porous medium consisting of spheres (d = 6 mm) was K 1 = 8.5·10 −9 m 2 , while permeability in the low permeable zone (d = 2 mm) was K 2 = 2·10 −9 m 2 .
In the first experiment, the whole cell was filled with a homogeneous porous medium consisting of glass spheres with a diameter of 6 mm ( Figure 3 ). The graph ( Figure 4 ) shows the position of the interface as a function of time. By reducing the gravity level, the capillary driven imbibition of fluid into porous sample begins. During the observation period, the interface did not exceed the height of 40 mm.

The flow of liquid in a porous medium (permeability: 8.5·10 −9 m 2 ; porosity: 0.458) under microgravity conditions (displacing fluid: viscosity: 1·10 −3 Pa·s, density: 10 3 kg/m 3 ; displaced gas: viscosity: 1.5·10 −5 Pa·s, density: 1.4 kg/m 3 ; surface tension: 0.0582 N/m).

The position of the interface as a function of time for a flow in a homogeneous medium (permeability: 8.5·10 −9 m 2 ; porosity: 0.458; displacing fluid: viscosity: 1·10 −3 Pa·s, density: 10 3 kg/m 3 ; displaced gas viscosity: 1.5·10 −5 Pa·s, density: 1.4 kg/m 3 ; surface tension: 0.0582 N/m).
In the second experiment, the lower layer of the medium (with a height of 4 cm) consisted of spheres with a diameter of 6 mm, and the upper layer consisted of spheres of smaller diameter (2 mm) ( Figure 5 ). The position of the interface is shown in the graph ( Figure 6 ). The process of imbibition is analogous to the case of a homogeneous medium until a zone of low permeability was reached. On crossing the border of high permeable and low permeable zones, the velocity of phase interface drastically increased upon entering the zone of lower permeability small sphere assembly. After a while, the interface velocity again decreased. The acceleration of the imbibition front is explained by the fact that the capillary forces in the medium consisting of small spheres were higher. Successive slowing down of the interface was due to the decrease of permeability. The effects of increasing capillary forces and drag forces acted on different time scales in unsteady-state flows: capillary forces increased by a jump, and then sustained constant value, while drag forces grew linearly on increasing the fluid penetration depth.

The flow of liquid in a porous medium consisting of two layers (bottom layer: height: 4 cm, permeability: 8.5·10 −9 m 2 , porosity: 0.458; upper layer: permeability: 2·10 −9 m 2 ; porosity: 0.466) under microgravity conditions (displacing fluid: viscosity: 1·10 −3 Pa·s, density: 10 3 kg/m 3 ; displaced gas: viscosity: 1.5·10 −5 Pa·s, density: 1.4 kg/m 3 ; surface tension: 0.0582 N/m).

The position of the interface as a function of time for a flow in an inhomogeneous medium (bottom layer: height: 4 cm, permeability: 8.5·10 −9 m 2 , porosity: 0.458; upper layer permeability: 2·10 −9 m 2 , porosity: 0,466; displacing fluid: viscosity: 1·10 −3 Pa·sec, density: 10 3 kg/m 3 ; displaced gas viscosity: 1.5·10 −5 Pa·s, density: 1.4 kg/m 3 ; surface tension: 0.0582 N/m).
In the third experiment, the cell was filled with spheres (d = 6 mm) and included a zone (30 × 20 × 30 mm 3 ) with a lower permeability in the central part of the cell near the left wall, filled with glass beads (d = 2 mm) ( Figure 7 ). The phase interface remained relatively flat in a homogeneous zone. On approaching the zone of lower permeability, the interface becomes curved, and the capillary creeping was faster in the zone of low permeability.

The flow of liquid in a porous medium (permeability: 8.5·10 −9 m 2 ; porosity: 0.458) containing an insert (3 × 2 × 3 cm 3 ) with a low permeability (permeability: 2·10 −9 m 2 ; porosity: 0,466) under microgravity conditions (displacing fluid: viscosity: 1·10 −3 Pa·s, density: 10 3 kg/m 3 ; displaced gas: viscosity: 1.5·10 −5 Pa·s, density: 1.4 kg/m 3 ; surface tension: 0.0582 N/m).
3. Mathematical model and numerical investigation
This section introduces the mathematical and numerical modeling used for the experiments. A more detailed description of can be found in [ 16 ].
3.1 Balance equations
The flow of two incompressible fluids in porous media is considered, without thermal effects taken into account. The imbibition is modeled by the Darcy law, considering the capillary effects at the boundary of the phases. Non-stationarity is taken into account. Permeability and porosity depend on the spatial co-ordinate and on the fluid pressure in the pores. The mathematical formulation of the problem describes the displacement of one fluid by another due to the pressure drop on the sides of the sample or due to a given flow on one side and also due to capillary effects.
The mass balance equations for the phases are as follows:
Here, φ is the porosity, ρ k is the intrinsic density of the k th phase, s k is the phase saturation, t is the time, u k , j is the j th component of the seepage velocity of the k th phase, and x j is the component of the radius vector (point coordinates). The equations are summed up over the repeated index j .
Note that the intrinsic phase velocity v k , j is expressed through the seepage velocity as:
Unlike the seepage velocity of a phase, intrinsic phase velocity is not determined where there is no phase (the seepage velocity at that place is zero).
Both fluids are incompressible. Dividing Eq. ( 1 ) by ρ k yields the reduced form:
The average volume seepage velocity u j is defined as:
Summation of the Eq. ( 3 ) using the definitions ( 4 ) leads to an equation for the total phase seepage velocity:
In the case of porosity not changing with time, the Eq. ( 5 ) reduces to the condition of the solenoidality of the fluid seepage velocity field:
The momentum equations from which the Darcy laws for each phase are derived are as follows:
where μ k is the dynamic viscosity, K 0 is the absolute permeability, p k is the pressure in the phase, and F k is the interaction force with other phases of the fluid. The right-hand side of ( 7 ) corresponds to the sum of the force of interaction with the skeleton or solid phase and the forces of interaction with other phases of the fluid.
To obtain Darcy equations, the inertial terms (i.e., non-stationary term ∂ ∂ t ρ k φ s k v k , j and convective term ∂ ∂ x j ρ k φ s k v k , i v k , j ) are usually neglected in ( 7 ), and the interaction forces with other phases, except the skeleton or solid phase), are modeled by modifying the basic interaction force with the skeleton by introducing relative permeability. We will neglect only the convective component of inertia, leaving the non-stationary component. After this, the Darcy equations are modified, containing the time derivative of the velocity:
We assume the relative permeability of the phase, depending on its saturation s k and, in general, on the saturation of other phases: K k R = θ k ∙ s k , where θ k is a proportionality coefficient. It should be noted that since the relative permeability is zero in the case of zero phase saturation s k , the singularity in this case in ( 8 ) has no place. So the modified Darcy equations can be written as follows:
Denote the parameter, which is in front of the time derivative of the velocity and has the dimension of time, as T k ; in general, it depends on its position in space, as does absolute permeability, but not on the current distribution of variable parameters (saturation, velocity, etc.):
The Darcy equation is then written in the following form:
The parameter T k can be called the characteristic inertia time. In the case of permeability K 0 being sufficiently small, as in ordinary porous media, this time is negligible and inertia can be neglected. But if the porous medium consists of stones/balls with a sufficiently large diameter, then such a time for low-viscosity liquids can be several seconds or more; it will most likely be impossible to neglect inertia.
Locally, the pressure difference between the phases is determined by capillary pressure, which depends only on the phase saturation, besides the skeleton data and physical data of the surface separating the phases. Hereinafter, we assume that there are only two fluid phases in the pore space, so that:
3.2 Modeling relative permeability and capillary pressure
The relative permeabilities K k R are calculated using the Brooks-Cory model [ 17 ]:
Here, k k 0 > 0 and n k 0 > 0 are the model parameters, and the effective saturation S k is determined by the residual saturations of 0 ≤ s k res ≤ 1 ( s 1 res + s 2 res < 1 ). It should be noted that for 0 ≤ S k ≤ 1 , a porous medium is impregnated with both fluids; the phase saturation at s k ≤ s k res is not described with this model. Further, we always assume that impregnation with both fluids takes place, and under these conditions, the zero alternative of ( 13 ) can be disregarded. It can also be noted that S 2 = 1 − S 1 , and thus index 1 is omitted further and at reduced saturation, the effective saturation of the second phase is expressed through the first. For the effective saturation and relative mobility of the phases not based on the model ( 13 ) and definition ( 14 ), we have the following expressions:
Capillary pressure from the k th phase is expressed through the J-function of Leverett [ 1 ] and some other parameters like:
where σ is the coefficient of surface tension, and α k is the angle between the wall from the phase k and the interface (wetting angle). If this angle is less than π / 2 , then the phase is wetting; if not, it is nonwetting.
Suppose the definition ( 15 ) holds for both phases with the same J-function. In this case, ( 12 ) leads to:
where the primes refer to nonwetting phase and, because of the connection between the wetting angles, we get
None of the known models of the Leverett function imply the right side of ( 16 ). Consequently, the expression ( 15 ) should be considered only for one of the phases, but from the side of the other phase, the capillary pressure is determined by ( 12 ). In most of models, a wetting phase k α k < π / 2 is modeled using ( 15 ), while the nonwetting phase k ∕ makes an adjustment to the definition of capillary pressure:
Using both expressions ( 15 ) for the wetting phase and ( 17 ) for the nonwetting, we express the capillary pressure using data from phase 1 (omitting the index 1 of capillary pressure, wetting angle, and saturation):
Let the J-function of Leverett be modeled by two parameters C J > 0 and a J > 0 and effective saturation:
Boundary conditions used:
At the inflow z = 0 , we set the pressure p = p in and maximal saturation s = 1 − s res 2 .
At the outflow, we set a zero pressure and zero saturation normal derivative.
At the impermeable walls, we set to zero the normal components of phase velocities u kn = 0 , and this corresponds to zero normal derivatives of pressure and saturation.
To demonstrate the role of inertial effects in this problem, two calculations are carried out: with and without inertia ( Figure 8 ). It is seen that the dynamics of imbibition on the left and right graphs are significantly different. When inertial effects are taken into account (left side), the graph has first a shape of a quadratic function of time (0–0.02 s), then linear (0.02–0.06 s), and then as a square root (0.06–2 s). When approaching zones with different permeability, these shape sections repeat: quadratic (2–3 s), linear (3–3.9 s), and square root. This result is consistent with the results presented in [ 7 ]. When inertial effects are not taken into account (right), the graph has the form of a monotonously increasing function close to a square root with a small break when passing through the border of zones with different permeability (4 cm).

Positions of the interface as a function of time for a flow in an inhomogeneous medium with inclusion of inertia (left) and excluding inertia (right) (bottom layer: height: 4 cm, permeability: 8.5·10 −8 m 2 , porosity: 0.45; upper layer: permeability: 2·10 −8 m 2 , porosity is 0.45; displacing fluid: viscosity: 1·10 −3 Pa·s, density: 10 3 kg/m 3 ; displaced gas: viscosity: 1.5·10 −5 Pa·s; density: 1.4 kg/m 3 ; surface tension: 0.0582 N/m).
4. Comparison of numerical calculations with experimental data
The microgravity quality was poor for the present experiments that does not permit us to perform quantitative comparison of results with the outcomes of numerical modeling, which were carried out for ideal conditions. Nevertheless, the qualitative results of the calculations are similar to the experiment.
In the case of flow in a homogeneous medium ( Figure 4 ), the displacement is uniform gradually slowing down. In the experiment, the speed falls down more than in calculations. This is because the mathematical model does not take into account the acceleration of gravity g, which is close to zero in the experiment, but not equal to zero.
In the case of an inhomogeneous medium ( Figure 6 ), when crossing the border of zones with different permeability, the graph has a complex space-time dependence form: quadratic (3–4.5 s), linear (4.5–5.5 s), then as square root. The main characteristic feature of the imbibition front passing through the boundary of media is its rapid acceleration (, it moves faster than if the medium were uniform, as in Figure 4 ). This corresponds to the results of the experiment.
The distribution of the saturation of the displacing liquid at different instants for the calculation, when the region contains an insert with a low permeability, is shown in Figure 9 . This calculation corresponds to the experiment shown in Figure 7 . In calculation, as in the experiment, having reached the insertion, the liquid begins to flow through the insert faster than in the zone with a higher permeability. The calculation also shows that the dispersion in a less permeable medium is higher ( Figure 9 ).

The result of numerical modeling of fluid flow in the region containing an insert with a low permeability (media permeability: 8.5·10 −9 m 2 , porosity: 0.458; insert: dimensions: 3 × 2 × 3 cm 3 , permeability: 2·10 −9 m 2 , porosity: 0.466; displacing fluid: viscosity: 1·10 −3 Pa·s, density: 10 3 kg/m 3 ; displaced gas: viscosity: 1.5·10 −5 Pa·s, density: 1.4 kg/m 3 ; surface tension: 0.0582 N/m).
5. Space experiments on capillary driven imbibition
Carrying out experiments on capillary seepage in space allows observing imbibition under conditions of higher quality microgravity and for longer periods. Such experiments were described in detail in [ 9 , 14 ]. Here, we give a brief description of these experiments and show the possibility of determining empirical constants in mathematical models based on experimental data.
5.1 Experiment payload
Experiments on capillary driven seepage of oil in natural porous medium (sand) were performed within the frames of the MIRROR GAS programme. The experiments were prepared and supervised by Dr. D’Arcy Hart, C-CORE (Memorial University of Newfoundland), Drs. Laurier Schramm and Fred Wassmuth, Petroleum Recovery Institute (PRI, Calgary). The MIRROR payload is shown in Figure 10 .

MIRROR payload ready for GAS container integration at Kennedy Space Center. Capillary flow experiment cells are in the right-hand part of the upper section.
Liquid seepage was observed in three sample cylinders with the same porous medium: mixture of 20% (by weight) kaolinite (a sort of clay) and 80% silica sand. The fluids moving in each of the three cells were crude oil, lubricating oil, and polymer in distilled water.
The media and fluids were transported separately into orbit. An experiment cell consisted of a reservoir and a soil cell, with a sliding gate dividing the two (see Figure 11 ). At the beginning of the experiment, the stepper motor retracted the sliding gate, exposing the soil to the reservoir fluid, whereupon the liquid began to leak under the action of capillary forces under microgravity conditions. The level of fluid in the soil was detected using 48 fiber optic sensor probes located along the length of the sample. The results of the experiment are shown in Figure 12 (black asterisk markers).

Experiment cell for space experiments on capillary driven imbibition.

Results of numerical modeling of the imbibition process of a liquid into a porous medium under the influence of capillary forces compared to experiment results.
5.2 Mathematical model for multiphase seepage
We consider the seepage of a liquid through the area of length L. The capillary forces are taken into account. The nonlinear convection-diffusion equation for a porous medium is [ 12 , 13 ].
where s is the saturation of the displacing fluid, φ is the porosity, D is the diffusion coefficient, and F is the convection flux.
The initial conditions for saturation s t x are
The boundary conditions are
where s min and s max are respectively the minimum and maximum possible saturations.
The convection-diffusion equation with initial and boundary conditions describes the displacement of a quantity s propagating as a step along the x -axis from the left to the right, if the convection flux is positive ( F > 0 ) [ 7 , 8 ]. The diffusion coefficient D is always positive; it blurs the front of propagation.
The effective saturation S is
Below, the index “O” corresponds to the displacing fluid (“oil”). The index “G” corresponds to the displaced liquid (“gas”). Dimensionless relative mobilities M O and M G are calculated by the Brooks and Corey model:
where n O , k O , n G , k G are empirical constants. When simulating two-phase seepage, the expression for F is as follows:
where u t is the total seepage velocity.
Formula ( 25 ) was obtained as a result of averaging in modeling integral parameters with the help of 1D Equation [ 12 ].
Additional effect associated with the instability of capillary affected flows is modeled by the application of an auxiliary term to F :
The details of such method for 1D approximation for 3D results are discussed in [ 13 ].
This term is proportional to the empirical coefficient F 0 . It intensifies the dispersion of the displacement front. The sign of F 0 is positive since the instability intensifies the flow.
The total seepage velocity is either specified (flux control)
or calculated using a definite integral (pressure control)
where ∆ P 0 is constant pressure drop.
The diffusion coefficient D is constructed from the sum of the diffusion coefficients due to the capillary forces d C and the dispersion, which simulates, in the one-dimensional approximation, the blurring of the front due to other effects.
Diffusion formed by capillary forces d C looks according to the Leverett model:
where θ is the wettability angle on the side of the displacing liquid, and C J , a J are parameters of the Leverett function. Similar procedure was described in [ 1 ].
The dispersion depends on the total flux of the phase and is proportional to its magnitude. Thus, considering dispersion and capillary effects, the diffusion coefficient D is modeled [ 10 , 11 ] as
We note that the additional factor which is proportional to the coefficient F 0 also enters into the dispersion structure.
Processing the mathematical model described above makes it possible to select the coefficients responsible for the blurring of the front on the basis of experimental data. We have obtained that for the three experiments described above, the optimal coefficients are as follows ( Table 1 ):
Crude oil | |||
Lubricating oil | |||
Polymer in distilled water |
Optimal coefficients for the three experiments.
The pressure drop or the specified flux was absent, so that the motion was carried out only by capillary forces.
The distribution of the saturation of the penetrating liquid in the calculated region over time is shown in Figure 12 . The parameters of each of the three calculations corresponded to the actual experiment. The experimental data are marked with black asterisk markers.
Thus, as can be seen from Figure 12 , the results of numerical calculations based on the proposed mathematical model are in good agreement with the experimental data. The blurring of the displacement front for each of the liquids is different: the smallest blurring is observed in crude oil ( Figure 12(a) ), and the front is most strongly blurred in the case of imbibition of polymer ( Figure 12(c) ). For each fluid, the empirical constants C J , a J , and F 0 are selected. The value of a J for all liquids is chosen to be 0.5. The coefficients C J and F 0 are different for each case, but they are of the same order. The coefficients were chosen in such a way that the blurring of the front in the numerical calculation would have the least difference from the experimental data.
6. Conclusions
Experiments on the imbibition of a fluid into an inhomogeneous artificial porous medium under microgravity conditions during parabolic flights are considered. A mathematical model for multiphase seepage considering inertial effects is developed. The results of the experiment are compared with the results of numerical simulation of the flow of a viscous liquid in a porous medium, taking into account capillary and inertial effects. It is shown that when the transition from a more permeable medium to a less permeable one takes place, the velocity of the imbibition front increases. This is because the capillary forces in a medium with a low permeability are larger. On further imbibition into the low permeable medium, the velocity of imbibition decreases much faster than that in highly permeable medium. It is also shown that the dispersion of fluid during its imbibition into a less permeable medium is much higher. A model describing the displacement front blur due to diffusion, capillary effects, and flux instability was developed; the model uses two empirical constants. This model can describe the displacement front peculiarities even in 1D problem setup. To estimate those empirical constants, the results of experiments in microgravity were used; those conditions were needed to exclude the gravity factor affecting the flux and diminishing the capillary effects.
Acknowledgments
Authors express warmest gratitude and high appreciations of the results by Professor Jean Claude Legros, who was initiator and principle investigator in these researches. The experimental hardware and methodology were developed by Dr. Eric Istasse and became the base of his thesis [ 15 ]. The authors with financial support of Russian Academy of Sciences performed theoretical investigations and numerical simulations.
- 1. Barenblatt GI, Entov VM, Ryzhik VM. Theory of Fluids Flows through Natural Rocks. Dordrecht, Boston, London: Kluwer Academic Publishers; 1990
- 2. Bear J, Bachmat Y. Introduction to Modelling of Transport Phenomena in Porous Media. Dordrecht, Boston, London: Kluwer Academic Publishers; 1990
- 3. Bear J. Dynamics of Fluids in Porous Media. New York: Dover Publications Inc.; 1988
- 4. Kaviany M. Principles of Heat Transfer in Porous Media. 2nd ed. New York: Springer-Verlag; 1995
- 5. Nield DA, Bejan A. Convection in Porous Media. New York/Berlin/Heidelberg/London: Springer-Verlag; 1992
- 6. Nigmatilin RI. Dynamics of Multiphase Media. Moscow Science Publication; 1987
- 7. Smirnov NN, Dushin VR, Legros JC, Istasse E, Boseret N, Mincke JC, et al. Multiphase flows in porous medium—Mathematical model and microgravity experiments. Microgravity Science and Technology. 1996; IX/3 :222-231
- 8. Smirnov NN, Nikitin VF, Norkin AV, Kudryavtseva OV, Legros JC, Istasse E, et al. Capillary driven filtration in porous media. Microgravity Science and Technology. Hanser Publications, Munich, Germany. 1999; XII/1 :23-35
- 9. Smirnov NN, Legros JC, Nikitin VF, Istasse E, Schramm L, Wassmuth F, et al. Filtration in artificial porous media and natural sands under microgravity conditions. Microgravity Science and Technology. Bremen, Germany: Z-Tec Publishing. 2003; XIV/2 :3-28
- 10. Smirnov NN, Nikitin VF, Ivashnyov OE, Maximenko A, Thiercelin M, Vedernikov A, et al. Microgravity investigations of instability and mixing flux in frontal displacement of fluids. Microgravity Science and Technology. 2004; XV/3 :3-28
- 11. Smirnov NN, Dushin V, Nikitin V, Philippov Y. Two phase flows in porous media under microgravity conditions. Microgravity Science and Technology. 2008; 20 (3–4):155-160
- 12. Smirnov NN, Dushin VR, Nikitin VF, Phylippov YG, Nerchenko VA. Three-dimensional convection and unstable displacement of viscous fluids from strongly encumbered space. Acta Astronautica. 2010; 66 :844-863
- 13. Smirnov NN, Nikitin VF, Maximenko A, Thiercelin M, Legros JC. Instability and mixing flux in frontal displacement of viscous fluids from porous media. Physics of Fluids. 2005; 17 :084102
- 14. Dushin VR, Nikitin VF, Smirnov NN, Skryleva EI, Tyurenkova VV. Microgravity investigation of capillary driven imbibition. Microgravity Science and Technology. Springer. 2018; 30 (4):393-398
- 15. Istasse E. Determination of capillary characteristics in porous media, Free University of Brussels, Faculty of Applied Sciences, Dissertation presented by Eric Istasse with a view to obtaining the Diploma of Advanced Studies in Applied Sciences, Director: Prof. Legros J.C., Academic Year 2000-2001, Brussels. 2001
- 16. Smirnov NN, Nikitin VF, Skryleva EI. Microgravity investigation of seepage flows in porous media. Microgravity Science and Technology. Springer. 2019; 31 (5):629-639. DOI: 10.1007/s12217-019-09733-7
- 17. Larry WL. Enhanced Oil Recovery. Englewood Cliffs, New Jersey: Prentice-Hall; 1989
© 2020 The Author(s). Licensee IntechOpen. This chapter is distributed under the terms of the Creative Commons Attribution 3.0 License , which permits unrestricted use, distribution, and reproduction in any medium, provided the original work is properly cited.
Continue reading from the same book
Published: 02 September 2020
By Angelika Diefenbach, Stephan Schneider and Thomas ...
1151 downloads
By Thais Russomano
1054 downloads
By Jean-Louis Thonnard, Laurent Opsomer, Philippe Lef...
1059 downloads
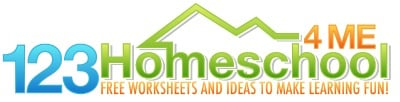
EASY Capillary Action Science Experiment for Kids
- Kids Activities
- Science Experiments
- Kindergarten

Are you tired of ads too?
For a small fee you can get this site – and all the free goodies – 100% ad-free!
Your kids are going to be blown away by this fun, colorful capillary action experiment that teaches a simple science principle . Use this capillary action for kids project with toddler, preschool, pre-k, kindergarten, first grade, 2nd grade, and 3rd grade students. This capillary action science experiment is sometimes called a walking water experiment . No matter what you call it, this beautiful walking rainbow activity is sure to AMAZE kids of all ages!

Capillary action experiment
This capillary action experiment will amaze kids from toddlers, preschoolers, and kindergarteners to grade 1, grade 2, and grade 3 students. You only need a couple simple materials you most likely already ahve tohome: paper towels, water, and food coloring to watch capillary action in most with this beautiful capiarlly action science experiment . Children will very quickly be able to watch as water moves or travels and by doing so it will create a beautiful rainbow, introduce color mixing, and delight young learners as they try to understand what just happened! This capillary experiment will produce a WOW moment for sure and is such an easy science experiment for kids !
Plus don’t miss all our free science lessons , printable 3rd grade worksheets , and super cool newton’s cradle project !
Capillary Action for Kids
Kids are naturally curious about the world around them. They ask lots of questions and perform their own experiments. Just look at a toddler who decides to blow into his milk to see what will happen. It sometimes takes them a lot of trial and error, but eventually they realize it will bubble up over the top of the glass and make a mess. Preschoolers stomp in water or mud puddles to see what will happen and then continue to jump more as they realize it makes things spray up and make a mess. Kindergartner find a worm, ladybug, spider, or other insect and follow it around the yard, pick it up and observe it.
Children are little scientists. It is us adults that teach them to stop exploring and trying new things when we harshly yell stop blowing bubbles in your milk instead of saying, it made a mess when you blew bubbles in your milk didn’t it. Let’s try a different activity. They need to know guidelines, methods to explore around them so they can investigate without causing mayhem or large messes.

Capillary action science experiment

Capillary experiment
- 5-10 drops of red food coloring to glass 1 and 7
- 15 drops of yellow food coloring to glass 3
- 5-10 drops food coloring to glass 5

Capillary action experiments

Capillary action kids

Capillary action activity

Capillary action experiment paper towel
Capillary action is the process in which a liquid moves up something solid, like a tube or into a material with a lot of small holes. This happens when 3 forces called cohesion, adhesion, and surface tension work together. Water molecules are considered cohesive (sticky to each other) and they adhere (stick) to the paper towel. As one water molecule moves up the paper towel it pulls the other molecules with it. The molecules pull each other along like a drawstring.

What is capillary action for kids

Capillary Experiment

Water capillary action experiment

Capillary action experiment explanation
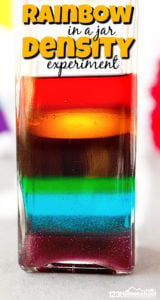
Rainbow Science
Looking for fun rainbow experiment ideas to get kids excited about exploring the world around them? Don’t miss these clever rainbow activity ideas!
- How to Grow Rainbow Crystals EASILY at Home – Spring Science Activity
- Rainbow in a Jar Density Experiments
- Simply Rainbow Density Experiment for springtime
- Delicious Rainbow Stem scienc with jelly beans
- Frozen Erruptions – Rainbow Science Activities for Preschoolers
- Colorful Capillary Action Experiment
- Easy Rainbow in a jar experiment with sugar
- Amazing growing a rainbow capillary action experiment
- Colorful candy science experiment
- Magical Color Changing Milk Experiment
- Colorful Polymer Experiments
- Beautiful Food Coloring Flower Experiment Worksheet
- Heat-Sensitive Color Chaning Slime
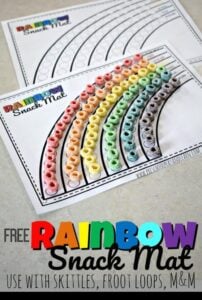
Rainbow Printables
Snag these rainbow worksheets and printable rainbow activities to make learning fun this spring:
- Free printable Rainbow Color Matching Game
- Rainbow Rhyming Activity for Kindergarten
- Rainbow Worksheets for kids
- Free Connect the Dot Rainbow Printable for Preschoolers
- Hands-on Rainbow Math Activities for Preschoolers
- Rainbow Playdough Shape Mat
- Lots of fun ideas in this Rainbow Preschool Theme

Rainbow Crafts and Activities
Looking for some really cute, simple, and beautiful rainbow craft ideas ? Check out these fun rainbow crafts for preschoolers!
- Yarn Wrapped Rainbow Craft for Kids
- Paper Plate Rainbow Craft for Preschoolers
- Rainbow Handprint Craft
- Easy Tissue Paper Rainbow Craft for Spring
- Craft Stick Rainbow Craft
- Beautiful Rainbow Slime Activity for Kids
- Rainbow Toast makes a yummy snack!
- Rainbow Painting with Cars Activity
- Super cute Rainbow Math with a DIY abacus activity
- Foam Puzzle Rainbow Activity for Preschoolers
- Bubble Snake Rainbow Activities
Looking for more fun, creative ways you can begin your free homeschool ? We have over 1,000,000 pages of FREE Printable Worksheets including resources for: pre k worksheets , kindergarten worksheets , 1st grade worksheets , 2nd grade worksheets , 3rd grade worksheets , 4th grade worksheets , 5th grade worksheets , 6th grade worksheets , and more. Plus see our history lessons for kids , printable math games , language arts worksheets , sight word worksheets , free alphabet printables , and cvc word activities for kids of all ages!

In addition, don’t miss our disney world planning and kids activities filled with ideas for every holiday and season of the year!
Beth Gorden
Beth Gorden is the creative multi-tasking creator of 123 Homeschool 4 Me. As a busy homeschooling mother of six, she strives to create hands-on learning activities and worksheets that kids will love to make learning FUN! She has created over 1 million pages of printables to help teach kids ABCs, science, English grammar, history, math, and so much more! Beth is also the creator of 2 additional sites with even more educational activities and FREE printables – www.kindergartenworksheetsandgames.com and www.preschoolplayandlearn.com. Beth studied at the University of Northwestern where she got a double major to make her effective at teaching children while making education FUN!
Leave a Reply Cancel reply
Your email address will not be published. Required fields are marked *
Adorei a pesquisa ,gostaria de saber mais ou menos quanto tempo de processo até o produto final?
30 minutos, no mas!

Soft Matter
Force decomposition and toughness estimation from puncture experiments in soft solids.
Several medical applications, like drug delivery and biosensing, are critically preceded by the insertion of needles and microneedles into biological tissue. However, the mechanical process of needle insertions, especially at high velocities, is currently not fully understood. Here, we explore the insertion of hollow needles into transparent silicone samples with an insertion velocity v ranging from 0.1 mm/s to 2.3 m/s (with needle radius R=101.5 µm, thus strain rates ~ v/R ranging from 1 s -1 to 2.3×10 4 s -1 ). We use a double-insertion method, where the needle is inserted and re-inserted at the same location, to estimate the fracture properties of the material. The deflection of the specimen free surface is found to be different between insertion and re-insertion experiments for identical needle positions, which is associated with different force magnitudes between insertion/reinsertion. This aspect was previously neglected in the original double-insertion method, thus here we develop a method based on imaging, image analyses and force measurements to decompose the measured force into individual force components, including deflection force F d , frictional and spreading force F f +F s , and cutting force F t . We estimate that the toughness Γ of our silicone samples, calculated using the cutting force F t and the crack dimensions, increases with needle velocity, and ranges within observed values in previous literature for the same material and for some soft biological materials. In addition to toughness Γ, other parameters, such as critical force F c and mechanical work W c , also show strain-rate dependence, suggesting tissue stiffening, due to accumulated strain energy, at high-speeds.
Supplementary files
- Supplementary information PDF (2288K)
Article information
Download citation, permissions.

P. Shrestha, C. Geffner, M. Jaffey, Z. Wu, M. Iapichino, M. Bacca and B. Stoeber, Soft Matter , 2024, Accepted Manuscript , DOI: 10.1039/D4SM00211C
To request permission to reproduce material from this article, please go to the Copyright Clearance Center request page .
If you are an author contributing to an RSC publication, you do not need to request permission provided correct acknowledgement is given.
If you are the author of this article, you do not need to request permission to reproduce figures and diagrams provided correct acknowledgement is given. If you want to reproduce the whole article in a third-party publication (excluding your thesis/dissertation for which permission is not required) please go to the Copyright Clearance Center request page .
Read more about how to correctly acknowledge RSC content .
Social activity
Search articles by author.
This article has not yet been cited.
Advertisements
Influence of Capillary Adhesion on the Sliding of a Cylinder Along the Surface of an Elastic Solid Taking Account of Wetting Hysteresis
- Makhovskaya, Yu. Yu.
The contact problem of steady sliding of a rigid cylinder over an elastic half-space in the presence of liquid menisci is considered, taking into account the hysteresis of the contact angle, which leads to different adhesion conditions at the entrance to the contact and at the exit from it. The problem was considered in a flat formulation and was solved by reducing it to the Riemann-Hilbert problem. An analytical expression for the contact pressure and a system of four equations for the numerical determination of the coordinates of the ends of the contact area and the meniscus zones are obtained. The calculation was carried out in the range of input parameters corresponding to the situation when a sliding cylinder models a separate protrusion of a rough surface. The distribution of contact pressure, the size of the contact area and its displacement relative to the axis of symmetry of the cylinder, the width of the menisci at the entrance to and exit from the contact, as well as the friction force caused by capillary adhesion were studied. It has been established, in particular, that the friction force significantly depends on the value of the contact angle hysteresis, and especially on the surface tension of the liquid, but weakly depends on the capillary pressure in the menisci, which, under conditions of thermodynamic equilibrium of the meniscus with the environment, is determined by the humidity of the surrounding air.
- elastic contact;
- capillary adhesion;
- contact angle hysteresis;
- sliding friction

IMAGES
VIDEO
COMMENTS
Capillary action is fluid flow through a narrow tube or space from surface tension, cohesion, and adhesion. For example, if you place a thin tube into water, the water flows up the the tube. Other names for the phenomenon are capillarity, capillary motion, and wicking. Capillary action does not require the force of gravity.
Capillary action of water (polar) compared to mercury (non-polar), in each case with respect to a polar surface such as glass (≡Si-OH). Capillary action (sometimes called capillarity, capillary motion, capillary rise, capillary effect, or wicking) is the process of a liquid flowing in a narrow space in opposition to or at least without the assistance of any external forces like gravity.
The height to which a liquid will rise in a capillary tube is determined by several factors as shown in the following equation: h = 2T cosθ rρg (7.1.1) (7.1.1) h = 2 T cos θ r ρ g. where. h is the height of the liquid inside the capillary tube relative to the surface of the liquid outside the tube, T is the surface tension of the liquid, θ ...
Capillary action is a phenomenon in which liquid flows through a narrow tube without the assistance of any force. It refers to the ascension of liquid through a capillary tube due to forces of adhesion and cohesion and, typically, against gravity. Capillary action occurs when the force of adhesion is greater than the force of cohesion. For ...
Capillary action is defined as the spontaneous flow of a liquid into a narrow tube or porous material. This movement does not require the force of gravity to occur. In fact, it often acts in opposition to gravity. Capillary action is sometimes called capillary motion, capillarity, or wicking. Capillary action is caused by the combination of ...
Forces in Capillary Action. Three main variables that determine whether a liquid possesses capillary action are: Cohesive force: It is the intermolecular bonding of a substance where its mutual attractiveness forces them to maintain a certain shape of the liquid.; Surface tension: This occurs as a result of like molecules, cohesive forces, banding together to form a somewhat impenetrable ...
Capillary action draws the ink/water up the tubes to varying heights. figure 1. Capillary tubes in action. Setting it up: Clamp the tubes to a lab stand and lower them into a petri dish. It is best to mix the ink and water before setting down the tubes. Be generous with the ink (black, blue or red); the setup needs to be illuminated from behind ...
See Figure 9 in this reference—this experiment is over 100 years old! It demonstrates how something called capillary action can lift water up higher in narrower spaces. ... called a meniscus, that curls up around the edge of the glass. This occurs because of two forces: adhesion (the attraction between the water molecules and the glass) and ...
Capillary action is important for moving water (and all of the things that are dissolved in it) around. It is defined as the movement of water within the spaces of a porous material due to the forces of adhesion, cohesion, and surface tension. Capillary action occurs because water is sticky, thanks to the forces of cohesion (water molecules ...
Forces in Capillary Action. Three main variables that determine whether a liquid possesses capillary action are: Cohesive force: It is the intermolecular bonding of a substance where its mutual attractiveness forces them to maintain a certain shape of the liquid.; Surface tension: This occurs as a result of like molecules, cohesive forces, banding together to form a somewhat impenetrable ...
Here is a simple capillary action definition to share with kids. Capillary action is the ability of a liquid to flow in narrow spaces without the help of an outside force, like gravity. Try This: Capillary action looks a bit like magic! Imagine you have a tiny straw, so tiny that you can't even see it. Now, dip one end of the straw into a ...
The height to which capillary forces can draw the liquid up depends on. 1) the size of the air gap between the two glass slides, 2) the surface tension, 3) the liquid's density - due to the gravity's pull, and. 4) the molecular (adhesive) forces between the liquid and the surface of the solid (here glass).
Water moves through the plant by means of capillary action. Capillary action occurs when the forces binding a liquid together (cohesion and surface tension) and the forces attracting that bound liquid to another surface ( adhesion) are greater than the force of gravity. The plant's stem sucks up water much like a straw does.
The experiment shows that the capillary force obtained by the 3-D model accurately describes the vertical and tangential forces of the clam-shell droplet on the fiber. Sharp shrinking of the cross-section on the droplet's upper part results in an exponential increase in tensile and tangential stresses, which makes the droplet break or move on ...
This rainbow science experiment is as magic as the science behind it. The colored water travels up the paper towel by a process called capillary action. Capillary action is the ability of a liquid to flow upward, against gravity, in narrow spaces. This is the same thing that helps water climb from a plant's roots to the leaves in the tree tops.
18.357 Interfacial Phenomena, Lecture 8. 8. Capillary Rise. Capillary rise is one of the most well-known and vivid illustrations of capillarity. It is exploited in a number of biological processes, including drinking strategies of insects, birds and bats and plays an important role in a number of geophysical settings, including flow in porous ...
The capillary force between two disks is typically repulsive at small distances, and becomes attractive with increasing separation distance for a fixed liquid volume. In addition, the radius ratio of the two disks has a crucial effect on the capillary forces.
This article was originally published with the title " Experiments in Capillary Force " in Scientific American Magazine Vol. 60 No. 3 (January 1889), p. 39 doi:10.1038 ...
About this book. Capillary Forces in Microassembly discusses the use of capillary forces as a gripping principle in microscale assembly. Clearly written and well-organized, this text brings together physical concepts at the microscale with practical applications in micromanipulation. Throughout this work, the reader will find a review of the ...
The chapter investigates imbibition into a porous medium under microgravity condition under the action of capillary forces. The study of capillary effects in terrestrial conditions is often difficult due to the influence of gravity, which makes it necessary to conduct experiments in microgravity. The chapter describes the features of experiments and the processing of experimental data in two ...
Capillary forces are commonly encountered in nature because of the spontaneous condensation of liquid from surrounding vapor, leading to the formation of a liquid bridge. In most cases, the advent of capillary forces by condensation leads to undesirable events such as an increase in the strength of granules, which leads to flow problems and/or caking of powder samples. The prediction and ...
A series of core flooding experiments were conducted to investigate the interplay of capillary and viscous forces in immiscible displacements. Water and crude oil were employed as defending fluid and different states of CO 2 were injected as invading fluid with various injection rates, which obtains a range of capillary numbers and viscosity ...
This capillary action experiment will amaze kids from toddlers, preschoolers, and kindergarteners to grade 1, grade 2, and grade 3 students. You only need a couple simple materials you most likely already ahve tohome: paper towels, water, and food coloring to watch capillary action in most with this beautiful capiarlly action science experiment.
Capillary Flow Experiment (CFE) This is a video clip of the Capillary Flow Experiment, Vane Gap -1 vessel at 45° vane angle in microgravity, µg. A large "finger" of fluid has filled the gap between the vane and chamber inside the surface closest to the camera view, at the critical angle of 45° (within ±1.5° of predicted value). The key ...
Check out these fun ways to demonstrate capillary action. Plus, all you need is a handful of standard household supplies. COLOR CHANGING FLOWERS. Learn about the forces of capillary action as you change your flowers from white to green. Or any color you like! Easy to set up and perfect for a group of kiddos to do simultaneously.
The results of the linear analysis agree with the preexisting experiments of de Bruyn [Phys. Fluids 9, 1599 (1997), 10.1063/1.869280] and nonlinear simulations of Weidner et al. [J. Colloid Interface Sci. 187, 243 (1997), 10.1006/jcis.1996.4711] in the limit of a thin film and extend the results for thick films. ... Interplay of capillary and ...
Capillary instability arises when the cohesive forces at the interface between two fluids are disrupted by perturbations, leading to the formation of characteristic patterns such as waves or droplets. The influence of gravity and fluid flow velocity is disregarded in the context of capillary instability analyses.
Force decomposition and toughness estimation from puncture experiments in soft solids ... In addition to toughness Γ, other parameters, such as critical force F c and mechanical work W c, also show strain-rate dependence, suggesting tissue stiffening, due to accumulated strain energy, at high-speeds.
capillary pull, a linear relationship exists between the minimum radius and the observed light ... Experiments performed using preexisting glass tubes con rm these results; thus, it will be possible to implement this metrology into the capillary pulling process. The raytracer developed for this project is the rst fully 3D capillary raytracing ...
The contact problem of steady sliding of a rigid cylinder over an elastic half-space in the presence of liquid menisci is considered, taking into account the hysteresis of the contact angle, which leads to different adhesion conditions at the entrance to the contact and at the exit from it. The problem was considered in a flat formulation and was solved by reducing it to the Riemann-Hilbert ...