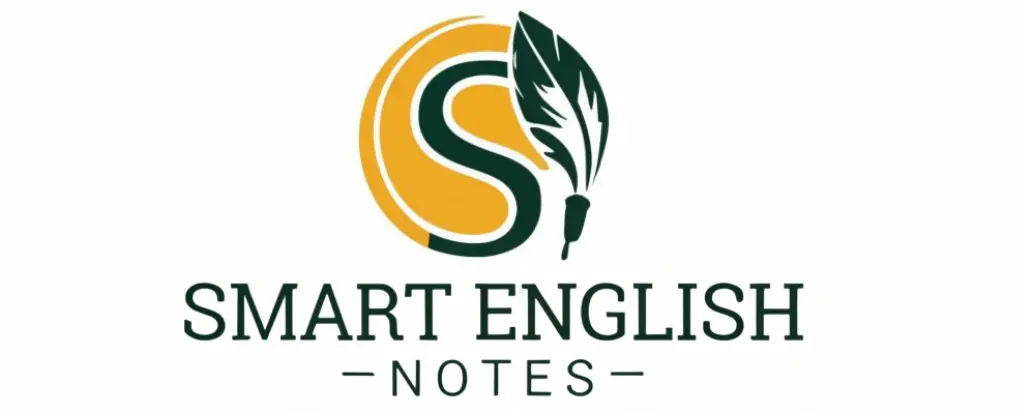

My School Laboratory Essay
Our school laboratory essay.
We are living in the world of science and technology. So, the need to understand scientific concepts and theories is extremely great. In response to this dire need, facilities for the study of physical sciences have been given great extension in both the higher and lower centers of education. But to understand the modern scientific concepts we can not do without a well-equipped laboratory. It‘s necessary that an educational institute has a good laboratory to cater to the needs of the students.
Our school is well known for imparting quality education, especially in science stream. The credit for this fame goes to our expert science teachers and the available sophisticated fine laboratory.
Our school laboratory is located in the third storey of the school building. It comprises of three separate halls specified for three main branches i.e. Physics, Chemistry, and Biology. Each hall is quite spacious (big) to accommodate fifty students at a time. The halls are well ventilated and have a very good lighting arrangement. These are well electrified to meet the students, demands. The system is designed to cater for individual and group work.
Please enable JavaScript
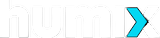
Our laboratory is equipped with the most modern, standard and sophisticated equipment. The apparatuses are available in abundance. The chemicals and specimens available are always fresh and latest. Every scientific concept is clarified in the laboratory by our highly educated teachers. Occasionally, expert scholars and scientists are invited to demonstrate the latest concepts. It has helped in developing a scientific approach among young scholars.
I wish our laboratory be given further development and expansion so that it proves a source of inspiration for other educational institutions to establish such ideal laboratories.
2 thoughts on “My School Laboratory Essay”
- Pingback: Essay Writing: Types, Components/Format , Characteristics and Samples - Smart eNotes
- Pingback: How to Write a Paragraph - Smart eNotes
Have something to say Cancel reply
This site uses Akismet to reduce spam. Learn how your comment data is processed .
Discover more from Smart English Notes
Subscribe now to keep reading and get access to the full archive.
Type your email…
Continue reading
- English Grammar Free Course
- English Grammar Tutorial
- Parts of Speech
- Figure of Speech
- Tenses Chart
- Essay Writing
- Email Writing
- NCERT English Solutions
- English Difference Between
- SSC CGL English Syllabus
- SBI PO English Syllabus
- SBI Clerk English Syllabus
- IBPS PO English Syllabus
- IBPS CLERK English Syllabus
Essay on Science in English: Check 200, 300 & 500 Words Essay
Science is the study of logic. It explains why the world is round, why stars twinkle, why light travels faster than sound, why hawks soar higher than crows, why sunflowers face the sun and other phenomena. Science answers every question logically rather than offering mystical interpretations. Students are very interested in science as a topic. This subject is indeed crucial for those hoping to pursue careers in science and related professions.
People who are knowledgeable in science are more self-assured and aware of their environment. Knowing the cause and origin of natural events, a person knowledgeable in science will not be afraid of them.
However, science also has a big impact on a country's technological advancement and illiteracy.
Table of Content
English-language Long and Short Science Essay
Essay on science (200 words), essay on science (300 words), essay on science (400 words), essay on science (500 words), essay on science (600 words).
We have included a brief and lengthy English essay on science below for your knowledge and convenience. The writings have been thoughtfully crafted to impart to you the relevance and meaning of science. You will understand what science is, why it matters in daily life, and how it advances national progress after reading the writings. These science essays can be used for essay writing, debate, and other related activities at your institution or school.
Science entails a thorough examination of the behavior of the physical and natural world. Research, experimentation, and observation are used in the study.
The scientific disciplines are diverse. The social sciences, formal sciences, and natural sciences are some of them. Subcategories and sub-sub-categories have been created from these basic categories. The natural sciences include physics, chemistry, biology, earth science, and astronomy; the social sciences include history, geography, economics, political science, sociology, psychology, social studies, and anthropology; and the formal sciences include computer science, logic, statistics, decision theory, and mathematics.
The world has positively transformed because of science. Throughout history, science has produced several inventions that have improved human convenience. We cannot fathom our lives without several of these inventions since they have become essential parts of them.
Global scientists persist in their experiments and occasionally produce more advanced innovations, some of which spark global revolutions. Even if science is helpful, some people have abused knowledge, usually those in positions of authority, to drive an arms race and destroy the environment.
There is no common ground between the ideologies of science and religion. These seeming opposite viewpoints have historically led to a number of confrontations and still do.
Science is a way to learn about, comprehend, examine, and experiment with the physical and natural features of the world in order to apply it to the development of newer technologies that improve human convenience. In science, observation and experimentation are broad and not restricted to a specific concept or area of study.
Applications of Science
Science has given us almost everything we use on a daily basis. Everything, from laptops to washing machines, microwaves to cell phones, and refrigerators to cars, is the result of scientific experimentation. Here are some ways that science affects our daily lives:
Not only are refrigerators, grills, and microwaves examples of scientific inventions, but gas stoves, which are frequently used for food preparation, are as well.
Medical Interventions
Scientific advancements have made it feasible to treat a number of illnesses and conditions. Thus, science encourages healthy living and has helped people live longer.
Interaction
These days, mobile phones and internet connections are necessities in our life and were all made possible by scientific advancements. These innovations have lowered barriers to communication and widened global connections.
E nergy Source
The creation and application of numerous energy forms have been facilitated by the discovery of atomic energy. One of its greatest innovations is electricity, and everyone is aware of the effects it has on daily life.
Variety in Cuisine
There has also been an increase in food diversity. These days, a wide variety of fruits and vegetables are available year-round. It's not necessary to wait for a given season to enjoy a certain meal. This modification is the result of scientific experimentation.
So, science is a part of our daily existence. Without scientific advancements, our lives would have been considerably more challenging and varied. Nonetheless, we cannot ignore the fact that a great deal of scientific innovation has contributed to environmental deterioration and a host of health issues for humankind.
There are essentially three main disciplines of science. The Natural Sciences, Social Sciences, and Formal Sciences are some of them. To examine different aspects, these branches are further divided into subcategories. This is a thorough examination of these groups and their subgroups.
Scientific Subdisciplines
Natural Science
This is the study of natural phenomena, as the name implies. It investigates how the cosmos and the world function. Physical science and life science are subcategories of natural science.
a) Science of Physics
The subcategories of physical science comprise the following:
- Physics is the study of matter's and energy's properties.
- Chemistry is the study of the materials that make up matter.
- The study of space and celestial bodies is called astronomy.
- Ecology is the study of how living things interact with their natural environments and with one another.
- Geology: It studies the composition and physical makeup of Earth.
- Earth science is the study of the atmosphere and the physical makeup of the planet.
- The study of the physical and biological components and phenomena of the ocean is known as oceanography.
- Meteorology: It studies the atmospheric processes.
The subcategories of life science include the following:
- The study of living things is called biology.
- The study of plants is known as botany.
- The study of animals is known as zoology.
c) Social Science
This includes examining social patterns and behavioral patterns in people. It is broken down into more than one subcategory. Among them are:
- History: The examination of past occurrences
- Political science is the study of political processes and governmental structures.
- Geographic: Study of the atmospheric and physical characteristics of Earth.
- Human society is studied in social studies.
- Sociology: The study of how societies form and operate.
Academic Sciences
It is the area of study that examines formal systems like logic and mathematics. It encompasses the subsequent subcategories:
- Numbers are studied in mathematics.
- Reasoning is the subject of logic.
- Statistics: It is the study of numerical data analysis.
- Mathematical analysis of decision-making in relation to profit and loss is known as decision theory.
- The study of abstract organization is known as systems theory.
- Computer science is the study of engineering and experimentation as a foundation for computer design and use.
Scientists from several fields have been doing in-depth research and testing numerous facets of the subject matter in order to generate novel ideas, innovations, and breakthroughs. Although these discoveries and technologies have made life easier for us, they have also permanently harmed both the environment and living things.
Introduction
Science is the study of various physical and natural phenomena' structures and behaviors. Before drawing any conclusions, scientists investigate these factors, make extensive observations, and conduct experiments. In the past, science has produced a number of inventions and discoveries that have been beneficial to humanity.
I deas in Religion and Science
In science, new ideas and technologies are developed through a methodical and rational process; in religion, however, beliefs and faith are the only factors considered. In science, conclusions are reached by careful observation, analysis, and experimentation; in religion, however, conclusions are rarely reached through reason. As a result, they have very different perspectives on things.
Science and Religion at Odds
Because science and religion hold different opinions on many issues, they are frequently perceived as being at odds. Unfortunately, these disputes occasionally cause social unrest and innocent people to suffer. These are a few of the most significant disputes that have happened.
The World's Creation
The world was formed in six days, according to many conservative Christians, sometime between 4004 and 8000 BCE. However, cosmologists assert that the Earth originated about 4.5 billion years ago and that the cosmos may be as old as 13.7 billion years.
The Earth as the Universe's Center
Among the most well-known clashes is this one. Earth was considered to be the center of the universe by the Roman Catholic Church. They say that it is surrounded by the Sun, Moon, stars, and other planets. Famous Italian mathematician and astronomer Galileo Galilei's discovery of the heliocentric system—in which the Sun is at the center of the solar system and the Earth and other planets orbit it—led to the conflict.
Eclipses of the Sun and Moon
Iraq was the scene of one of the first wars. The locals were informed by the priests that the moon eclipse was caused by the gods' restlessness. These were seen as foreboding and intended to overthrow the kings. When the local astronomers proposed a scientific explanation for the eclipse, a disagreement arose.
There are still many myths and superstitions concerning solar and lunar eclipses around the world, despite astronomers providing a compelling and rational explanation for their occurrence.
In addition to these, there are a number of other fields in which religious supporters and scientists hold divergent opinions. While scientists, astronomers, and biologists have evidence to support their claims, the majority of people adhere closely to religious beliefs.
Not only do religious activists frequently oppose scientific methods and ideas, but many other facets of society have also taken issue with science since its discoveries are leading to a host of social, political, environmental, and health problems. Nuclear weapons are one example of a scientific invention that threatens humanity. In addition, the processes involved in preparation and the utilization of the majority of scientifically created equipment contribute to pollution, making life more difficult for all.
In the previous few decades, a number of scientific advancements and discoveries have greatly eased people's lives. The previous ten years were not an anomaly. A good number of important scientific discoveries were acknowledged. The top ten most amazing recent scientific inventions are shown below.
New Developments and Findings in Science
Amputee Gains Control of Biomechanical Hand via Mental After a tragic accident took away his forearm, Pierpaolo Petruzziello, an Italian, used his mind to control a biomechanical hand attached to his arm. The hand used wires and electrodes to connect to the nerves in his arm. He became the first to become skilled at doing motions like gripping objects, wriggling his fingers, and moving.
The Global Positioning System
In 2005, the Global Positioning System, or GPS as it is more often known, went into commercial use. It was incorporated into mobile devices and worked wonders for tourists all over the world. Traveling to more recent locations and needing instructions couldn't be simpler.
The Self-Driving Car Toyota debuted Prius shortly after Google launched its own self-driving car experiment in 2008. The accelerator, steering wheel, and brake pedals are absent from this vehicle. It runs without the need for user input because it is driven by an electric motor. To guarantee that the driverless experience is seamless and secure, it is integrated with specialized software, a collection of sensors, and precise digital maps.
Android, widely regarded as one of the most significant innovations of the decade, revolutionized the market by flooding it with devices running Java and Symbian earlier on. These days, Android is the operating system used by the majority of smartphones. Millions of applications are supported by it.
c) Computer Vision
A number of sub-domains fall under the umbrella of computer vision, including learning, video tracking, object recognition, object pose estimation, event detection, indexing, picture restoration, and scene reconstruction. In order to produce symbolic information, the field includes methods for processing, analyzing, obtaining, and understanding images in high-dimensional data from the real world.
d) Touch Screen Technology
It appears that touch screen technology has taken over the planet. The popularity of touch screen gadgets can be attributed to their ease of use. These gadgets are becoming quite popular everywhere.
e) Method of 3D Printing
The 3D printer is capable of producing a wide range of items, such as lamps, cookware, accessories, and much more. Alternatively referred to as additive manufacturing, this process uses digital model data from electronic data sources like Additive Manufacturing Files (AMF) to construct three-dimensional items of any shape.
Git Hub is an online hosting service and version control repository that was founded in 2008. It provides features including bug tracking, task management, feature requests, and the sharing of codes, apps, and other materials. The GitHub platform was first developed in 2007, and the website went live in 2008.
f) Smart Timepieces
The market for smart watches has been around for a while. The more recent models, like the one introduced by Apple, have garnered enormous popularity and come with a number of extra capabilities. Nearly all of the functionality found on smartphones are included in these watches, which are also more convenient to wear and use.
g) Websites for Crowdfunding
The emergence of crowdsourcing websites like Indiegogo, Kickstarter, and GoFundMe has been a blessing for innovators. Inventors, artists, and other creative people can share their ideas and gain the funding they need to put them into action by using these websites.
Global scientists constantly observe and experiment to develop new scientific discoveries that improve people's lives. Not only do they consistently create new technologies, but they also adapt the ones that already exist whenever there is an opportunity. Even while these innovations have made life easier for humans, you are all aware of the numerous environmental, social, and political risks they have brought about.
500+ Words Essay on Mother Teresa in English For Students 500+ Words Essay on Swami Vivekananda in English for Students Rabindranath Tagore Essay in English For Students APJ Abdul Kalam Essay For Students: Check 500 Words Essay
Essay on Science- FAQs
Who is father of science.
Galileo is the father of science.
Why is it called science?
The word "scientia" has Latin origins and originally meant "knowledge," "an expertness," or "experience."
What is science for students?
Science is the study of the world by observation, recording, listening, and watching. Science is the application of intellectual inquiry into the nature of the world and its behavior. Think like a scientist, anyone can.
What is science's primary goal or objective?
Science's primary goal is to provide an explanation for the facts. Moreover, science does not prohibit the explanation of facts in an arbitrary manner. Additionally, science organizes the data and develops theories to explain the data.
Describe what a scientific fact is.
Repeatable, meticulous observations or measurements made through experiments or other methods are referred to as scientific facts. Furthermore, empirical evidence is another name for a scientific fact. Most importantly, the development of scientific hypotheses depends on scientific facts.
Similar Reads
- School English
- English Blogs
Improve your Coding Skills with Practice
What kind of Experience do you want to share?
The Ultimate Guide to Science Lab Essay Structure and Format
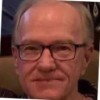
The science lab essay is a fundamental component of scientific study, serving as a detailed record of an experiment and its results. It follows a specific structure and format, designed to ensure clarity, consistency, and comprehensibility. This essay will delve into the structure and format of a science lab essay, discussing their importance, common mistakes, and how to avoid them.
<h2 style="font-weight: bold; margin: 12px 0;">What is the structure of a science lab essay?</h2>A science lab essay typically follows a specific structure, which includes an introduction, methods, results, discussion, and conclusion. The introduction sets the stage for the experiment, providing background information and stating the purpose of the study. The methods section details the procedures used in the experiment, while the results section presents the data collected. The discussion section interprets these results, and the conclusion summarizes the findings and suggests future research directions. This structure ensures that the essay is organized, logical, and easy to follow, allowing readers to understand the experiment and its significance.
<h2 style="font-weight: bold; margin: 12px 0;">How to format a science lab essay?</h2>Formatting a science lab essay involves adhering to specific guidelines. These may vary depending on the academic institution or the scientific journal where the essay will be published. However, some common elements include a title page, abstract, introduction, methods, results, discussion, conclusion, references, and appendices if necessary. The essay should be typed in a clear, readable font, usually Times New Roman 12 point. Margins should be set to 1 inch on all sides, and the text should be double-spaced. All pages should be numbered, and the title of the essay should appear on the first page.
<h2 style="font-weight: bold; margin: 12px 0;">Why is the structure and format important in a science lab essay?</h2>The structure and format of a science lab essay are crucial for several reasons. Firstly, they ensure that the essay is organized and logical, making it easier for readers to follow the experiment and understand its results. Secondly, they allow for consistency in scientific reporting, which is essential for the replication of studies and the advancement of scientific knowledge. Lastly, they ensure that all necessary information is included in the essay, preventing any misunderstandings or misinterpretations.
<h2 style="font-weight: bold; margin: 12px 0;">What are common mistakes in structuring and formatting a science lab essay?</h2>Common mistakes in structuring and formatting a science lab essay include not following the prescribed structure, omitting important sections, and not adhering to formatting guidelines. For example, some students may neglect to include a methods section, making it impossible for others to replicate their experiment. Others may fail to double-space their text or use the correct font, making their essay difficult to read. These mistakes can lead to confusion, misinterpretation of results, and even rejection of the essay by academic institutions or scientific journals.
<h2 style="font-weight: bold; margin: 12px 0;">How to avoid mistakes in structuring and formatting a science lab essay?</h2>To avoid mistakes in structuring and formatting a science lab essay, students should familiarize themselves with the required structure and format before they begin writing. They should also proofread their essay carefully, checking for any missing sections or formatting errors. Using a checklist can be helpful in ensuring that all necessary elements are included. Additionally, students should seek feedback from their instructors or peers, as they may be able to spot mistakes that the student has overlooked.
In conclusion, the structure and format of a science lab essay are crucial for effective scientific communication. They ensure that the essay is organized, logical, and easy to follow, allowing readers to understand the experiment and its significance. By adhering to these guidelines, students can produce high-quality science lab essays that contribute to the advancement of scientific knowledge.

Related Essays
Mastering the science lab: a guide to writing effective essays.
Mastering the science lab essay is a crucial skill for students and researchers alike. It requires a clear understanding of the scientific method, a knack for detailed observation, and the ability to communicate complex ideas in a clear and concise manner. This essay will delve into the key components of an effective science lab essay, the importance of clarity and coherence, the role of scientific terminology, the significance of the conclusion, and strategies for improving science lab essay writing skills. What are the key components of an effective science lab essay?An effective science lab essay is composed of several key components. Firstly, it should have a clear and concise introduction that provides an overview of the experiment, including the purpose and the hypothesis. This is followed by the materials and methods section, which details the procedures followed and the equipment used in the experiment. The results section comes next, presenting the data collected during the experiment in an organized and understandable manner. This could be in the form of tables, graphs, or charts. The discussion section follows, interpreting the results and comparing them with the initial hypothesis. Lastly, the conclusion summarizes the findings and suggests further research if necessary. Each section should be written in a clear, concise, and logical manner, using appropriate scientific terminology. How can one ensure clarity and coherence in a science lab essay?Ensuring clarity and coherence in a science lab essay requires careful planning and organization. Start by outlining the essay, detailing what each section will cover. Use clear, concise language and avoid jargon or overly complex terms. Each paragraph should focus on a single idea or result, and the transition between paragraphs should be smooth and logical. Use visual aids like tables, graphs, and charts to present data, but make sure they are properly labeled and referenced in the text. Lastly, proofread the essay multiple times to check for errors and inconsistencies. Why is it important to use scientific terminology in a science lab essay?Using scientific terminology in a science lab essay is crucial for several reasons. Firstly, it ensures precision and accuracy in describing the experiment and its results. Scientific terms have specific meanings, reducing the chance of misinterpretation. Secondly, it demonstrates a good understanding of the subject matter, which can enhance the credibility of the essay. Lastly, it aligns with the conventions of scientific writing, making the essay more professional and acceptable in academic settings. What role does the conclusion play in a science lab essay?The conclusion plays a significant role in a science lab essay. It serves to summarize the key findings of the experiment, relating them back to the initial hypothesis. It provides an opportunity to interpret the results in a broader context, discussing their implications and relevance. The conclusion also suggests areas for further research, acknowledging the limitations of the current experiment. It should be concise, clear, and compelling, leaving the reader with a strong understanding of the experiment's significance. How can one improve their science lab essay writing skills?Improving science lab essay writing skills can be achieved through several strategies. Regular practice is key, as it helps to familiarize oneself with the structure and style of scientific writing. Reading scientific literature can also be beneficial, providing examples of well-written essays. Seeking feedback from teachers or peers can provide valuable insights into areas of improvement. Additionally, attending workshops or courses on scientific writing can provide further guidance and tips. Lastly, proofreading and editing are crucial steps in refining the essay and ensuring its clarity and coherence.In conclusion, writing an effective science lab essay is a multifaceted task that requires a deep understanding of the scientific process, a keen eye for detail, and strong writing skills. By understanding the key components of a lab essay, ensuring clarity and coherence, using appropriate scientific terminology, crafting a compelling conclusion, and continually seeking to improve, one can master the art of science lab essay writing. This not only aids in academic success but also prepares one for future scientific endeavors.
Science Lab Report Writing: Tips and Tricks for Success
Writing a science lab report is a fundamental part of any scientific investigation. It not only documents the details of the experiment but also demonstrates the scientific process in action. A well-written lab report communicates the purpose, method, findings, and implications of an experiment clearly and effectively. This essay provides insights into the key components of a lab report and offers tips for writing each section effectively. What are the key components of a science lab report?A science lab report typically consists of several key components, each serving a specific purpose. The first component is the title, which should be concise yet descriptive, providing a clear indication of the experiment's subject. Following the title is the abstract, a brief summary of the entire report, including the purpose of the experiment, the method used, the main findings, and the conclusions drawn. The introduction provides background information and outlines the objectives and hypothesis of the experiment. The methods and materials section details the procedures followed and the equipment used, allowing others to replicate the experiment. The results section presents the data collected, often in the form of tables, graphs, or charts. The discussion or analysis section interprets these results, and the conclusion summarizes the findings and suggests areas for further research. Lastly, references are included to acknowledge any sources used. How to write an effective introduction for a science lab report?An effective introduction for a science lab report should provide sufficient background information to allow the reader to understand the context and significance of the experiment. It should start with a broad overview of the topic, gradually narrowing down to the specific research question or hypothesis. The introduction should also clearly state the objectives of the experiment and explain why it is being conducted. It's important to keep the language clear and concise, avoiding unnecessary jargon. While the introduction should be informative, it should also be engaging to capture the reader's interest. What is the importance of the methods and materials section in a science lab report?The methods and materials section of a science lab report is crucial as it provides the details necessary for others to replicate the experiment. This section should describe the procedures followed, the equipment used, and any materials involved in the experiment. It should be written in a clear, step-by-step manner, ensuring that every detail is included. This section is not just a list of instructions, but a record of what was actually done during the experiment. It allows other researchers to verify the results by repeating the experiment, contributing to the scientific method's emphasis on reproducibility and transparency. How to effectively present results in a science lab report?Presenting results effectively in a science lab report involves clear, concise, and accurate reporting of the data collected during the experiment. This section should include all relevant data, presented in an organized manner, often in the form of tables, graphs, or charts. It's important to remember that this section is purely for presenting data, not interpreting it. Any figures or tables used should be clearly labeled, and any statistical analyses should be explained. The results section should be written in the past tense, as it describes what was observed during the experiment. What should be included in the conclusion of a science lab report?The conclusion of a science lab report should summarize the main findings of the experiment and relate them back to the objectives and hypothesis outlined in the introduction. It should discuss whether the results support the hypothesis, and explain any discrepancies or unexpected findings. The conclusion should also suggest areas for further research, particularly if the results raise new questions or if there were limitations in the experiment. It's important to keep the conclusion concise and focused, avoiding the introduction of new information or unnecessary repetition.In conclusion, writing a science lab report involves careful attention to detail, clear and concise writing, and a thorough understanding of the experiment conducted. Each section of the report serves a specific purpose and contributes to the overall understanding of the research. By following the tips and tricks discussed in this essay, one can enhance their lab report writing skills and contribute effectively to the scientific community.
Science Lab Essay Examples: Learn from the Best
The art of scientific writing is a skill that is honed over time, with practice and guidance. One of the most effective ways to learn this skill is by studying science lab essay examples. These examples serve as practical guides, providing insights into the structure, language, and presentation of scientific findings. This essay explores the importance of science lab essay examples, how to learn from them, and where to find them. What are some examples of science lab essays?Science lab essays are academic papers that detail the process, findings, and implications of a laboratory experiment. Examples of such essays include "The Effect of Temperature on Enzyme Activity," "The Impact of Light Intensity on Photosynthesis," or "The Influence of pH on the Growth of Bacteria." These essays typically follow a structured format, starting with an introduction that outlines the purpose of the experiment, followed by a materials and methods section, results, discussion, and conclusion. They are characterized by their use of scientific language, clear and concise descriptions, and objective reporting of results. How can I learn from science lab essay examples?Learning from science lab essay examples involves more than just reading the content. It requires a critical analysis of the structure, language, and presentation of data. Pay attention to how the writer introduces the topic, presents the hypothesis, describes the experimental procedure, and discusses the results. Notice the use of scientific terminology and the way data is presented in tables, graphs, or diagrams. By studying these elements, you can gain insights into how to write a compelling and scientifically sound lab report. Why are science lab essay examples important for students?Science lab essay examples are crucial for students as they provide a practical guide on how to structure and write their own lab reports. They offer insights into the appropriate use of scientific language, the presentation of data, and the discussion of results. By studying these examples, students can understand the expectations for their own work, learn how to present their findings effectively, and develop their scientific writing skills. Furthermore, these examples can inspire students to explore different scientific concepts and develop their own experiments. Where can I find science lab essay examples?Science lab essay examples can be found in various places. Academic journals and textbooks often include lab reports as examples of how to present scientific findings. Online academic resources, such as Google Scholar, JSTOR, and PubMed, also provide access to a wealth of lab reports across various scientific disciplines. Additionally, many universities and colleges have online writing centers that provide examples of well-written lab reports. It's important to ensure that the examples you use are from reputable sources to ensure you're learning from the best. Who can benefit from studying science lab essay examples?Anyone involved in scientific research can benefit from studying science lab essay examples. This includes high school and college students taking science courses, graduate students conducting research, and even professional scientists. Studying these examples can help improve one's scientific writing skills, understand how to present data effectively, and learn how to structure a lab report properly. Furthermore, educators can use these examples as teaching tools to guide their students in writing their own lab reports.In conclusion, science lab essay examples are invaluable resources for anyone involved in scientific research. They provide a blueprint for structuring and writing a lab report, showcasing the appropriate use of scientific language and effective presentation of data. By studying these examples, students and researchers alike can improve their scientific writing skills, understand the expectations for their work, and gain inspiration for their own experiments. Whether you're a high school student just starting out in science, a graduate student conducting complex research, or a professional scientist, studying science lab essay examples can significantly enhance your scientific writing and communication skills.
How to Write a Compelling Science Lab Essay: From Introduction to Conclusion
Writing a compelling science lab essay requires a clear understanding of the experiment's purpose, a detailed account of the methodology, an objective presentation of the results, a thoughtful analysis of these results, and a concise conclusion that summarizes the findings and suggests future research directions. This essay will guide you through each of these components, providing you with the tools you need to write a compelling science lab essay. What are the key components of a compelling science lab essay?The key components of a compelling science lab essay include a clear and concise introduction, a detailed methodology section, a results section that presents the data collected, an analysis section that interprets the results, and a conclusion that summarizes the findings and suggests future research directions. The introduction should provide background information on the topic and state the purpose of the experiment. The methodology section should detail the procedures followed in the experiment, while the results section should present the data collected in a clear and organized manner. The analysis section should interpret these results in the context of the experiment's purpose, and the conclusion should summarize the findings and suggest areas for future research. How to structure a science lab essay effectively?An effective science lab essay should follow a logical structure that guides the reader through the experiment process. It should start with an introduction that provides background information and states the purpose of the experiment. This should be followed by a methodology section that details the procedures followed in the experiment. The next section should present the results of the experiment in a clear and organized manner. This should be followed by an analysis section that interprets the results in the context of the experiment's purpose. The essay should conclude with a summary of the findings and suggestions for future research. What is the role of the introduction in a science lab essay?The introduction in a science lab essay serves to provide the reader with background information on the topic and to state the purpose of the experiment. It sets the stage for the rest of the essay by providing context and explaining why the experiment is important. The introduction should be clear and concise, providing enough information for the reader to understand the topic but not so much that it overwhelms them. It should also include a thesis statement that clearly states the purpose of the experiment. How to present results in a science lab essay?The results section of a science lab essay should present the data collected during the experiment in a clear and organized manner. This can be done through the use of tables, graphs, and charts, which can help to visualize the data and make it easier for the reader to understand. The results should be presented objectively, without any interpretation or analysis. This section should simply state what was found during the experiment, leaving the interpretation for the analysis section. What should be included in the conclusion of a science lab essay?The conclusion of a science lab essay should summarize the findings of the experiment and suggest areas for future research. It should restate the purpose of the experiment and summarize the results, then interpret these results in the context of the experiment's purpose. The conclusion should also suggest areas for future research, indicating what further work could be done to expand on the findings of the experiment.In conclusion, writing a compelling science lab essay involves more than just presenting data. It requires a clear and concise introduction that provides background information and states the purpose of the experiment, a detailed methodology section that guides the reader through the experiment process, an objective results section that presents the data collected, a thoughtful analysis section that interprets these results, and a concise conclusion that summarizes the findings and suggests future research directions. By following these guidelines, you can write a science lab essay that is not only informative but also engaging and compelling.
Popular Essays
Common Interview Questions and How to Answer Them
Intelligent Transport System
Prognosis of Breast Cancer
How Electric Motors Work
What is President's Day
You And Me Forever
racial identity
Our America
Why is Communication Important in The Workplace
Organelles in Eukaryotic Cells
The Impact of False Recall on Learning and Memory
Beyond the Curtain: The Educational Value of Black Box Theatre at Saint Joseph's Academy
Global Perspectives on Inequality and Poverty: Lessons and Strategies for Change
The Evolution of Phenotypes: A Competitive Perspective
Beyond the Hype: A Scientific Review of Salvia Divinorum's Potential Risks and Benefits
How to Manage Your Time Effectively for School and Leisure
RFID Implementation Challenges and Successes at Metro Group: A Detailed Review
The American Dream in Crisis: Economic Inequality and the Erosion of Power
Physical Work and Job Satisfaction: A Case Study of Employee Perceptions
The Impact of COPE Methods on Student Performance: A Case Study Analysis
Socioeconomic Factors Influencing Childhood Obesity Rates: A Comprehensive Analysis
Hyperactivation of the Amygdala: Implications for Emotional Processing and Behavior
Top 10 Must-Visit Beaches on Oahu for Students on a Budget
Finding Inspiration in Illness: A Guide to Creative Writing
Organizational Culture and Its Role in Shaping Ethical Business Practices
The Importance of Ethical Practice in Social Care: A Personal Perspective
From Vacuuming to More: Unlocking the Potential of Roomba and Similar Robots
Investing in Human Potential: The Economic and Social Benefits of Self-Sufficiency Programs
From Sonnets to Free Verse: A Journey Through My Favorite Poetry Styles
International Laws and Conventions Governing Chemical Weapons: Focus on Mustard Gas
Active vs. Passive Immunity: Which One is Right for You?
Heart Failure Diagnosis: A Comprehensive Guide for Healthcare Professionals
Teaching Tolerance Through Literature: Lessons from Ramona's Experiences
The Successes of Public Schools: A Celebration of Achievement
From Babbling to Sentences: Understanding the Milestones of Language Development
Building a Writing Habit: The Importance of Consistent Creative Writing Logs
Best Restaurants in Lafayette-West Lafayette for a Purdue Game Day
Family Law Act 1975: A Case Study Approach to Understanding its Application
The Ultimate Guide to Mountain Biking in Montana's National Parks
Restorative Practices for Student Discipline: Moving Beyond Punishment and Towards Repair
The Hidden Costs of Homelessness: Economic Impact on U.S. Communities
Triage Protocols in Emergency Medicine: Balancing Resources and Patient Needs
The Heart's Electrical Symphony: A Guide to Cardiac Physiology
Crafting a Compelling Minor Case Essay: Tips and Strategies for Success
Case Studies in Evidence Law and Audit Program Implementation
The Power of Storytelling: Using Narrative Techniques to Enhance Your COM 155 Essay
Muhammad Ali's Inspirational Quotes: Words that Changed the World
The Impact of Tourism on Lesbos Island: Balancing Development and Sustainability
The Advantages of Military Experience in the Job Market
Data Analytics and Big Data: Unlocking Insights in the Information Age
*** Enter the $2,000 College Transitions No Essay Scholarship Contest ***
How to Write a Lab Report – with Example/Template
April 11, 2024
Perhaps you’re in the midst of your challenging AP chemistry class in high school, or perhaps college you’re enrolled in biology , chemistry , or physics at university. At some point, you will likely be asked to write a lab report. Sometimes, your teacher or professor will give you specific instructions for how to format and write your lab report, and if so, use that. In case you’re left to your own devices, here are some guidelines you might find useful. Continue reading for the main elements of a lab report, followed by a detailed description of the more writing-heavy parts (with a lab report example/lab report template). Lastly, we’ve included an outline that can help get you started.
What is a lab report?
A lab report is an overview of your experiment. Essentially, it explains what you did in the experiment and how it went. Most lab reports end up being 5-10 pages long (graphs or other images included), though the length depends on the experiment. Here are some brief explanations of the essential parts of a lab report:
Title : The title says, in the most straightforward way possible, what you did in the experiment. Often, the title looks something like, “Effects of ____ on _____.” Sometimes, a lab report also requires a title page, which includes your name (and the names of any lab partners), your instructor’s name, and the date of the experiment.
Abstract : This is a short description of key findings of the experiment so that a potential reader could get an idea of the experiment before even beginning.
Introduction : This is comprised of one or several paragraphs summarizing the purpose of the lab. The introduction usually includes the hypothesis, as well as some background information.
Lab Report Example (Continued)
Materials : Perhaps the simplest part of your lab report, this is where you list everything needed for the completion of your experiment.
Methods : This is where you describe your experimental procedure. The section provides necessary information for someone who would want to replicate your study. In paragraph form, write out your methods in chronological order, though avoid excessive detail.
Data : Here, you should document what happened in the experiment, step-by-step. This section often includes graphs and tables with data, as well as descriptions of patterns and trends. You do not need to interpret all of the data in this section, but you can describe trends or patterns, and state which findings are interesting and/or significant.
Discussion of results : This is the overview of your findings from the experiment, with an explanation of how they pertain to your hypothesis, as well as any anomalies or errors.
Conclusion : Your conclusion will sum up the results of your experiment, as well as their significance. Sometimes, conclusions also suggest future studies.
Sources : Often in APA style , you should list all texts that helped you with your experiment. Make sure to include course readings, outside sources, and other experiments that you may have used to design your own.
How to write the abstract
The abstract is the experiment stated “in a nutshell”: the procedure, results, and a few key words. The purpose of the academic abstract is to help a potential reader get an idea of the experiment so they can decide whether to read the full paper. So, make sure your abstract is as clear and direct as possible, and under 200 words (though word count varies).
When writing an abstract for a scientific lab report, we recommend covering the following points:
- Background : Why was this experiment conducted?
- Objectives : What problem is being addressed by this experiment?
- Methods : How was the study designed and conducted?
- Results : What results were found and what do they mean?
- Conclusion : Were the results expected? Is this problem better understood now than before? If so, how?
How to write the introduction
The introduction is another summary, of sorts, so it could be easy to confuse the introduction with the abstract. While the abstract tends to be around 200 words summarizing the entire study, the introduction can be longer if necessary, covering background information on the study, what you aim to accomplish, and your hypothesis. Unlike the abstract (or the conclusion), the introduction does not need to state the results of the experiment.
Here is a possible order with which you can organize your lab report introduction:
- Intro of the intro : Plainly state what your study is doing.
- Background : Provide a brief overview of the topic being studied. This could include key terms and definitions. This should not be an extensive literature review, but rather, a window into the most relevant topics a reader would need to understand in order to understand your research.
- Importance : Now, what are the gaps in existing research? Given the background you just provided, what questions do you still have that led you to conduct this experiment? Are you clarifying conflicting results? Are you undertaking a new area of research altogether?
- Prediction: The plants placed by the window will grow faster than plants placed in the dark corner.
- Hypothesis: Basil plants placed in direct sunlight for 2 hours per day grow at a higher rate than basil plants placed in direct sunlight for 30 minutes per day.
- How you test your hypothesis : This is an opportunity to briefly state how you go about your experiment, but this is not the time to get into specific details about your methods (save this for your results section). Keep this part down to one sentence, and voila! You have your introduction.
How to write a discussion section
Here, we’re skipping ahead to the next writing-heavy section, which will directly follow the numeric data of your experiment. The discussion includes any calculations and interpretations based on this data. In other words, it says, “Now that we have the data, why should we care?” This section asks, how does this data sit in relation to the hypothesis? Does it prove your hypothesis or disprove it? The discussion is also a good place to mention any mistakes that were made during the experiment, and ways you would improve the experiment if you were to repeat it. Like the other written sections, it should be as concise as possible.
Here is a list of points to cover in your lab report discussion:
- Weaker statement: These findings prove that basil plants grow more quickly in the sunlight.
- Stronger statement: These findings support the hypothesis that basil plants placed in direct sunlight grow at a higher rate than basil plants given less direct sunlight.
- Factors influencing results : This is also an opportunity to mention any anomalies, errors, or inconsistencies in your data. Perhaps when you tested the first round of basil plants, the days were sunnier than the others. Perhaps one of the basil pots broke mid-experiment so it needed to be replanted, which affected your results. If you were to repeat the study, how would you change it so that the results were more consistent?
- Implications : How do your results contribute to existing research? Here, refer back to the gaps in research that you mentioned in your introduction. Do these results fill these gaps as you hoped?
- Questions for future research : Based on this, how might your results contribute to future research? What are the next steps, or the next experiments on this topic? Make sure this does not become too broad—keep it to the scope of this project.
How to write a lab report conclusion
This is your opportunity to briefly remind the reader of your findings and finish strong. Your conclusion should be especially concise (avoid going into detail on findings or introducing new information).
Here are elements to include as you write your conclusion, in about 1-2 sentences each:
- Restate your goals : What was the main question of your experiment? Refer back to your introduction—similar language is okay.
- Restate your methods : In a sentence or so, how did you go about your experiment?
- Key findings : Briefly summarize your main results, but avoid going into detail.
- Limitations : What about your experiment was less-than-ideal, and how could you improve upon the experiment in future studies?
- Significance and future research : Why is your research important? What are the logical next-steps for studying this topic?
Template for beginning your lab report
Here is a compiled outline from the bullet points in these sections above, with some examples based on the (overly-simplistic) basil growth experiment. Hopefully this will be useful as you begin your lab report.
1) Title (ex: Effects of Sunlight on Basil Plant Growth )
2) Abstract (approx. 200 words)
- Background ( This experiment looks at… )
- Objectives ( It aims to contribute to research on…)
- Methods ( It does so through a process of…. )
- Results (Findings supported the hypothesis that… )
- Conclusion (These results contribute to a wider understanding about…)
3) Introduction (approx. 1-2 paragraphs)
- Intro ( This experiment looks at… )
- Background ( Past studies on basil plant growth and sunlight have found…)
- Importance ( This experiment will contribute to these past studies by…)
- Hypothesis ( Basil plants placed in direct sunlight for 2 hours per day grow at a higher rate than basil plants placed in direct sunlight for 30 minutes per day.)
- How you will test your hypothesis ( This hypothesis will be tested by a process of…)
4) Materials (list form) (ex: pots, soil, seeds, tables/stands, water, light source )
5) Methods (approx. 1-2 paragraphs) (ex: 10 basil plants were measured throughout a span of…)
6) Data (brief description and figures) (ex: These charts demonstrate a pattern that the basil plants placed in direct sunlight…)
7) Discussion (approx. 2-3 paragraphs)
- Support or reject hypothesis ( These findings support the hypothesis that basil plants placed in direct sunlight grow at a higher rate than basil plants given less direct sunlight.)
- Factors that influenced your results ( Outside factors that could have altered the results include…)
- Implications ( These results contribute to current research on basil plant growth and sunlight because…)
- Questions for further research ( Next steps for this research could include…)
- Restate your goals ( In summary, the goal of this experiment was to measure…)
- Restate your methods ( This hypothesis was tested by…)
- Key findings ( The findings supported the hypothesis because…)
- Limitations ( Although, certain elements were overlooked, including…)
- Significance and future research ( This experiment presents possibilities of future research contributions, such as…)
- Sources (approx. 1 page, usually in APA style)
Final thoughts – Lab Report Example
Hopefully, these descriptions have helped as you write your next lab report. Remember that different instructors may have different preferences for structure and format, so make sure to double-check when you receive your assignment. All in all, make sure to keep your scientific lab report concise, focused, honest, and organized. Good luck!
For more reading on coursework success, check out the following articles:
- How to Write the AP Lang Argument Essay (With Example)
- How to Write the AP Lang Rhetorical Analysis Essay (With Example)
- 49 Most Interesting Biology Research Topics
- 50 Best Environmental Science Research Topics
- High School Success
Sarah Mininsohn
With a BA from Wesleyan University and an MFA from the University of Illinois at Urbana-Champaign, Sarah is a writer, educator, and artist. She served as a graduate instructor at the University of Illinois, a tutor at St Peter’s School in Philadelphia, and an academic writing tutor and thesis mentor at Wesleyan’s Writing Workshop.
- 2-Year Colleges
- ADHD/LD/Autism/Executive Functioning
- Application Strategies
- Best Colleges by Major
- Best Colleges by State
- Big Picture
- Career & Personality Assessment
- College Essay
- College Search/Knowledge
- College Success
- Costs & Financial Aid
- Data Visualizations
- Dental School Admissions
- Extracurricular Activities
- General Knowledge
- Graduate School Admissions
- High Schools
- Homeschool Resources
- Law School Admissions
- Medical School Admissions
- Navigating the Admissions Process
- Online Learning
- Outdoor Adventure
- Private High School Spotlight
- Research Programs
- Summer Program Spotlight
- Summer Programs
- Teacher Tools
- Test Prep Provider Spotlight
“Innovative and invaluable…use this book as your college lifeline.”
— Lynn O'Shaughnessy
Nationally Recognized College Expert
$2,000 No Essay Scholarship
Presented by College Transitions
- Win $2,000 for college • 1 minute or less to enter • No essay required • Open to students and parents in the U.S.
Create your account today and easily enter all future sweepstakes!
Enter to Win $2,000 Today!

America's Lab Report: Investigations in High School Science (2006)
Chapter: 3 laboratory experiences and student learning, 3 laboratory experiences and student learning.
In this chapter, the committee first identifies and clarifies the learning goals of laboratory experiences and then discusses research evidence on attainment of those goals. The review of research evidence draws on three major strands of research: (1) cognitive research illuminating how students learn; (2) studies that examine laboratory experiences that stand alone, separate from the flow of classroom science instruction; and (3) research projects that sequence laboratory experiences with other forms of science instruction. 1 We propose the phrase “integrated instructional units” to describe these research and design projects that integrate laboratory experiences within a sequence of science instruction. In the following section of this chapter, we present design principles for laboratory experiences derived from our analysis of these multiple strands of research and suggest that laboratory experiences designed according to these principles are most likely to accomplish their learning goals. Next we consider the role of technology in supporting student learning from laboratory experiences. The chapter concludes with a summary.
GOALS FOR LABORATORY EXPERIENCES
Laboratories have been purported to promote a number of goals for students, most of which are also the goals of science education in general (Lunetta, 1998; Hofstein and Lunetta, 1982). The committee commissioned a paper to examine the definition and goals of laboratory experiences (Millar, 2004) and also considered research reviews on laboratory education that have identified and discussed learning goals (Anderson, 1976; Hofstein and Lunetta, 1982; Lazarowitz and Tamir, 1994; Shulman and Tamir, 1973). While these inventories of goals vary somewhat, a core set remains fairly consistent. Building on these commonly stated goals, the committee developed a comprehensive list of goals for or desired outcomes of laboratory experiences:
Enhancing mastery of subject matter . Laboratory experiences may enhance student understanding of specific scientific facts and concepts and of the way in which these facts and concepts are organized in the scientific disciplines.
Developing scientific reasoning . Laboratory experiences may promote a student’s ability to identify questions and concepts that guide scientific
investigations; to design and conduct scientific investigations; to develop and revise scientific explanations and models; to recognize and analyze alternative explanations and models; and to make and defend a scientific argument. Making a scientific argument includes such abilities as writing, reviewing information, using scientific language appropriately, constructing a reasoned argument, and responding to critical comments.
Understanding the complexity and ambiguity of empirical work . Interacting with the unconstrained environment of the material world in laboratory experiences may help students concretely understand the inherent complexity and ambiguity of natural phenomena. Laboratory experiences may help students learn to address the challenges inherent in directly observing and manipulating the material world, including troubleshooting equipment used to make observations, understanding measurement error, and interpreting and aggregating the resulting data.
Developing practical skills . In laboratory experiences, students may learn to use the tools and conventions of science. For example, they may develop skills in using scientific equipment correctly and safely, making observations, taking measurements, and carrying out well-defined scientific procedures.
Understanding of the nature of science . Laboratory experiences may help students to understand the values and assumptions inherent in the development and interpretation of scientific knowledge, such as the idea that science is a human endeavor that seeks to understand the material world and that scientific theories, models, and explanations change over time on the basis of new evidence.
Cultivating interest in science and interest in learning science . As a result of laboratory experiences that make science “come alive,” students may become interested in learning more about science and see it as relevant to everyday life.
Developing teamwork abilities . Laboratory experiences may also promote a student’s ability to collaborate effectively with others in carrying out complex tasks, to share the work of the task, to assume different roles at different times, and to contribute and respond to ideas.
Although most of these goals were derived from previous research on laboratory experiences and student learning, the committee identified the new goal of “understanding the complexity and ambiguity of empirical work” to reflect the unique nature of laboratory experiences. Students’ direct encounters with natural phenomena in laboratory science courses are inherently more ambiguous and messy than the representations of these phenomena in science lectures, textbooks, and mathematical formulas (Millar, 2004). The committee thinks that developing students’ ability to recognize this complexity and develop strategies for sorting through it is an essential
goal of laboratory experiences. Unlike the other goals, which coincide with the goals of science education more broadly and may be advanced through lectures, reading, or other forms of science instruction, laboratory experiences may be the only way to advance the goal of helping students understand the complexity and ambiguity of empirical work.
RECENT DEVELOPMENTS IN RESEARCH AND DESIGN OF LABORATORY EXPERIENCES
In reviewing evidence on the extent to which students may attain the goals of laboratory experiences listed above, the committee identified a recent shift in the research. Historically, laboratory experiences have been separate from the flow of classroom science instruction and often lacked clear learning goals. Because this approach remains common today, we refer to these isolated interactions with natural phenomena as “typical” laboratory experiences. 2 Reflecting this separation, researchers often engaged students in one or two experiments or other science activities and then conducted assessments to determine whether their understanding of the science concept underlying the activity had increased. Some studies directly compared measures of student learning following laboratory experiences with measures of student learning following lectures, discussions, videotapes, or other methods of science instruction in an effort to determine which modes of instruction were most effective.
Over the past 10 years, some researchers have shifted their focus. Assuming that the study of the natural world requires opportunities to directly encounter that world, investigators are integrating laboratory experiences and other forms of instruction into instructional sequences in order to help students progress toward science learning goals. These studies draw on principles of learning derived from the rapid growth in knowledge from cognitive research to address the question of how to design science instruction, including laboratory experiences, in order to support student learning.
Given the complexity of these teaching and learning sequences, the committee struggled with how best to describe them. Initially, the committee used the term “science curriculum units.” However, that term failed to convey the importance of integration in this approach to sequencing laboratory experiences with other forms of teaching and learning. The research reviewed by the committee indicated that these curricula not only integrate laboratory experiences in the flow of science instruction, but also integrate
student learning about both the concepts and processes of science. To reflect these aspects of the new approach, the committee settled on the term “integrated instructional units” in this report.
The following sections briefly describe principles of learning derived from recent research in the cognitive sciences and their application in design of integrated instructional units.
Principles of Learning Informing Integrated Instructional Units
Recent research and development of integrated instructional units that incorporate laboratory experiences are based on a large and growing body of cognitive research. This research has led to development of a coherent and multifaceted theory of learning that recognizes that prior knowledge, context, language, and social processes play critical roles in cognitive development and learning (National Research Council, 1999). Taking each of these factors into account, the National Research Council (NRC) report How People Learn identifies four critical principles that support effective learning environments (Glaser, 1994; National Research Council, 1999), and a more recent NRC report, How Students Learn , considers these principles as they relate specifically to science (National Research Council, 2005). These four principles are summarized below.
Learner-Centered Environments
The emerging integrated instructional units are designed to be learner-centered. This principle is based on research showing that effective instruction begins with what learners bring to the setting, including cultural practices and beliefs, as well as knowledge of academic content. Taking students’ preconceptions into account is particularly critical in science instruction. Students come to the classroom with conceptions of natural phenomena that are based on their everyday experiences in the world. Although these conceptions are often reasonable and can provide satisfactory everyday explanations to students, they do not always match scientific explanations and break down in ways that students often fail to notice. Teachers face the challenge of engaging with these intuitive ideas, some of which are more firmly rooted than others, in order to help students move toward a more scientific understanding. In this way, understanding scientific knowledge often requires a change in—not just an addition to—what students notice and understand about the world (National Research Council, 2005).
Knowledge-Centered Environments
The developing integrated instructional units are based on the principle that learning is enhanced when the environment is knowledge-centered. That is, the laboratory experiences and other instruction included in integrated instructional units are designed to help students learn with understanding, rather than simply acquiring sets of disconnected facts and skills (National Research Council, 1999).
In science, the body of knowledge with which students must engage includes accepted scientific ideas about natural phenomena as well as an understanding of what it means to “do science.” These two aspects of science are reflected in the goals of laboratory experiences, which include mastery of subject matter (accepted scientific ideas about phenomena) and several goals related to the processes of science (understanding the complexity of empirical work, development of scientific reasoning). Research on student thinking about science shows a progression of ideas about scientific knowledge and how it is justified. At the first stage, students perceive scientific knowledge as right or wrong. Later, students characterize discrepant ideas and evidence as “mere opinion.” Eventually, students recognize scientific knowledge as being justified by evidence derived through rigorous research. Several studies have shown that a large proportion of high school students are at the first stage in their views of scientific knowledge (National Research Council, 2005).
Knowledge-centered environments encourage students to reflect on their own learning progress (metacognition). Learning is facilitated when individuals identify, monitor, and regulate their own thinking and learning. To be effective problem solvers and learners, students need to determine what they already know and what else they need to know in any given situation, including when things are not going as expected. For example, students with better developed metacognitive strategies will abandon an unproductive problem-solving strategy very quickly and substitute a more productive one, whereas students with less effective metacognitive skills will continue to use the same strategy long after it has failed to produce results (Gobert and Clement, 1999). The basic metacognitive strategies include: (1) connecting new information to former knowledge, (2) selecting thinking strategies deliberately, and (3) monitoring one’s progress during problem solving.
A final aspect of knowledge-centered learning, which may be particularly relevant to integrated instructional units, is that the practices and activities in which people engage while learning shape what they learn. Transfer (the ability to apply learning in varying situations) is made possible to the extent that knowledge and learning are grounded in multiple contexts. Transfer is more difficult when a concept is taught in a limited set of contexts or through a limited set of activities. By encountering the same concept at work in multiple contexts (such as in laboratory experiences and in discussion),
students can develop a deeper understanding of the concept and how it can be used as well as the ability to transfer what has been learned in one context to others (Bransford and Schwartz, 2001).

Assessment to Support Learning
Another important principle of learning that has informed development of integrated instructional units is that assessment can be used to support learning. Cognitive research has shown that feedback is fundamental to learning, but feedback opportunities are scarce in most classrooms. This research indicates that formative assessments provide students with opportunities to revise and improve the quality of their thinking while also making their thinking apparent to teachers, who can then plan instruction accordingly. Assessments must reflect the learning goals of the learning environment. If the goal is to enhance understanding and the applicability of knowledge, it is not sufficient to provide assessments that focus primarily on memory for facts and formulas. The Thinkertools science instructional unit discussed in the following section incorporates this principle, including formative self-assessment tools that help students advance toward several of the goals of laboratory experiences.
Community-Centered Environments
Research has shown that learning is enhanced in a community setting, when students and teachers share norms that value knowledge and participation (see Cobb et al., 2001). Such norms increase people’s opportunities and motivation to interact, receive feedback, and learn. Learning is enhanced when students have multiple opportunities to articulate their ideas to peers and to hear and discuss others’ ideas. A community-centered classroom environment may not be organized in traditional ways. For example, in science classrooms, the teacher is often the sole authority and arbiter of scientific knowledge, placing students in a relatively passive role (Lemke, 1990). Such an organization may promote students’ view that scientific knowledge is a collection of facts about the world, authorized by expert scientists and irrelevant to students’ own experience. The instructional units discussed below have attempted to restructure the social organization of the classroom and encourage students and the teacher to interact and learn from each other.
Design of Integrated Instructional Units
The learning principles outlined above have begun to inform design of integrated instructional units that include laboratory experiences with other types of science learning activities. These integrated instructional units were
developed through research programs that tightly couple research, design, and implementation in an iterative process. The research programs are beginning to document the details of student learning, development, and interaction when students are given systematic support—or scaffolding—in carefully structured social and cognitive activities. Scaffolding helps to guide students’ thinking, so that they can gradually take on more autonomy in carrying out various parts of the activities. Emerging research on these integrated instructional units provides guidance about how to design effective learning environments for real-world educational settings (see Linn, Davis, and Bell, 2004a; Cobb et al., 2003; Design-Based Research Collective, 2003).
Integrated instructional units interweave laboratory experiences with other types of science learning activities, including lectures, reading, and discussion. Students are engaged in framing research questions, designing and executing experiments, gathering and analyzing data, and constructing arguments and conclusions as they carry out investigations. Diagnostic, formative assessments are embedded into the instructional sequences and can be used to gauge student’s developing understanding and to promote their self-reflection on their thinking.
With respect to laboratory experiences, these instructional units share two key features. The first is that specific laboratory experiences are carefully selected on the basis of research-based ideas of what students are likely to learn from them. For example, any particular laboratory activity is likely to contribute to learning only if it engages students’ current thinking about the target phenomena and is likely to make them critically evaluate their ideas in relation to what they see during the activity. The second is that laboratory experiences are explicitly linked to and integrated with other learning activities in the unit. The assumption behind this second feature is that just because students do a laboratory activity, they may not necessarily understand what they have done. Nascent research on integrated instructional units suggests that both framing a particular laboratory experience ahead of time and following it with activities that help students make sense of the experience are crucial in using a laboratory experience to support science learning. This “integration” approach draws on earlier research showing that intervention and negotiation with an authority, usually a teacher, was essential to help students make meaning out of their laboratory activities (Driver, 1995).
Examples of Integrated Instructional Units
Scaling up chemistry that applies.
Chemistry That Applies (CTA) is a 6-8 week integrated instructional unit designed to help students in grades 8-10 understand the law of conservation
of matter. Created by researchers at the Michigan Department of Education (Blakeslee et al., 1993), this instructional unit was one of only a few curricula that were highly rated by American Assocation for the Advancement of Science Project 2061 in its study of middle school science curricula (Kesidou and Roseman, 2002). Student groups explore four chemical reactions—burning, rusting, the decomposition of water, and the volcanic reaction of baking soda and vinegar. They cause these reactions to happen, obtain and record data in individual notebooks, analyze the data, and use evidence-based arguments to explain the data.
The instructional unit engages the students in a carefully structured sequence of hands-on laboratory investigations interwoven with other forms of instruction (Lynch, 2004). Student understanding is “pressed” through many experiences with the reactions and by group and individual pressures to make meaning of these reactions. For example, video transcripts indicate that students engaged in “science talk” during teacher demonstrations and during student experiments.
Researchers at George Washington University, in a partnership with Montgomery County public schools in Maryland, are currently conducting a five-year study of the feasibility of scaling up effective integrated instructional units, including CTA (Lynch, Kuipers, Pyke, and Szesze, in press). In 2001-2002, CTA was implemented in five highly diverse middle schools that were matched with five comparison schools using traditional curriculum materials in a quasi-experimental research design. All 8th graders in the five CTA schools, a total of about 1,500 students, participated in the CTA curriculum, while all 8th graders in the matched schools used the science curriculum materials normally available. Students were given pre- and posttests.
In 2002-2003, the study was replicated in the same five pairs of schools. In both years, students who participated in the CTA curriculum scored significantly higher than comparison students on a posttest. Average scores of students who participated in the CTA curriculum showed higher levels of fluency with the concept of conservation of matter (Lynch, 2004). However, because the concept is so difficult, most students in both the treatment and control group still have misconceptions, and few have a flexible, fully scientific understanding of the conservation of matter. All subgroups of students who were engaged in the CTA curriculum—including low-income students (eligible for free and reduced-price meals), black and Hispanic students, English language learners, and students eligible for special educational services—scored significantly higher than students in the control group on the posttest (Lynch and O’Donnell, 2005). The effect sizes were largest among three subgroups considered at risk for low science achievement, including Hispanic students, low-income students, and English language learners.
Based on these encouraging results, CTA was scaled up to include about 6,000 8th graders in 20 schools in 2003-2004 and 12,000 8th graders in 37 schools in 2004-2005 (Lynch and O’Donnell, 2005).
ThinkerTools
The ThinkerTools instructional unit is a sequence of laboratory experiences and other learning activities that, in its initial version, yielded substantial gains in students’ understanding of Newton’s laws of motion (White, 1993). Building on these positive results, ThinkerTools was expanded to focus not only on mastery of these laws of motion but also on scientific reasoning and understanding of the nature of science (White and Frederiksen, 1998). In the 10-week unit, students were guided to reflect on their own thinking and learning while they carry out a series of investigations. The integrated instructional unit was designed to help them learn about science processes as well as about the subject of force and motion. The instructional unit supports students as they formulate hypotheses, conduct empirical investigations, work with conceptually analogous computer simulations, and refine a conceptual model for the phenomena. Across the series of investigations, the integrated instructional unit introduces increasingly complex concepts. Formative assessments are integrated throughout the instructional sequence in ways that allow students to self-assess and reflect on core aspects of inquiry and epistemological dimensions of learning.
Researchers investigated the impact of Thinker Tools in 12 7th, 8th, and 9th grade classrooms with 3 teachers and 343 students. The researchers evaluated students’ developing understanding of scientific investigations using a pre-post inquiry test. In this assessment, students were engaged in a thought experiment that asked them to conceptualize, design, and think through a hypothetical research study. Gains in scores for students in the reflective self-assessment classes and control classrooms were compared. Results were also broken out by students categorized as high and low achieving, based on performance on a standardized test conducted before the intervention. Students in the reflective self-assessment classes exhibited greater gains on a test of investigative skills. This was especially true for low-achieving students. The researchers further analyzed specific components of the associated scientific processes—formulation of hypotheses, designing an experiment, predicting results, drawing conclusions from made-up results, and relating those conclusions back to the original hypotheses. Students in the reflective-self-assessment classes did better on all of these components than those in control classrooms, especially on the more difficult components (drawing conclusions and relating them to the original hypotheses).
Computer as Learning Partner
Beginning in 1980, a large group of technologists, classroom teachers, and education researchers developed the Computer as Learning Partner (CLP)
integrated instructional unit. Over 10 years, the team developed and tested eight versions of a 12-week unit on thermodynamics. Each year, a cohort of about 300 8th grade students participated in a sequence of teaching and learning activities focused primarily on a specific learning goal—enhancing students’ understanding of the difference between heat and temperature (Linn, 1997). The project engaged students in a sequence of laboratory experiences supported by computers, discussions, and other forms of science instruction. For example, computer images and words prompted students to make predictions about heat and conductivity and perform experiments using temperature-sensitive probes to confirm or refute their predictions. Students were given tasks related to scientific phenomena affecting their daily lives—such as how to keep a drink cold for lunch or selecting appropriate clothing for hiking in the mountains—as a way to motivate their interest and curiosity. Teachers play an important role in carrying out the curriculum, asking students to critique their own and each others’ investigations and encouraging them to reflect on their own thinking.
Over 10 years of study and revision, the integrated instructional unit proved increasingly effective in achieving its stated learning goals. Before the sequenced instruction was introduced, only 3 percent of middle school students could adequately explain the difference between heat and temperature. Eight versions later, about half of the students participating in CLP could explain this difference, representing a 400 percent increase in achievement. In addition, nearly 100 percent of students who participated in the final version of the instructional unit demonstrated understanding of conductors (Linn and Songer, 1991). By comparison, only 25 percent of a group of undergraduate chemistry students at the University of California at Berkeley could adequately explain the difference between heat and temperature. A longitudinal study comparing high school seniors who participated in the thermodynamics unit in middle school with seniors who had received more traditional middle school science instruction found a 50 percent improvement in CLP students’ performance in distinguishing between heat and temperature (Linn and Hsi, 2000)
Participating in the CLP instructional unit also increased students’ interest in science. Longitudinal studies of CLP participants revealed that, among those who went on to take high school physics, over 90 percent thought science was relevant to their lives. And 60 percent could provide examples of scientific phenomena in their daily lives. By comparison, only 60 percent of high school physics students who had not participated in the unit during middle school thought science was relevant to their lives, and only 30 percent could give examples in their daily lives (Linn and Hsi, 2000).
EFFECTIVENESS OF LABORATORY EXPERIENCES
Description of the literature review.
The committee’s review of the literature on the effectiveness of laboratory experiences considered studies of typical laboratory experiences and emerging research focusing on integrated instructional units. In reviewing both bodies of research, we aim to specify how laboratory experiences can further each of the science learning goals outlined at the beginning of this chapter.
Limitations of the Research
Our review was complicated by weaknesses in the earlier research on typical laboratory experiences, isolated from the stream of instruction (Hofstein and Lunetta, 1982). First, the investigators do not agree on a precise definition of the “laboratory” experiences under study. Second, many studies were weak in the selection and control of variables. Investigators failed to examine or report important variables relating to student abilities and attitudes. For example, they failed to note students’ prior laboratory experiences. They also did not give enough attention to extraneous factors that might affect student outcomes, such as instruction outside the laboratory. Third, the studies of typical laboratory experiences usually involved a small group of students with little diversity, making it difficult to generalize the results to the large, diverse population of U.S. high schools today. Fourth, investigators did not give enough attention to the adequacy of the instruments used to measure student outcomes. As an example, paper and pencil tests that focus on testing mastery of subject matter, the most frequently used assessment, do not capture student attainment of all of the goals we have identified. Such tests are not able to measure student progress toward goals that may be unique to laboratory experiences, such as developing scientific reasoning, understanding the complexity and ambiguity of empirical work, and development of practical skills.
Finally, most of the available research on typical laboratory experiences does not fully describe these activities. Few studies have examined teacher behavior, the classroom learning environment, or variables identifying teacher-student interaction. In addition, few recent studies have focused on laboratory manuals—both what is in them and how they are used. Research on the intended design of laboratory experiences, their implementation, and whether the implementation resembles the initial design would provide the understanding needed to guide improvements in laboratory instruction. However, only a few studies of typical laboratory experiences have measured the effectiveness of particular laboratory experiences in terms of both the extent
to which their activities match those that the teacher intended and the extent to which the students’ learning matches the learning objectives of the activity (Tiberghien, Veillard, Le Marchal, Buty, and Millar, 2000).
We also found weaknesses in the evolving research on integrated instructional units. First, these new units tend to be hothouse projects; researchers work intensively with teachers to construct atypical learning environments. While some have been developed and studied over a number of years and iterations, they usually involve relatively small samples of students. Only now are some of these efforts expanding to a scale that will allow robust generalizations about their value and how best to implement them. Second, these integrated instructional units have not been designed specifically to contrast some version of laboratory or practical experience with a lack of such experience. Rather, they assume that educational interventions are complex, systemic “packages” (Salomon, 1996) involving many interactions that may influence specific outcomes, and that science learning requires some opportunities for direct engagement with natural phenomena. Researchers commonly aim to document the complex interactions between and among students, teachers, laboratory materials, and equipment in an effort to develop profiles of successful interventions (Cobb et al., 2003; Collins, Joseph, and Bielaczyc, 2004; Design-Based Research Collective, 2003). These newer studies focus on how to sequence laboratory experiences and other forms of science instruction to support students’ science learning.
Scope of the Literature Search
A final note on the review of research: the scope of our study did not allow for an in-depth review of all of the individual studies of laboratory education conducted over the past 30 years. Fortunately, three major reviews of the literature from the 1970s, 1980s, and 1990s are available (Lazarowitz and Tamir, 1994; Lunetta, 1998; Hofstein and Lunetta, 2004). The committee relied on these reviews in our analysis of studies published before 1994. To identify studies published between 1994 and 2004, the committee searched electronic databases.
To supplement the database search, the committee commissioned three experts to review the nascent body of research on integrated instructional units (Bell, 2005; Duschl, 2004; Millar, 2004). We also invited researchers who are currently developing, revising, and studying the effectiveness of integrated instructional units to present their findings at committee meetings (Linn, 2004; Lynch, 2004).
All of these activities yielded few studies that focused on the high school level and were conducted in the United States. For this reason, the committee expanded the range of the literature considered to include some studies targeted at middle school and some international studies. We included stud-
ies at the elementary through postsecondary levels as well as studies of teachers’ learning in our analysis. In drawing conclusions from studies that were not conducted at the high school level, the committee took into consideration the extent to which laboratory experiences in high school differ from those in elementary and postsecondary education. Developmental differences among students, the organizational structure of schools, and the preparation of teachers are a few of the many factors that vary by school level and that the committee considered in making inferences from the available research. Similarly, when deliberating on studies conducted outside the United States, we considered differences in the science curriculum, the organization of schools, and other factors that might influence the outcomes of laboratory education.
Mastery of Subject Matter
Evidence from research on typical laboratory experiences.
Claims that typical laboratory experiences help students master science content rest largely on the argument that opportunities to directly interact with, observe, and manipulate materials will help students to better grasp difficult scientific concepts. It is believed that these experiences will force students to confront their misunderstandings about phenomena and shift toward more scientific understanding.
Despite these claims, there is almost no direct evidence that typical laboratory experiences that are isolated from the flow of science instruction are particularly valuable for learning specific scientific content (Hofstein and Lunetta, 1982, 2004; Lazarowitz and Tamir, 1994). White (1996) points out that many major reviews of science education from the 1960s and 1970s indicate that laboratory work does little to improve understanding of science content as measured by paper and pencil tests, and later studies from the 1980s and early 1990s do not challenge this view. Other studies indicate that typical laboratory experiences are no more effective in helping students master science subject matter than demonstrations in high school biology (Coulter, 1966), demonstration and discussion (Yager, Engen, and Snider, 1969), and viewing filmed experiments in chemistry (Ben-Zvi, Hofstein, Kempa, and Samuel, 1976). In contrast to most of the research, a single comparative study (Freedman, 2002) found that students who received regular laboratory instruction over the course of a school year performed better on a test of physical science knowledge than a control group of students who took a similar physical science course without laboratory activities.
Clearly, most of the evidence does not support the argument that typical laboratory experiences lead to improved learning of science content. More specifically, concrete experiences with phenomena alone do not appear to
force students to confront their misunderstandings and reevaluate their own assumptions. For example, VandenBerg, Katu, and Lunetta (1994) reported, on the basis of clinical studies with individual students, that hands-on activities with introductory electricity materials facilitated students’ understanding of the relationships among circuit elements and variables. The carefully selected practical activities created conceptual conflict in students’ minds—a first step toward changing their naïve ideas about electricity. However, the students remained unable to develop a fully scientific mental model of a circuit system. The authors suggested that greater engagement with conceptual organizers, such as analogies and concept maps, could have helped students develop more scientific understandings of basic electricity. Several researchers, including Dupin and Joshua (1987), have reported similar findings. Studies indicate that students often hold beliefs so intensely that even their observations in the laboratory are strongly influenced by those beliefs (Champagne, Gunstone, and Klopfer, 1985, cited in Lunetta, 1998; Linn, 1997). Students tend to adjust their observations to fit their current beliefs rather than change their beliefs in the face of conflicting observations.
Evidence from Research on Integrated Instructional Units
Current integrated instructional units build on earlier studies that found integration of laboratory experiences with other instructional activities enhanced mastery of subject matter (Dupin and Joshua, 1987; White and Gunstone, 1992, cited in Lunetta, 1998). A recent review of these and other studies concluded (Hofstein and Lunetta, 2004, p. 33):
When laboratory experiences are integrated with other metacognitive learning experiences such as “predict-observe-explain” demonstrations (White and Gunstone, 1992) and when they incorporate the manipulation of ideas instead of simply materials and procedures, they can promote the learning of science.
Integrated instructional units often focus on complex science topics that are difficult for students to understand. Their design is based on research on students’ intuitive conceptions of a science topic and how those conceptions differ from scientific conceptions. Students’ ideas often do not match the scientific understanding of a phenomenon and, as noted previously, these intuitive notions are resistant to change. For this reason, the sequenced units incorporate instructional activities specifically designed to confront intuitive conceptions and provide an environment in which students can construct normative conceptions. The role of laboratory experiences is to emphasize the discrepancies between students’ intuitive ideas about the topic and scientific ideas, as well as to support their construction of normative understanding. In order to help students link formal, scientific concepts to real
phenomena, these units include a sequence of experiences that will push them to question their intuitive and often inaccurate ideas.
Emerging studies indicate that exposure to these integrated instructional units leads to demonstrable gains in student mastery of a number of science topics in comparison to more traditional approaches. In physics, these subjects include Newtonian mechanics (Wells, Hestenes, and Swackhamer, 1995; White, 1993); thermodynamics (Songer and Linn, 1991); electricity (Shaffer and McDermott, 1992); optics (Bell and Linn, 2000; Reiner, Pea, and Shulman, 1995); and matter (Lehrer, Schauble, Strom, and Pligge, 2001; Smith, Maclin, Grosslight, and Davis, 1997; Snir, Smith, and Raz, 2003). Integrated instructional units in biology have enhanced student mastery of genetics (Hickey, Kindfield, Horwitz, and Christie, 2003) and natural selection (Reiser et al., 2001). A chemistry unit has led to gains in student understanding of stoichiometry (Lynch, 2004). Many, but not all, of these instructional units combine computer-based simulations of the phenomena under study with direct interactions with these phenomena. The role of technology in providing laboratory experiences is described later in this chapter.
Developing Scientific Reasoning
While philosophers of science now agree that there is no single scientific method, they do agree that a number of reasoning skills are critical to research across the natural sciences. These reasoning skills include identifying questions and concepts that guide scientific investigations, designing and conducting scientific investigations, developing and revising scientific explanations and models, recognizing and analyzing alternative explanations and models, and making and defending a scientific argument. It is not necessarily the case that these skills are sequenced in a particular way or used in every scientific investigation. Instead, they are representative of the abilities that both scientists and students need to investigate the material world and make meaning out of those investigations. Research on children’s and adults’ scientific reasoning (see the review by Zimmerman, 2000) suggests that effective experimentation is difficult for most people and not learned without instructional support.
Early research on the development of investigative skills suggested that students could learn aspects of scientific reasoning through typical laboratory instruction in college-level physics (Reif and St. John, 1979, cited in Hofstein and Lunetta, 1982) and in high school and college biology (Raghubir, 1979; Wheatley, 1975, cited in Hofstein and Lunetta, 1982).
More recent research, however, suggests that high school and college science teachers often emphasize laboratory procedures, leaving little time for discussion of how to plan an investigation or interpret its results (Tobin, 1987; see Chapter 4 ). Taken as a whole, the evidence indicates that typical laboratory work promotes only a few aspects of the full process of scientific reasoning—making observations and organizing, communicating, and interpreting data gathered from these observations. Typical laboratory experiences appear to have little effect on more complex aspects of scientific reasoning, such as the capacity to formulate research questions, design experiments, draw conclusions from observational data, and make inferences (Klopfer, 1990, cited in White, 1996).
Research developing from studies of integrated instructional units indicates that laboratory experiences can play an important role in developing all aspects of scientific reasoning, including the more complex aspects, if the laboratory experiences are integrated with small group discussion, lectures, and other forms of science instruction. With carefully designed instruction that incorporates opportunities to conduct investigations and reflect on the results, students as young as 4th and 5th grade can develop sophisticated scientific thinking (Lehrer and Schauble, 2004; Metz, 2004). Kuhn and colleagues have shown that 5th graders can learn to experiment effectively, albeit in carefully controlled domains and with extended supervised practice (Kuhn, Schauble, and Garcia-Mila, 1992). Explicit instruction on the purposes of experiments appears necessary to help 6th grade students design them well (Schauble, Giaser, Duschl, Schulze, and John, 1995).These studies suggest that laboratory experiences must be carefully designed to support the development of scientific reasoning.
Given the difficulty most students have with reasoning scientifically, a number of instructional units have focused on this goal. Evidence from several studies indicates that, with the appropriate scaffolding provided in these units, students can successfully reason scientifically. They can learn to design experiments (Schauble et al., 1995; White and Frederiksen, 1998), make predictions (Friedler, Nachmias, and Linn, 1990), and interpret and explain data (Bell and Linn, 2000; Coleman, 1998; Hatano and Inagaki, 1991; Meyer and Woodruff, 1997; Millar, 1998; Rosebery, Warren, and Conant, 1992; Sandoval and Millwood, 2005). Engagement with these instructional units has been shown to improve students’ abilities to recognize discrepancies between predicted and observed outcomes (Friedler et al., 1990) and to design good experiments (Dunbar, 1993; Kuhn et al., 1992; Schauble et al., 1995; Schauble, Klopfer, and Raghavan, 1991).
Integrated instructional units seem especially beneficial in developing scientific reasoning skills among lower ability students (White and Frederiksen, 1998).
Recently, research has focused on an important element of scientific reasoning—the ability to construct scientific arguments. Developing, revising, and communicating scientific arguments is now recognized as a core scientific practice (Driver, Newton, and Osborne, 2000; Duschl and Osborne, 2002). Laboratory experiences play a key role in instructional units designed to enhance students’ argumentation abilities, because they provide both the impetus and the data for constructing scientific arguments. Such efforts have taken many forms. For example, researchers working with young Haitian-speaking students in Boston used the students’ own interests to develop scientific investigations. Students designed an investigation to determine which school drinking fountain had the best-tasting water. The students designed data collection protocols, collected and analyzed their data, and then argued about their findings (Rosebery et al., 1992). The Knowledge Integration Environment project asked middle school students to examine a common set of evidence to debate competing hypotheses about light propagation. Overall, most students learned the scientific concept (that light goes on forever), although those who made better arguments learned more than their peers (Bell and Linn, 2000). These and other examples (e.g., Sandoval and Millwood, 2005) show that students in middle and high school can learn to argue scientifically, by learning to coordinate theoretical claims with evidence taken from their laboratory investigations.
Developing Practical Skills
Science educators and researchers have long claimed that learning practical laboratory skills is one of the important goals for laboratory experiences and that such skills may be attainable only through such experiences (White, 1996; Woolnough, 1983). However, development of practical skills has been measured in research less frequently than mastery of subject matter or scientific reasoning. Such practical outcomes deserve more attention, especially for laboratory experiences that are a critical part of vocational or technical training in some high school programs. When a primary goal of a program or course is to train students for jobs in laboratory settings, they must have the opportunity to learn to use and read sophisticated instruments and carry out standardized experimental procedures. The critical questions about acquiring these skills through laboratory experiences may not be whether laboratory experiences help students learn them, but how the experiences can be constructed so as to be most effective in teaching such skills.
Some research indicates that typical laboratory experiences specifically focused on learning practical skills can help students progress toward other goals. For example, one study found that students were often deficient in the simple skills needed to successfully carry out typical laboratory activities, such as using instruments to make measurements and collect accurate data (Bryce and Robertson, 1985). Other studies indicate that helping students to develop relevant instrumentation skills in controlled “prelab” activities can reduce the probability that important measurements in a laboratory experience will be compromised due to students’ lack of expertise with the apparatus (Beasley, 1985; Singer, 1977). This research suggests that development of practical skills may increase the probability that students will achieve the intended results in laboratory experiences. Achieving the intended results of a laboratory activity is a necessary, though not sufficient, step toward effectiveness in helping students attain laboratory learning goals.
Some research on typical laboratory experiences indicates that girls handle laboratory equipment less frequently than boys, and that this tendency is associated with less interest in science and less self-confidence in science ability among girls (Jovanovic and King, 1998). It is possible that helping girls to develop instrumentation skills may help them to participate more actively and enhance their interest in learning science.
Studies of integrated instructional units have not examined the extent to which engagement with these units may enhance practical skills in using laboratory materials and equipment. This reflects an instructional emphasis on helping students to learn scientific ideas with real understanding and on developing their skills at investigating scientific phenomena, rather than on particular laboratory techniques, such as taking accurate measurements or manipulating equipment. There is no evidence to suggest that students do not learn practical skills through integrated instructional units, but to date researchers have not assessed such practical skills.
Understanding the Nature of Science
Throughout the past 50 years, studies of students’ epistemological beliefs about science consistently show that most of them have naïve views about the nature of scientific knowledge and how such knowledge is constructed and evaluated by scientists over time (Driver, Leach, Millar, and Scott, 1996; Lederman, 1992). The general public understanding of science is similarly inaccurate. Firsthand experience with science is often seen as a key way to advance students’ understanding of and appreciation for the conventions of science. Laboratory experiences are considered the primary mecha-
nism for providing firsthand experience and are therefore assumed to improve students’ understanding of the nature of science.
Research on student understanding of the nature of science provides little evidence of improvement with science instruction (Lederman, 1992; Driver et al., 1996). Although much of this research historically did not examine details of students’ laboratory experiences, it often included very large samples of science students and thus arguably captured typical laboratory experiences (research from the late 1950s through the 1980s is reviewed by Lederman, 1992). There appear to be developmental trends in students’ understanding of the relations between experimentation and theory-building. Younger students tend to believe that experiments yield direct answers to questions; during middle and high school, students shift to a vague notion of experiments being tests of ideas. Only a small number of students appear to leave high school with a notion of science as model-building and experimentation, in an ongoing process of testing and revision (Driver et al., 1996; Carey and Smith, 1993; Smith et al., 2000). The conclusion that most experts draw from these results is that the isolated nature and rote procedural focus of typical laboratory experiences inhibits students from developing robust conceptions of the nature of science. Consequently, some have argued that the nature of science must be an explicit target of instruction (Khishfe and Abd-El-Khalick, 2002; Lederman, Abd-El-Khalick, Bell, and Schwartz, 2002).
As discussed above, there is reasonable evidence that integrated instructional units help students to learn processes of scientific inquiry. However, such instructional units do not appear, on their own, to help students develop robust conceptions of the nature of science. One large-scale study of a widely available inquiry-oriented curriculum, in which integrated instructional units were an explicit feature, showed no significant change in students’ ideas about the nature of science after a year’s instruction (Meichtry, 1993). Students engaged in the BGuILE science instructional unit showed no gains in understanding the nature of science from their participation, and they seemed not even to see their experience in the unit as necessarily related to professional science (Sandoval and Morrison, 2003). These findings and others have led to the suggestion that the nature of science must be an explicit target of instruction (Lederman et al., 2002).
There is evidence from the ThinkerTools science instructional unit that by engaging in reflective self-assessment on their own scientific investiga-
tions, students gained a more sophisticated understanding of the nature of science than matched control classes who used the curriculum without the ongoing monitoring and evaluation of their own and others’ research (White and Frederiksen, 1998). Students who engaged in the reflective assessment process “acquire knowledge of the forms that scientific laws, models, and theories can take, and of how the development of scientific theories is related to empirical evidence” (White and Frederiksen, 1998, p. 92). Students who participated in the laboratory experiences and other learning activities in this unit using the reflective assessment process were less likely to “view scientific theories as immutable and never subject to revision” (White and Frederiksen, 1998, p. 72). Instead, they saw science as meaningful and explicable. The ThinkerTools findings support the idea that attention to nature of science issues should be an explicit part of integrated instructional units, although even with such attention it remains difficult to change students’ ideas (Khishfe and Abd-el-Khalick, 2002).
A survey of several integrated instructional units found that they seem to bridge the “language gap” between science in school and scientific practice (Duschl, 2004). The units give students “extended opportunities to explore the relationship between evidence and explanation,” helping them not only to develop new knowledge (mastery of subject matter), but also to evaluate claims of scientific knowledge, reflecting a deeper understanding of the nature of science (Duschl, 2004). The available research leaves open the question of whether or not these experiences help students to develop an explicit, reflective conceptual framework about the nature of science.
Cultivating Interest in Science and Interest in Learning Science
Studies of the effect of typical laboratory experiences on student interest are much rarer than those focusing on student achievement or other cognitive outcomes (Hofstein and Lunetta, 2004; White, 1996). The number of studies that address interest, attitudes, and other affective outcomes has decreased over the past decade, as researchers have focused almost exclusively on cognitive outcomes (Hofstein and Lunetta, 2004). Among the few studies available, the evidence is mixed. Some studies indicate that laboratory experiences lead to more positive attitudes (Renner, Abraham, and Birnie, 1985; Denny and Chennell, 1986). Other studies show no relation between laboratory experiences and affect (Ato and Wilkinson, 1986; Freedman, 2002), and still others report laboratory experiences turned students away from science (Holden, 1990; Shepardson and Pizzini, 1993).
There are, however, two apparent weaknesses in studies of interest and attitude (Hofstein and Lunetta, 1982). One is that researchers often do not carefully define interest and how it should be measured. Consequently, it is unclear if students simply reported liking laboratory activities more than other classroom activities, or if laboratory activities engendered more interest in science as a field, or in taking science courses, or something else. Similarly, studies may report increased positive attitudes toward science from students’ participation in laboratory experiences, without clear description of what attitudes were measured, how large the changes were, or whether changes persisted over time.
Student Perceptions of Typical Laboratory Experiences
Students’ perceptions of laboratory experiences may affect their interest and engagement in science, and some studies have examined those perceptions. Researchers have found that students often do not have clear ideas about the general or specific purposes of their work in typical science laboratory activities (Chang and Lederman, 1994) and that their understanding of the goals of lessons frequently do not match their teachers’ goals for the same lessons (Hodson, 1993; Osborne and Freyberg, 1985; Wilkenson and Ward, 1997). When students do not understand the goals of experiments or laboratory investigations, negative consequences for learning occur (Schauble et al., 1995). In fact, students often do not make important connections between the purpose of a typical laboratory investigation and the design of the experiments. They do not connect the experiment with what they have done earlier, and they do not note the discrepancies among their own concepts, the concepts of their peers, and those of the science community (Champagne et al., 1985; Eylon and Linn, 1988; Tasker, 1981). As White (1998) notes, “to many students, a ‘lab’ means manipulating equipment but not manipulating ideas.” Thus, in considering how laboratory experiences may contribute to students’ interest in science and to other learning goals, their perceptions of those experiences must be considered.
A series of studies using the Science Laboratory Environment Inventory (SLEI) has demonstrated links between students’ perceptions of laboratory experiences and student outcomes (Fraser, McRobbie, and Giddings, 1993; Fraser, Giddings, and McRobbie, 1995; Henderson, Fisher, and Fraser, 2000; Wong and Fraser, 1995). The SLEI, which has been validated cross-nationally, measures five dimensions of the laboratory environment: student cohesiveness, open-endedness, integration, rule clarity, and material environment (see Table 3-1 for a description of each scale). Using the SLEI, researchers have studied students’ perceptions of chemistry and biology laboratories in several countries, including the United States. All five dimensions appear to be positively related with student attitudes, although the
TABLE 3-1 Descriptive Information for the Science Laboratory Environment Inventory
relation of open-endedness with attitudes seems to vary with student population. In some populations, there is a negative relation to attitudes (Fraser et al., 1995) and to some cognitive outcomes (Henderson et al., 2000).
Research using the SLEI indicates that positive student attitudes are particularly strongly associated with cohesiveness (the extent to which students know, help, and are supportive of one another) and integration (the extent to which laboratory activities are integrated with nonlaboratory and theory classes) (Fraser et al.,1995; Wong and Fraser, 1995). Integration also shows a positive relation to students’ cognitive outcomes (Henderson et al., 2000; McRobbie and Fraser, 1993).
Students’ interest and attitudes have been measured less often than other goals of laboratory experiences in studies of integrated instructional units. When evidence is available, it suggests that students who participate in these units show greater interest in and more positive attitudes toward science. For example, in a study of ThinkerTools, completion of projects was used as a measure of student interest. The rate of submitting completed projects was higher for students in the ThinkerTools curriculum than for those in traditional instruction. This was true for all grades and ability levels (White and
Frederiksen, 1998). This study also found that students’ ongoing evaluation of their own and other students’ thinking increased motivation and self-confidence in their individual ability: students who participated in this ongoing evaluation not only turned in their final project reports more frequently, but they were also less likely to turn in reports that were identical to their research partner’s.
Participation in the ThinkerTools instructional unit appears to change students’ attitudes toward learning science. After completing the integrated instructional unit, fewer students indicated that “being good at science” was a result of inherited traits, and fewer agreed with the statement, “In general, boys tend to be naturally better at science than girls.” In addition, more students indicated that they preferred taking an active role in learning science, rather than simply being told the correct answer by the teacher (White and Frederiksen, 1998).
Researchers measured students’ engagement and motivation to master the complex topic of conservation of matter as part of the study of CTA. Students who participated in the CTA curriculum had higher levels of basic engagement (active participation in activities) and were more likely to focus on learning from the activities than students in the control group (Lynch et al., in press). This positive effect on engagement was especially strong among low-income students. The researchers speculate, “perhaps as a result of these changes in engagement and motivation, they learned more than if they had received the standard curriculum” (Lynch et al., in press).
Students who participated in CLP during middle school, when surveyed years later as high school seniors, were more likely to report that science is relevant to their lives than students who did not participate (Linn and Hsi, 2000). Further research is needed to illuminate which aspects of this instructional unit contribute to increased interest.
Developing Teamwork Abilities
Teamwork and collaboration appear in research on typical laboratory experiences in two ways. First, working in groups is seen as a way to enhance student learning, usually with reference to literature on cooperative learning or to the importance of providing opportunities for students to discuss their ideas. Second and more recently, attention has focused on the ability to work in groups as an outcome itself, with laboratory experiences seen as an ideal opportunity to develop these skills. The focus on teamwork as an outcome is usually linked to arguments that this is an essential skill for workers in the 21st century (Partnership for 21st Century Skills, 2003).
There is considerable evidence that collaborative work can help students learn, especially if students with high ability work with students with low ability (Webb and Palincsar, 1996). Collaboration seems especially helpful to lower ability students, but only when they work with more knowledgeable peers (Webb, Nemer, Chizhik, and Sugrue, 1998). Building on this research, integrated instructional units engage students in small-group collaboration as a way to encourage them to connect what they know (either from their own experiences or from prior instruction) to their laboratory experiences. Often, individual students disagree about prospective answers to the questions under investigation or the best way to approach them, and collaboration encourages students to articulate and explain their reasoning. A number of studies suggest that such collaborative investigation is effective in helping students to learn targeted scientific concepts (Coleman, 1998; Roschelle, 1992).
Extant research lacks specific assessment of the kinds of collaborative skills that might be learned by individual students through laboratory work. The assumption appears to be that if students collaborate and such collaborations are effective in supporting their conceptual learning, then they are probably learning collaborative skills, too.
Overall Effectiveness of Laboratory Experiences
The two bodies of research—the earlier research on typical laboratory experiences and the emerging research on integrated instructional units—yield different findings about the effectiveness of laboratory experiences in advancing the goals identified by the committee. In general, the nascent body of research on integrated instructional units offers the promise that laboratory experiences embedded in a larger stream of science instruction can be more effective in advancing these goals than are typical laboratory experiences (see Table 3-2 ).
Research on the effectiveness of typical laboratory experiences is methodologically weak and fragmented. The limited evidence available suggests that typical laboratory experiences, by themselves, are neither better nor worse than other methods of science instruction for helping students master science subject matter. However, more recent research indicates that integrated instructional units enhance students’ mastery of subject matter. Studies have demonstrated increases in student mastery of complex topics in physics, chemistry, and biology.
Typical laboratory experiences appear, based on the limited research available, to support some aspects of scientific reasoning; however, typical laboratory experiences alone are not sufficient for promoting more sophisticated scientific reasoning abilities, such as asking appropriate questions,
TABLE 3-2 Attainment of Educational Goals in Typical Laboratory Experiences and Integrated Instructional Units
designing experiments, and drawing inferences. Research on integrated instructional units provides evidence that the laboratory experiences and other forms of instruction they include promote development of several aspects of scientific reasoning, including the ability to ask appropriate questions, design experiments, and draw inferences.
The evidence indicates that typical laboratory experiences do little to increase students’ understanding of the nature of science. In contrast, some studies find that participating in integrated instructional units that are designed specifically with this goal in mind enhances understanding of the nature of science.
The available research suggests that typical laboratory experiences can play a role in enhancing students’ interest in science and in learning science. There is evidence that engagement with the laboratory experiences and other learning activities included in integrated instructional units enhances students’ interest in science and motivation to learn science.
In sum, the evolving research on integrated instructional units provides evidence of increases in students’ understanding of subject matter, development of scientific reasoning, and interest in science, compared with students who received more traditional forms of science instruction. Studies conducted to date also suggest that the units are effective in helping diverse groups of students attain these three learning goals. In contrast, the earlier research on typical laboratory experiences indicates that such typical laboratory experiences are neither better nor worse than other forms of science instruction in supporting student mastery of subject matter. Typical laboratory experiences appear to aid in development of only some aspects of scientific reasoning, and they appear to play a role in enhancing students’ interest in science and in learning science.
Due to a lack of available studies, the committee was unable to draw conclusions about the extent to which either typical laboratory experiences or laboratory experiences incorporated into integrated instructional units might advance the other goals identified at the beginning of this chapter—enhancing understanding of the complexity and ambiguity of empirical work, acquiring practical skills, and developing teamwork skills.
PRINCIPLES FOR DESIGN OF EFFECTIVE LABORATORY EXPERIENCES
The three bodies of research we have discussed—research on how people learn, research on typical laboratory experiences, and developing research on how students learn in integrated instructional units—yield information that promises to inform the design of more effective laboratory experiences.
The committee considers the emerging evidence sufficient to suggest four general principles that can help laboratory experiences achieve the goals outlined above. It must be stressed, however, that research to date has not described in much detail how these principles can be implemented nor how each principle might relate to each of the educational goals of laboratory experiences.
Clearly Communicated Purposes
Effective laboratory experiences have clear learning goals that guide the design of the experience. Ideally these goals are clearly communicated to students. Without a clear understanding of the purposes of a laboratory activity, students seem not to get much from it. Conversely, when the purposes of a laboratory activity are clearly communicated by teachers to students, then students seem capable of understanding them and carrying them out. There seems to be no compelling evidence that particular purposes are more understandable to students than others.
Sequenced into the Flow of Instruction
Effective laboratory experiences are thoughtfully sequenced into the flow of classroom science instruction. That is, they are explicitly linked to what has come before and what will come after. A common theme in reviews of laboratory practice in the United States is that laboratory experiences are presented to students as isolated events, unconnected with other aspects of classroom work. In contrast, integrated instructional units embed laboratory experiences with other activities that build on the laboratory experiences and push students to reflect on and better understand these experiences. The way a particular laboratory experience is integrated into a flow of activities should be guided by the goals of the overall sequence of instruction and of the particular laboratory experience.
Integrated Learning of Science Concepts and Processes
Research in the learning sciences (National Research Council, 1999, 2001) strongly implies that conceptual understanding, scientific reasoning, and practical skills are three capabilities that are not mutually exclusive. An educational program that partitions the teaching and learning of content from the teaching and learning of process is likely to be ineffective in helping students develop scientific reasoning skills and an understanding of science as a way of knowing. The research on integrated instructional units, all of which intertwine exploration of content with process through laboratory experiences, suggests that integration of content and process promotes attainment of several goals identified by the committee.
Ongoing Discussion and Reflection
Laboratory experiences are more likely to be effective when they focus students more on discussing the activities they have done during their laboratory experiences and reflecting on the meaning they can make from them, than on the laboratory activities themselves. Crucially, the focus of laboratory experiences and the surrounding instructional activities should not simply be on confirming presented ideas, but on developing explanations to make sense of patterns of data. Teaching strategies that encourage students to articulate their hypotheses about phenomena prior to experimentation and to then reflect on their ideas after experimentation are demonstrably more successful at supporting student attainment of the goals of mastery of subject matter, developing scientific reasoning, and increasing interest in science and science learning. At the same time, opportunities for ongoing discussion and reflection could potentially support students in developing teamwork skills.
COMPUTER TECHNOLOGIES AND LABORATORY EXPERIENCES
From scales to microscopes, technology in many forms plays an integral role in most high school laboratory experiences. Over the past two decades, personal computers have enabled the development of software specifically designed to help students learn science, and the Internet is an increasingly used tool for science learning and for science itself. This section examines the role that computer technologies now and may someday play in science learning in relation to laboratory experiences. Certain uses of computer technology can be seen as laboratory experiences themselves, according to the committee’s definition, to the extent that they allow students to interact with data drawn directly from the world. Other uses, less clearly laboratory experiences in themselves, provide certain features that aid science learning.
Computer Technologies Designed to Support Learning
Researchers and science educators have developed a number of software programs to support science learning in various ways. In this section, we summarize what we see as the main ways in which computer software can support science learning through providing or augmenting laboratory experiences.
Scaffolded Representations of Natural Phenomena
Perhaps the most common form of science education software are programs that enable students to interact with carefully crafted models of natural phenomena that are difficult to see and understand in the real world and have proven historically difficult for students to understand. Such programs are able to show conceptual interrelationships and connections between theoretical constructs and natural phenomena through the use of multiple, linked representations. For example, velocity can be linked to acceleration and position in ways that make the interrelationships understandable to students (Roschelle, Kaput, and Stroup, 2000). Chromosome genetics can be linked to changes in pedigrees and populations (Horowitz, 1996). Molecular chemical representations can be linked to chemical equations (Kozma, 2003).
In the ThinkerTools integrated instructional unit, abstracted representations of force and motion are provided for students to help them “see” such ideas as force, acceleration, and velocity in two dimensions (White, 1993; White and Frederiksen, 1998). Objects in the ThinkerTools microworld are represented as simple, uniformly sized “dots” to avoid students becoming confused about the idea of center of mass. Students use the microworld to solve various problems of motion in one or two dimensions, using the com-
puter keyboard to apply forces to dots to move them along specified paths. Part of the key to the software’s guidance is that it provides representations of forces and accelerations in which students can see change in response to their actions. A “dot trace,” for example, shows students how applying more force affects an object’s acceleration in a predictable way. A “vector cross” represents the individual components of forces applied in two dimensions in a way that helps students to link those forces to an object’s motion.
ThinkerTools is but one example of this type of interactive, representational software. Others have been developed to help students reason about motion (Roschelle, 1992), electricity (Gutwill, Fredericksen, and White, 1999), heat and temperature (Linn, Bell, and Hsi, 1998), genetics (Horwitz and Christie, 2000), and chemical reactions (Kozma, 2003), among others. These programs differ substantially from one another in how they represent their target phenomena, as there are substantial differences in the topics themselves and in the problems that students are known to have in understanding them. They share, however, a common approach to solving a similar set of problems—how to represent natural phenomena that are otherwise invisible in ways that help students make their own thinking explicit and guide them to normative scientific understanding.
When used as a supplement to hands-on laboratory experiences within integrated instructional units, these representations can support students’ conceptual change (e.g., Linn et al., 1998; White and Frederiksen, 1998). For example, students working through the ThinkerTools curriculum always experiment with objects in the real world before they work with the computer tools. The goals of the laboratory experiences are to provide some experience with the phenomena under study and some initial ideas that can then be explored on the computer.
Structured Simulations of Inaccessible Phenomena
Various types of simulations of phenomena represent another form of technology for science learning. These simulations allow students to explore and observe phenomena that are too expensive, infeasible, or even dangerous to interact with directly. Strictly speaking, a computer simulation is a program that simulates a particular phenomenon by running a computational model whose behavior can sometimes be changed by modifying input parameters to the model. For example, the GenScope program provides a set of linked representations of genetics and genetics phenomena that would otherwise be unavailable for study to most students (Horowitz and Christie, 2000). The software represents alleles, chromosomes, family pedigrees, and the like and links representations across levels in ways that enable students to trace inherited traits to specific genetic differences. The software uses an underlying Mendelian model of genetic inheritance to gov-
ern its behavior. As with the representations described above, embedding the use of the software in a carefully thought out curriculum sequence is crucial to supporting student learning (Hickey et al., 2000).
Another example in biology is the BGuILE project (Reiser et al., 2001). The investigators created a series of structured simulations allowing students to investigate problems of evolution by natural selection. In the Galapagos finch environment, for example, students can examine a carefully selected set of data from the island of Daphne Major to explain a historical case of natural selection. The BGuILE software does not, strictly speaking, consist of simulations because it does not “run” a model; from a student’s perspective, it simulates either Daphne Major or laboratory experiments on tuberculosis bacteria. Studies show that students can learn from the BGuILE environments when these environments are embedded in a well-organized curriculum (Sandoval and Reiser, 2004). They also show that successful implementation of such technology-supported curricula relies heavily on teachers (Tabak, 2004).
Structured Interactions with Complex Phenomena and Ideas
The examples discussed here share a crucial feature. The representations built into the software and the interface tools provided for learners are intended to help them learn in very specific ways. There are a great number of such tools that have been developed over the last quarter of a century. Many of them have been shown to produce impressive learning gains for students at the secondary level. Besides the ones mentioned, other tools are designed to structure specific scientific reasoning skills, such as prediction (Friedler et al., 1990) and the coordination of claims with evidence (Bell and Linn, 2000; Sandoval, 2003). Most of these efforts integrate students’ work on the computer with more direct laboratory experiences. Rather than thinking of these representations and simulations as a way to replace laboratory experiences, the most successful instructional sequences integrate them with a series of empirical laboratory investigations. These sequences of science instruction focus students’ attention on developing a shared interpretation of both the representations and the real laboratory experiences in small groups (Bell, 2005).
Computer Technologies Designed to Support Science
Advances in computer technologies have had a tremendous impact on how science is done and on what scientists can study. These changes are vast, and summarizing them is well beyond the scope of the committee’s charge. We found, however, that some innovations in scientific practice, especially uses of the Internet, are beginning to be applied to secondary
science education. With respect to future laboratory experiences, perhaps the most significant advance in many scientific fields is the aggregation of large, varied data sets into Internet-accessible databases. These databases are most commonly built for specific scientific communities, but some researchers are creating and studying new, learner-centered interfaces to allow access by teachers and schools. These research projects build on instructional design principles illuminated by the integrated instructional units discussed above.
One example is the Center for Embedded Networked Sensing (CENS), a National Science Foundation Science and Technology Center investigating the development and deployment of large-scale sensor networks embedded in physical environments. CENS is currently working on ecosystem monitoring, seismology, contaminant flow transport, and marine microbiology. As sensor networks come on line, making data available, science educators at the center are developing middle school curricula that include web-based tools to enable students to explore the same data sets that the professional scientists are exploring (Pea, Mills, and Takeuchi, 2004).
The interfaces professional scientists use to access such databases tend to be too inflexible and technical for students to use successfully (Bell, 2005). Bounding the space of possible data under consideration, supporting appropriate considerations of theory, and promoting understanding of the norms used in the visualization can help support students in developing a shared understanding of the data. With such support, students can develop both conceptual understanding and understanding of the data analysis process. Focusing students on causal explanation and argumentation based on the data analysis process can help them move from a descriptive, phenomenological view of science to one that considers theoretical issues of cause (Bell, 2005).
Further research and evaluation of the educational benefit of student interaction with large scientific databases are absolutely necessary. Still, the development of such efforts will certainly expand over time, and, as they change notions of what it means to conduct scientific experiments, they are also likely to change what it means to conduct a school laboratory.
The committee identified a number of science learning goals that have been attributed to laboratory experiences. Our review of the evidence on attainment of these goals revealed a recent shift in research, reflecting some movement in laboratory instruction. Historically, laboratory experiences have been disconnected from the flow of classroom science lessons. We refer to these separate laboratory experiences as typical laboratory experiences. Reflecting this separation, researchers often engaged students in one or two
experiments or other science activities and then conducted assessments to determine whether their understanding of the science concept underlying the activity had increased. Some studies compared the outcomes of these separate laboratory experiences with the outcomes of other forms of science instruction, such as lectures or discussions.
Over the past 10 years, researchers studying laboratory education have shifted their focus. Drawing on principles of learning derived from the cognitive sciences, they have asked how to sequence science instruction, including laboratory experiences, in order to support students’ science learning. We refer to these instructional sequences as “integrated instructional units.” Integrated instructional units connect laboratory experiences with other types of science learning activities, including lectures, reading, and discussion. Students are engaged in framing research questions, making observations, designing and executing experiments, gathering and analyzing data, and constructing scientific arguments and explanations.
The two bodies of research on typical laboratory experiences and integrated instructional units, including laboratory experiences, yield different findings about the effectiveness of laboratory experiences in advancing the science learning goals identified by the committee. The earlier research on typical laboratory experiences is weak and fragmented, making it difficult to draw precise conclusions. The weight of the evidence from research focused on the goals of developing scientific reasoning and enhancing student interest in science showed slight improvements in both after students participated in typical laboratory experiences. Research focused on the goal of student mastery of subject matter indicates that typical laboratory experiences are no more or less effective than other forms of science instruction (such as reading, lectures, or discussion).
Studies conducted to date on integrated instructional units indicate that the laboratory experiences, together with the other forms of instruction included in these units, show greater effectiveness for these same three goals (compared with students who received more traditional forms of science instruction): improving students’ mastery of subject matter, increasing development of scientific reasoning, and enhancing interest in science. Integrated instructional units also appear to be effective in helping diverse groups of students progress toward these three learning goals . A major limitation of the research on integrated instructional units, however, is that most of the units have been used in small numbers of science classrooms. Only a few studies have addressed the challenge of implementing—and studying the effectiveness of—integrated instructional units on a wide scale.
Due to a lack of available studies, the committee was unable to draw conclusions about the extent to which either typical laboratory experiences or integrated instructional units might advance the other goals identified at the beginning of this chapter—enhancing understanding of the complexity
and ambiguity of empirical work, acquiring practical skills, and developing teamwork skills. Further research is needed to clarify how laboratory experiences might be designed to promote attainment of these goals.
The committee considers the evidence sufficient to identify four general principles that can help laboratory experiences achieve the learning goals we have outlined. Laboratory experiences are more likely to achieve their intended learning goals if (1) they are designed with clear learning outcomes in mind, (2) they are thoughtfully sequenced into the flow of classroom science instruction, (3) they are designed to integrate learning of science content with learning about the processes of science, and (4) they incorporate ongoing student reflection and discussion.
Computer software and the Internet have enabled development of several tools that can support students’ science learning, including representations of complex phenomena, simulations, and student interaction with large scientific databases. Representations and simulations are most successful in supporting student learning when they are integrated in an instructional sequence that also includes laboratory experiences. Researchers are currently developing tools to support student interaction with—and learning from—large scientific databases.
Anderson, R.O. (1976). The experience of science: A new perspective for laboratory teaching . New York: Columbia University, Teachers College Press.
Ato, T., and Wilkinson, W. (1986). Relationships between the availability and use of science equipment and attitudes to both science and sources of scientific information in Benue State, Nigeria. Research in Science and Technological Education , 4 , 19-28.
Beasley, W.F. (1985). Improving student laboratory performance: How much practice makes perfect? Science Education , 69 , 567-576.
Bell, P. (2005). The school science laboratory: Considerations of learning, technology, and scientific practice . Paper prepared for the Committee on High School Science Laboratories: Role and Vision. Available at: http://www7.nationalacademies.org/bose/July_12-13_2004_High_School_Labs_Meeting_Agenda.html [accessed June 2005].
Bell, P., and Linn, M.C. (2000). Scientific arguments as learning artifacts: Designing for learning from the web with KIE. International Journal of Science Education , 22 (8), 797-817.
Ben-Zvi, R., Hofstein, A., Kampa, R.F, and Samuel, D. (1976). The effectiveness of filmed experiments in high school chemical education. Journal of Chemical Education , 53 , 518-520.
Blakeslee, T., Bronstein, L., Chapin, M., Hesbitt, D., Peek, Y., Thiele, E., and Vellanti, J. (1993). Chemistry that applies . Lansing: Michigan Department of Education. Available at: http://www.ed-web2.educ.msu.edu/CCMS/secmod/Cluster3.pdf [accessed Feb. 2005].
Bransford, J.D., and Schwartz, D.L. (2001). Rethinking transfer: A simple proposal with multiple implications. In A. Iran-Nejad, and P.D. Pearson (Eds.), Review of research in education (pp. 61-100). Washington, DC: American Educational Research Association.
Bryce, T.G.K., and Robertson, I.J. (1985). What can they do: A review of practical assessment in science. Studies in Science Education , 12 , 1-24.
Carey, S., and Smith, C. (1993). On understanding the nature of scientific knowledge. Educational Psychologist , 28 , 235-251.
Champagne, A.B., Gunstone, R.F., and Klopfer, L.E. (1985). Instructional consequences of students’ knowledge about physical phenomena. In L.H.T. West and A.L. Pines (Eds.), Cognitive structure and conceptual change (pp. 61-68). New York: Academic Press.
Chang, H.P., and Lederman, N.G. (1994). The effect of levels of co-operation within physical science laboratory groups on physical science achievement. Journal of Research in Science Teaching , 31 , 167-181.
Cobb, P., Confrey, J., diSessa, A., Lehrer, R., and Schauble, L. (2003). Design experiments in educational research. Educational Researcher , 32 (1), 9-13.
Cobb, P., Stephan, M., McClain, K., and Gavemeijer, K. (2001). Participating in classroom mathematical practices. Journal of the Learning Sciences , 10 , 113-164.
Coleman, E.B. (1998). Using explanatory knowledge during collaborative problem solving in science. Journal of the Learning Sciences , 7 (3, 4), 387-427.
Collins, A., Joseph, D., and Bielaczyc, K. (2004). Design research: Theoretical and methodological issues. Journal of the Learning Sciences , 13 (1), 15-42.
Coulter, J.C. (1966). The effectiveness of inductive laboratory demonstration and deductive laboratory in biology. Journal of Research in Science Teaching , 4 , 185-186.
Denny, M., and Chennell, F. (1986). Exploring pupils’ views and feelings about their school science practicals: Use of letter-writing and drawing exercises. Educational Studies , 12 , 73-86.
Design-Based Research Collective. (2003). Design-based research: An emerging paradigm for educational inquiry. Educational Researcher , 32 (1), 5-8.
Driver, R. (1995). Constructivist approaches to science teaching. In L.P. Steffe and J. Gale (Eds.), Constructivism in education (pp. 385-400). Hillsdale, NJ: Lawrence Erlbaum.
Driver, R., Leach, J., Millar, R., and Scott, P. (1996). Young people’s images of science . Buckingham, UK: Open University Press.
Driver, R., Newton, P., and Osborne, J. (2000). Establishing the norms of scientific argumentation in classrooms. Science Education , 84 , 287-312.
Dunbar, K. (1993). Concept discovery in a scientific domain. Cognitive Science , 17 , 397-434.
Dupin, J.J., and Joshua, S. (1987). Analogies and “modeling analogies” in teaching: Some examples in basic electricity. Science Education , 73 , 791-806.
Duschl, R.A. (2004). The HS lab experience: Reconsidering the role of evidence, explanation and the language of science . Paper prepared for the Committee on High School Science Laboratories: Role and Vision, July 12-13, National Research Council, Washington, DC. Available at: http://www7.nationalacademies.org/bose/July_12-13_2004_High_School_Labs_Meeting_Agenda.html [accessed July 2005].
Duschl, R.A., and Osborne, J. (2002). Supporting and promoting argumentation discourse in science education. Studies in Science Education , 38 , 39-72.
Eylon, B., and Linn, M.C. (1988). Learning and instruction: An examination of four research perspectives in science education. Review of Educational Research , 58 (3), 251-301.
Fraser, B.J., Giddings, G.J., and McRobbie, C.J. (1995). Evolution and validation of a personal form of an instrument for assessing science laboratory classroom environments. Journal of Research in Science Teaching , 32 , 399-422.
Fraser, B.J., McRobbie, C.J., and Giddings, G.J. (1993). Development and cross-national validation of a laboratory classroom environment instrument for senior high school science. Science Education , 77 , 1-24.
Freedman, M.P. (2002). The influence of laboratory instruction on science achievement and attitude toward science across gender differences. Journal of Women and Minorities in Science and Engineering , 8 , 191-200.
Friedler, Y., Nachmias, R., and Linn, M.C. (1990). Learning scientific reasoning skills in microcomputer-based laboratories. Journal of Research in Science Teaching , 27 (2), 173-192.
Glaser, R. (1994). Learning theory and instruction. In G. d’Ydewalle, P. Eelen, and P. Bertelson (Eds.), International perspectives on science, volume 2: The state of the art (pp. 341-357). Hove, England: Erlbaum.
Gobert, J., and Clement, J. (1999). The effects of student-generated diagrams versus student-generated summaries on conceptual understanding of spatial, causal, and dynamic knowledge in plate tectonics. Journal of Research in Science Teaching , 36 (1), 39-53.
Gutwill, J.P., Fredericksen, J.R., and White, B.Y. (1999). Making their own connections: Students’ understanding of multiple models in basic electricity. Cognition and Instruction , 17 (3), 249-282.
Hatano, G., and Inagaki, K. (1991). Sharing cognition through collective comprehension activity. In L.B. Resnick, J.M. Levine, and S.D. Teasley (Eds.), Perspectives on socially shared cognition (pp. 331-348). Washington, DC: American Psychological Association.
Henderson, D., Fisher, D., and Fraser, B. (2000). Interpersonal behavior, laboratory learning environments, and student outcomes in senior biology classes. Journal of Research in Science Teaching , 37 , 26-43.
Hickey, D.T., Kindfield, A.C.H., Horwitz, P., and Christie, M.A. (2000). Integrating instruction, assessment, and evaluation in a technology-based genetics environment: The GenScope follow-up study. In B.J. Fishman and S.F. O’Connor-Divelbiss (Eds.), Proceedings of the International Conference of the Learning Sciences (pp. 6-13). Mahwah, NJ: Lawrence Erlbaum.
Hickey, D.T., Kindfield, A.C., Horwitz, P., and Christie, M.A. (2003). Integrating curriculum, instruction, assessment, and evaluation in a technology-supported genetics environment. American Educational Research Journal , 40 (2), 495-538.
Hodson, D. (1993). Philosophic stance of secondary school science teachers, curriculum experiences, and children’s understanding of science: Some preliminary findings. Interchange , 24 , 41-52.
Hofstein, A., and Lunetta, V.N. (1982). The role of the laboratory in science teaching: Neglected aspects of research. Review of Educational Research , 52 (2), 201-217.
Hofstein, A., and Lunetta, V.N. (2004). The laboratory in science education: Foundations for the twenty-first century. Science Education , 88 , 28-54.
Holden, C. (1990). Animal rights activism threatens dissection. Science , 25 , 751.
Horowitz, P. (1996). Linking models to data: Hypermodels for science education. High School Journal , 79 (2), 148-156.
Horowitz, P., and Christie, M.A. (2000). Computer-based manipulatives for teaching scientific reasoning: An example. In M.J. Jacobson and R.B. Kozma (Eds.), Innovations in science and mathematics education: Advanced designs for technologies of learning (pp. 163-191). Mahwah, NJ: Lawrence Erlbaum.
Jovanovic, J., and King, S.S. (1998). Boys and girls in the performance-based science classroom: Who’s doing the performing? American Educational Research Journal , 35 (3), 477-496.
Kesidou, S., and Roseman, J. (2002). How well do middle school science programs measure up? Findings from Project 2061’s curriculum review. Journal of Research in Science Teaching , 39 (6), 522-549.
Khishfe, R., and Abd-El-Khalick, F. (2002). Influence of explicit and reflective versus implicit inquiry-oriented instruction on sixth graders’ views of nature of science. Journal of Research in Science Teaching , 39 (7), 551-578.
Klopfer, L.E. (1990). Learning scientific enquiry in the student laboratory. In E. Hegarty-Hazel (Ed.), The student laboratory and the science curriculum (pp. 95-118). London, England: Routledge.
Kozma, R.B. (2003). The material features of multiple representations and their cognitive and social affordances for science understanding. Learning and Instruction , 13 , 205-226.
Kuhn, D., Schauble, L., and Garcia-Mila, M. (1992). Cross-domain development of scientific reasoning. Cognition and Instruction , 9 (4), 285-327.
Lazarowitz, R., and Tamir, P. (1994). Research on using laboratory instruction in science. In D.L. Gabel (Ed.), Handbook of research on science teaching and learning (pp. 94-130). New York: Macmillan.
Lederman, N.G. (1992). Students’ and teachers’ conceptions of the nature of science: A review of the research. Journal of Research in Science Teaching , 29 (4), 331-359.
Lederman, N.G., Abd-El-Khalick, F., Bell, R.L., and Schwartz, R.S. (2002). Views of nature of science questionnaire: Toward valid and meaningful assessment of learners’ conceptions of nature of science. Journal of Research in Science Teaching , 39 (6), 497-521.
Lehrer, R., and Schauble, L. (2004). Scientific thinking and science literacy: Supporting development in learning contexts. In W. Damon, R. Lerner, K. Anne Renninger, and E. Sigel (Eds.), Handbook of child psychology, sixth edition, volume four: Child psychology in practice . Hoboken, NJ: John Wiley & Sons.
Lehrer, R., Schauble, L., Strom, D., and Pligge, M. (2001). Similarity of form and substance: Modeling material kind. In S.M. Carver and D. Klahr (Eds.), Cognition and instruction: Twenty-five years of progress . Mahwah, NJ: Lawrence Erlbaum.
Lemke, J. (1990). Talking science: Language, learning, and values . Norwood, NJ: Ablex.
Linn, M.C. (1997). The role of the laboratory in science learning. Elementary School Journal , 97 , 401-417.
Linn, M.C. (2004). High school science laboratories: How can technology contribute? Presentation to the Committee on High School Science Laboratories: Role and Vision. June. Available at: http://www7.nationalacademies.org/bose/June_3-4_2004_High_School_Labs_Meeting_Agenda.html [accessed April 2005].
Linn, M.C., Bell, P., and Hsi, S. (1998). Using the Internet to enhance student understanding of science: The knowledge integration environment. Interactive Learning Environments , 6 (1-2), 4-38.
Linn, M.C., Davis, E., and Bell, P. (2004a). Inquiry and technology. In M.C. Linn, E. Davis, and P. Bell, (Eds.), Internet environments for science education . Mahwah, NJ: Lawrence Erlbaum.
Linn, M.C., Davis, E., and Bell, P. (Eds.). (2004b). Internet environments for science education . Mahwah, NJ: Lawrence Erlbaum.
Linn, M.C., and Hsi, S. (2000). Computers, teachers, peers . Mahwah, NJ: Lawrence Erlbaum.
Linn, M.C., and Songer, B. (1991). Teaching thermodynamics to middle school children: What are appropriate cognitive demands? Journal of Research in Science Teaching , 28 (10), 885-918.
Lunetta, V.N. (1998). The school science laboratory. In B.J. Fraser and K.G. Tobin (Eds.), International handbook of science education (pp. 249-262). London, England: Kluwer Academic.
Lynch, S. (2004). What are the effects of highly rated, lab-based curriculum materials on diverse learners? Presentation to the Committee on High School Science Laboratories: Role and Vision. July 12. Available at: http://www7.nationalacademies.org/bose/July_12-13_2004_High_School_Labs_Meeting_Agenda.html [accessed Oct. 2004].
Lynch, S., Kuipers, J., Pyke, C., and Szesze, M. (In press). Examining the effects of a highly rated science curriculum unitinstructional unit on diverse populations: Results from a planning grant. Journal of Research in Science Teaching .
Lynch, S., and O’Donnell, C. (2005). The evolving definition, measurement, and conceptualization of fidelity of implementation in scale-up of highly rated science curriculum unitsintegrated instructional units in diverse middle schools . Paper presented at the annual meeting of the American Educational Research Association, April 7, Montreal, Canada.
McRobbie, C.J., and Fraser, B.J. (1993). Associations between student outcomes and psychosocial science environment. Journal of Educational Research , 87 , 78-85.
Meichtry, Y.J. (1993). The impact of science curricula on student views about the nature of science. Journal of Research in Science Teaching , 30 (5), 429-443.
Metz, K.E. (2004). Children’s understanding of scientific inquiry: Their conceptualization of uncertainty in investigations of their own design. Cognition and Instruction , 22 (2), 219-290.
Meyer, K., and Woodruff, E. (1997). Consensually driven explanation in science teaching. Science Education , 80 , 173-192.
Millar, R. (1998). Rhetoric and reality: What practical work in science education is really for. In J. Wellington (Ed.), Practical work in school science: Which way now? (pp. 16-31). London, England: Routledge.
Millar, R. (2004). The role of practical work in the teaching and learning of science . Paper prepared for the Committee on High School Science Laboratories: Role and Vision. Available at: http://www7.nationalacademies.org/bose/June3-4_2004_High_School_Labs_Meeting_Agenda.html [accessed April 2005].
National Research Council. (1999). How people learn: Brain, mind, experience, and school . Committee on Developments in the Science of Learning, J.D. Bransford, A.L. Brown, and R.R. Cocking (Eds.). Washington, DC: National Academy Press.
National Research Council. (2001). Eager to learn: Educating our preschoolers . Committee on Early Childhood Pedagogy. B.T. Bowman, M.S. Donovan, and M.S. Burns (Eds.). Commission on Behavioral and Social Sciences and Education. Washington, DC: National Academy Press.
National Research Council. (2005). Systems for state science assessment . Committee on Test Design for K-12 Science Achievement, M.R. Wilson and M.W. Bertenthal (Eds.). Board on Testing and Assessment, Center for Education. Division of Behavioral and Social Sciences and Education. Washington, DC: The National Academies Press.
Osborne, R., and Freyberg, P. (1985). Learning in science: The implications of children’s science . London, England: Heinemann.
Partnership for 21st Century Skills. (2003). Learning for the 21st century . Washington, DC: Author. Available at: http://www.21stcenturyskills.org/reports/learning.asp [accessed April 2005].
Pea, R., Mills, M., and Takeuchi, L. (Eds). (2004). Making SENS: Science education networks of sensors . Report from an OMRON-sponsored workshop of the Media-X Program at Stanford University, October 3. Stanford, CA: Stanford Center for Innovations in Learning. Available at:: http://www.makingsens.stanford.edu/index.html [accessed May 2005].
Raghubir, K.P. (1979). The laboratory investigative approach to science instruction. Journal of Research in Science Teaching , 16 , 13-18.
Reif, F., and St. John, M. (1979) Teaching physicists thinking skills in the laboratory. American Journal of Physics , 47 (11), 950-957.
Reiner, M., Pea, R.D., and Shulman, D.J. (1995). Impact of simulator-based instruction on diagramming in geometrical optics by introductory physics students. Journal of Science Education and Technology , 4 (3), 199-225.
Reiser, B.J., Tabak, I., Sandoval, W.A., Smith, B.K., Steinmuller, F., and Leone, A.J. (2001). BGuILE: Strategic and conceptual scaffolds for scientific inquiry in biology classrooms. In S.M. Carver and D. Klahr (Eds.), Cognition and instruction: Twenty-five years of progress (pp. 263-305). Mahwah, NJ: Lawrence Erlbaum.
Renner, J.W., Abraham, M.R., and Birnie, H.H. (1985). Secondary school students’ beliefs about the physics laboratory, Science Education , 69 , 649-63.
Roschelle, J. (1992). Learning by collaborating: Convergent conceptual change. Journal of the Learning Sciences , 2 (3), 235-276.
Roschelle, J., Kaput, J., and Stroup, W. (2000). SimCalc: Accelerating students’ engagement with the mathematics of change. In M.J. Jacobsen and R.B. Kozma (Eds). Learning the sciences of the 21st century: Research, design, and implementing advanced technology learning environments (pp. 47-75). Hillsdale, NJ: Lawrence Erlbaum.
Rosebery, A.S., Warren, B., and Conant, F.R. (1992). Appropriating scientific discourse: Findings from language minority classrooms. Journal of the Learning Sciences , 2 (1), 61-94.
Salomon, G. (1996). Studying novel learning environments as patterns of change. In S. Vosniadou, E. De Corte, R. Glaser, and H. Mandl (Eds.), International perspectives on the design of technology-supported learning environments (pp. 363-377). Mahwah, NJ: Lawrence Erlbaum.
Sandoval, W.A. (2003). Conceptual and epistemic aspects of students’ scientific explanations. Journal of the Learning Sciences , 12 (1), 5-51.
Sandoval, W.A., and Millwood, K.A. (2005). The quality of students’ use of evidence in written scientific explanations. Cognition and Instruction , 23 (1), 23-55.
Sandoval, W.A., and Morrison, K. (2003). High school students’ ideas about theories and theory change after a biological inquiry unit. Journal of Research in Science Teaching , 40 (4), 369-392.
Sandoval, W.A., and Reiser, B.J. (2004). Explanation-driven inquiry: Integrating conceptual and epistemic supports for science inquiry. Science Education , 88 , 345-372.
Schauble, L., Glaser, R., Duschl, R.A., Schulze, S., and John, J. (1995). Students’ understanding of the objectives and procedures of experimentation in the science classroom. Journal of the Learning Sciences , 4 (2), 131-166.
Schauble, L., Klopfer, L.E., and Raghavan, K. (1991). Students’ transition from an engineering model to a science model of experimentation. Journal of Research in Science Teaching , 28 (9), 859-882.
Shaffer, P.S., and McDermott, L.C. (1992). Research as a guide for curriculum development: An example from introductory electricity. Part II: Design of instructional strategies. American Journal of Physics , 60 (11), 1003-1013.
Shepardson, D.P., and Pizzini, E.L. (1993). A comparison of student perceptions of science activities within three instructional approaches. School Science and Mathematics , 93 , 127-131.
Shulman, L.S., and Tamir, P. (1973). Research on teaching in the natural sciences. In R.M.W. Travers (Ed.), Second handbook of research on teaching . Chicago: Rand-McNally.
Singer, R.N. (1977). To err or not to err: A question for the instruction of psychomotor skills. Review of Educational Research , 47 , 479-489.
Smith, C.L., Maclin, D., Grosslight, L., and Davis, H. (1997). Teaching for understanding: A study of students’ pre-instruction theories of matter and a comparison of the effectiveness of two approaches to teaching about matter and density. Cognition and Instruction , 15 , 317-394.
Smith, C.L., Maclin, D., Houghton, C., and Hennessey, M. (2000). Sixth-grade students’ epitemologies of science: The impact of school science experiences on epitemological development. Cognition and Instruction , 18 , 349-422.
Snir, J., Smith, C.L., and Raz, G. (2003). Linking phenomena with competing underlying models: A software tool for introducing students to the particulate model of matter. Science Education , 87 (6), 794-830.
Songer, N.B., and Linn, M.C. (1991). How do students’ views of science influence knowledge integration? Journal of Research in Science Teaching , 28 (9), 761-784.
Tabak, I. (2004). Synergy: a complement to emerging patterns of distributed scaffolding. Journal of the Learning Sciences , 13 (3), 305-335.
Tasker, R. (1981). Children’s views and classroom experiences. Australian Science Teachers’ Journal , 27 , 33-37.
Tiberghien, A., Veillard, L., Le Marechal, J.-F., Buty, C., and Millar, R. (2000). An analysis of labwork tasks used in science teaching at upper secondary school and university levels in several European countries. Science Education , 85 , 483-508.
Tobin, K. (1987). Forces which shape the implemented curriculum in high school science and mathematics. Teaching and Teacher Education , 3 (4), 287-298.
VandenBerg, E., Katu, N., and Lunetta, V.N. (1994). The role of “experiments” in conceptual change . Paper presented at the annual meeting of the National Association for Research in Science Teaching, Anaheim, CA.
Webb, N.M., Nemer, K.M., Chizhik, A.W., and Sugrue, B. (1998). Equity issues in collaborative group assessment: Group composition and performance. American Educational Research Journal , 35 (4), 607-652.
Webb, N.M., and Palincsar, A.S. (1996). Group processes in the classroom. In D.C. Berliner and R.C. Calfee (Eds.), Handbook of educational psychology (pp. 841-873). New York: Macmillan.
Wells, M., Hestenes, D., and Swackhamer, G. (1995). A modeling method for high school physics instruction. American Journal of Physics , 63 (7), 606-619.
Wheatley, J.H. (1975).Evaluating cognitive learning in the college science laboratory. Journal of Research in Science Teaching , 12 , 101-109.
White, B.Y. (1993). ThinkerTools: Causal models, conceptual change, and science education. Cognition and Instruction , 10 (1), 1-100.
White, B.Y., and Frederiksen, J.R. (1998). Inquiry, modeling, and metacognition: Making science accessible to all students. Cognition and Instruction , 16 (1), 3-118.
White, R.T. (1996). The link between the laboratory and learning. International Journal of Science Education , 18 , 761-774.
White, R.T., and Gunstone, R.F. (1992). Probing understanding . London, England: Falmer.
Wilkenson, J.W., and Ward, M. (1997). The purpose and perceived effectiveness of laboratory work in secondary schools. Australian Science Teachers’ Journal , 43-55.
Wong, A.F.L., and Fraser, B.J. (1995). Cross-validation in Singapore of the science laboratory environment inventory. Psychological Reports , 76 , 907-911.
Woolnough, B.E. (1983). Exercises, investigations and experiences. Physics Education , 18 , 60-63.
Yager, R.E., Engen, J.B., and Snider, C.F. (1969). Effects of the laboratory and demonstration method upon the outcomes of instruction in secondary biology. Journal of Research in Science Teaching , 5 , 76-86.
Zimmerman, C. (2000). The development of scientific reasoning skills. Developmental Review , 20 , 99-149.
Laboratory experiences as a part of most U.S. high school science curricula have been taken for granted for decades, but they have rarely been carefully examined. What do they contribute to science learning? What can they contribute to science learning? What is the current status of labs in our nation's high schools as a context for learning science? This book looks at a range of questions about how laboratory experiences fit into U.S. high schools:
- What is effective laboratory teaching?
- What does research tell us about learning in high school science labs?
- How should student learning in laboratory experiences be assessed?
- Do all student have access to laboratory experiences?
- What changes need to be made to improve laboratory experiences for high school students?
- How can school organization contribute to effective laboratory teaching?
With increased attention to the U.S. education system and student outcomes, no part of the high school curriculum should escape scrutiny. This timely book investigates factors that influence a high school laboratory experience, looking closely at what currently takes place and what the goals of those experiences are and should be. Science educators, school administrators, policy makers, and parents will all benefit from a better understanding of the need for laboratory experiences to be an integral part of the science curriculum—and how that can be accomplished.
READ FREE ONLINE
Welcome to OpenBook!
You're looking at OpenBook, NAP.edu's online reading room since 1999. Based on feedback from you, our users, we've made some improvements that make it easier than ever to read thousands of publications on our website.
Do you want to take a quick tour of the OpenBook's features?
Show this book's table of contents , where you can jump to any chapter by name.
...or use these buttons to go back to the previous chapter or skip to the next one.
Jump up to the previous page or down to the next one. Also, you can type in a page number and press Enter to go directly to that page in the book.
Switch between the Original Pages , where you can read the report as it appeared in print, and Text Pages for the web version, where you can highlight and search the text.
To search the entire text of this book, type in your search term here and press Enter .
Share a link to this book page on your preferred social network or via email.
View our suggested citation for this chapter.
Ready to take your reading offline? Click here to buy this book in print or download it as a free PDF, if available.
Get Email Updates
Do you enjoy reading reports from the Academies online for free ? Sign up for email notifications and we'll let you know about new publications in your areas of interest when they're released.
Select a year to see courses
Learn online or on-campus during the term or school holidays
- OC Test Preparation
- Selective School Test Preparation
- Maths Acceleration
- English Advanced
- Maths Standard
- Maths Advanced
- Maths Extension 1
- English Standard
- Maths Extension 2
Get HSC exam ready in just a week
- UCAT Exam Preparation
Select a year to see available courses
- English Units 1/2
- Maths Methods Units 1/2
- Biology Units 1/2
- Chemistry Units 1/2
- Physics Units 1/2
- English Units 3/4
- Maths Methods Units 3/4
- Biology Unit 3/4
- Chemistry Unit 3/4
- Physics Unit 3/4
- UCAT Preparation Course
- Matrix Learning Methods
- Matrix Term Courses
- Matrix Holiday Courses
- Matrix+ Online Courses
- Campus overview
- Castle Hill
- Strathfield
- Sydney City
- Year 3 NAPLAN Guide
- OC Test Guide
- Selective Schools Guide
- NSW Primary School Rankings
- NSW High School Rankings
- NSW High Schools Guide
- ATAR & Scaling Guide
- HSC Study Planning Kit
- Student Success Secrets
- Reading List
- Year 6 English
- Year 7 & 8 English
- Year 9 English
- Year 10 English
- Year 11 English Standard
- Year 11 English Advanced
- Year 12 English Standard
- Year 12 English Advanced
- HSC English Skills
- How To Write An Essay
- How to Analyse Poetry
- English Techniques Toolkit
- Year 7 Maths
- Year 8 Maths
- Year 9 Maths
- Year 10 Maths
- Year 11 Maths Advanced
- Year 11 Maths Extension 1
- Year 12 Maths Standard 2
- Year 12 Maths Advanced
- Year 12 Maths Extension 1
- Year 12 Maths Extension 2
Science guides to help you get ahead
- Year 11 Biology
- Year 11 Chemistry
- Year 11 Physics
- Year 12 Biology
- Year 12 Chemistry
- Year 12 Physics
- Physics Practical Skills
- Periodic Table
- VIC School Rankings
- VCE English Study Guide
- Set Location
Welcome to Matrix Education
To ensure we are showing you the most relevant content, please select your location below.
How to Write a Scientific Report | Step-by-Step Guide
Got to document an experiment but don't know how? In this post, we'll guide you step-by-step through how to write a scientific report and provide you with an example.
Get free study tips and resources delivered to your inbox.
Join 75,893 students who already have a head start.
" * " indicates required fields
You might also like
- Helping My Child Study Effectively
- Year 10 Subject Selection Seminar Presentation
- How To Create A Study Plan That Works
- The Ultimate Macbeth Overview | Skills Understanding Shakespeare
- How To Approach Creative Writing in Years 9 and 10
Related courses
Year 9 science, year 10 science.
Is your teacher expecting you to write an experimental report for every class experiment? Are you still unsure about how to write a scientific report properly? Don’t fear! We’ll guide you through all parts of a scientific report, step-by-step.
How to write a scientific report:
- What is a scientific report
- General rules for writing Scientific reports
- Introduction/Background information
- Risk assessment
What is a scientific report?
A scientific report documents all aspects of an experimental investigation. This includes:
- The aim of the experiment
- The hypothesis
- An introduction to the relevant background theory
- The methods used
- The results
- A discussion of the results
- The conclusion
Scientific reports allow their readers to understand the experiment without doing it themselves. These reports also give others the opportunity to check the methodology of the experiment to ensure the validity of the results.
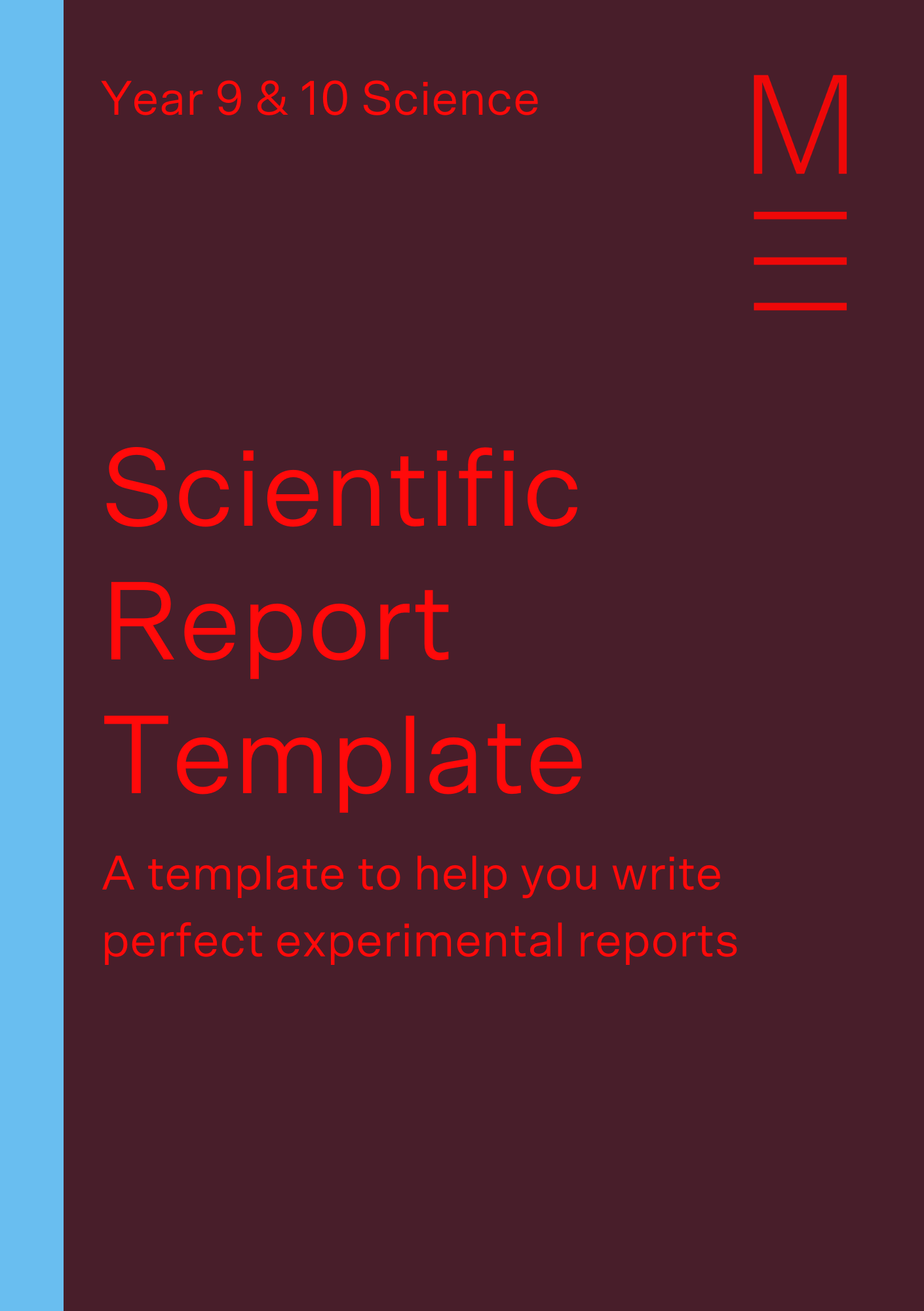
Download your free experimental report template
A template to teach you to write perfect science reports
Done! Your download has been emailed.
Please allow a few minutes for it to land in your inbox.
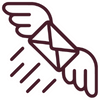
We take your privacy seriously. T&Cs and Privacy Policy .
A scientific report is written in several stages. We write the introduction, aim, and hypothesis before performing the experiment, record the results during the experiment, and complete the discussion and conclusions after the experiment.
But, before we delve deeper into how to write a scientific report, we need to have a science experiment to write about! Choose an experiment to use as an example from our 7 Simple Experiments You Can Do At Home article.
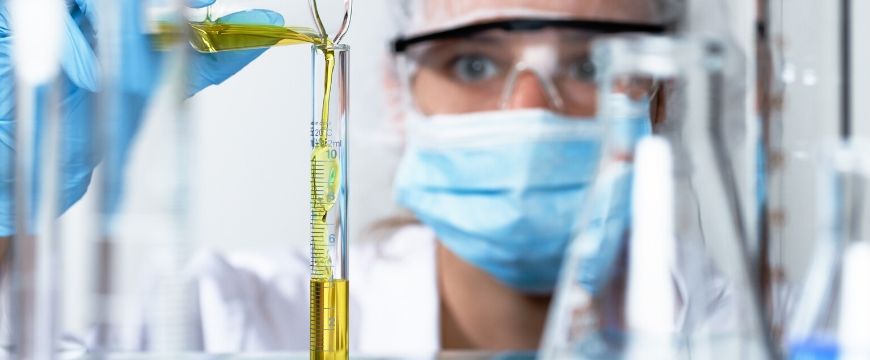
General rules for writing scientific reports
Learning how to write a scientific report is different from writing English essays or speeches.
You have to use:
- Passive voice (which you should avoid when writing for other subjects like English!)
- Past-tense language
- Headings and subheadings
- A pencil to draw scientific diagrams and graphs
- Simple and clear lines for scientific diagrams
- Tables and graphs where necessary
Structure of scientific reports
Now that you know the general rules on how to write scientific reports, let’s look at the conventions for their structure!
The title should simply introduce what your experiment is about.
The Role of Light in Photosynthesis
2. Introduction/Background information
Write a paragraph that gives your readers background information to understand your experiment.
This includes explaining scientific theories, processes, and other related knowledge.
Photosynthesis is a vital process for life. It occurs when plants intake carbon dioxide, water, and light, and results in the production of glucose and water. The light required for photosynthesis is absorbed by chlorophyll, the green pigment of plants, which is contained in the chloroplasts.
The glucose produced through photosynthesis is stored as starch, which is used as an energy source for the plant and its consumers.
The presence of starch in the leaves of a plant indicates that photosynthesis has occurred.
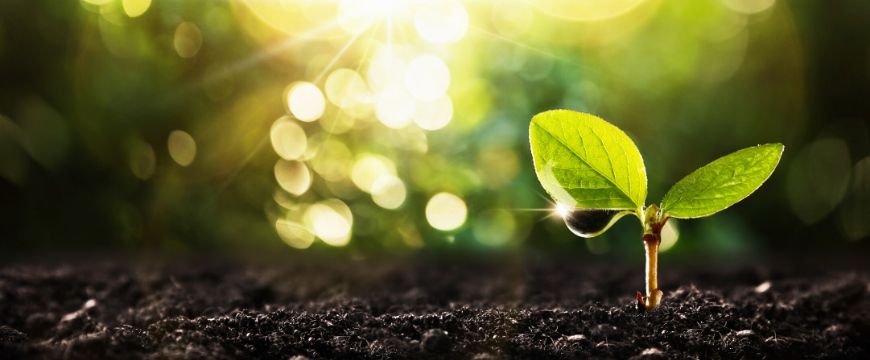
The aim tells us what is going to be tested in the experiment. This should be short, concise, and clear.
The aim of the experiment is to test whether light is required for photosynthesis to occur.
4. Hypothesis
The hypothesis is what you predict the outcome of the experiment will be. You have to use background information to make an educated prediction.
It is predicted that photosynthesis will occur only in leaves that are exposed to light and not in leaves that are not exposed to light. This will be indicated by the presence or absence of starch in the leaves.
5. Risk assessment
Identify the hazards related to the experiment and explain how you would prevent or minimise the risks. A hazard is something that can cause harm, and a risk is how likely it is that harm will occur because of the hazard.
A table is an excellent way to present your risk assessment.
Remember, you have to specify the type of harm that can occur because of the hazard. It’s not enough to simply identify the hazard.
- Do not write: “Scissors are sharp.”
- Instead, write: “Scissors are sharp and can cause injury.”
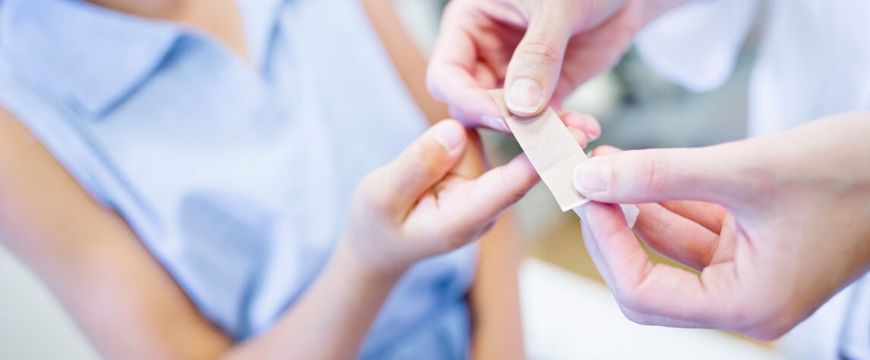
The method has 3 parts:
- A list of every material used
- Steps of what you did in the experiment
- A scientific diagram of the experimental apparatus
Let’s break down what you need to do for each section.
6a. Materials
This must list every piece of equipment and material used in the experiment.
Remember, you need to specify the amount of each material you used.
- 1 geranium plant
- Aluminium foil
- 2 test tubes
- 1 test tube rack
- 1 pair of scissors
- 1 250 mL beaker
- 1 pair of forceps
- 1 10 mL measuring cylinder
- Iodine solution (5 mL)
- Methylated spirit (50 mL)
- Boiling water
- 2 Petri dishes
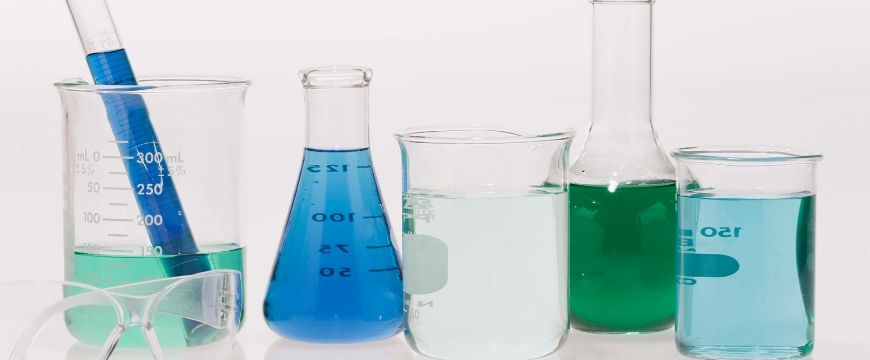
As a rule of thumb, you should write the method clearly and in a way that readers can repeat the experiment and get similar results.
Using a numbered list for the steps of your experimental procedure is much clearer than writing a whole paragraph of text. The steps should:
- Be written in a sequential order, based on when they were performed
- Specify any equipment that was used
- Specify the quantity of any materials that were used
You also need to use past tense and passive voice when you’re writing your method. Scientific reports are supposed to show the readers what you did in the experiment, not what you will do.
- Aluminium foil was used to fully cover a leaf of the geranium plant. The plant was left in the sun for three days.
- On the third day, the covered leaf and 1 non-covered leaf were collected from the plant. The foil was removed from the covered leaf, and a 1 cm square was cut from each leaf using a pair of scissors.
- 150 mL of water was boiled in a kettle and poured into a 250 mL beaker.
- Using forceps, the 1 cm square of covered leaf was placed into the beaker of boiling water for 2 minutes. It was then placed in a test tube labelled “dark”.
- The water in the beaker was discarded and replaced with 150 mL of freshly boiled water.
- Using forceps, the 1 cm square non-covered leaf was placed into the beaker of boiling water for 2 minutes. It was then placed in a test tube labelled “light”.
- 5 mL of methylated spirit was measured with a measuring cylinder and poured into each test tube so that the leaves were fully covered.
- The water in the beaker was replaced with 150 mL of freshly boiled water and both the “light” and “dark” test tubes were immersed in the beaker of boiling water for 5 minutes.
- The leaves were collected from each test tube with forceps, rinsed under cold running water, and placed onto separate labelled Petri dishes.
- 3 drops of iodine solution were added to each leaf.
- Both Petri dishes were placed side by side and observations were recorded.
- The experiment was repeated 5 times, and results were compared between different groups.
6c. Diagram
After you complete your steps, it’s time to draw your scientific diagrams! Here are some rules for drawing scientific diagrams:
- Always use a pencil to draw your scientific diagrams.
- Use simple, sharp, 2D lines and shapes to draw your diagram. Don’t draw 3D shapes or use shading.
- Label everything in your diagram.
- Use thin, straight lines to label your diagram. Don’t use arrows.
- Ensure that the label lines touch the outline of the equipment you are labelling and do not cross over it or stop short of it.
- The label lines should never cross over each other.
- Use a ruler for any straight lines in your diagram.
- Draw a sufficiently large diagram so all components can be seen clearly.
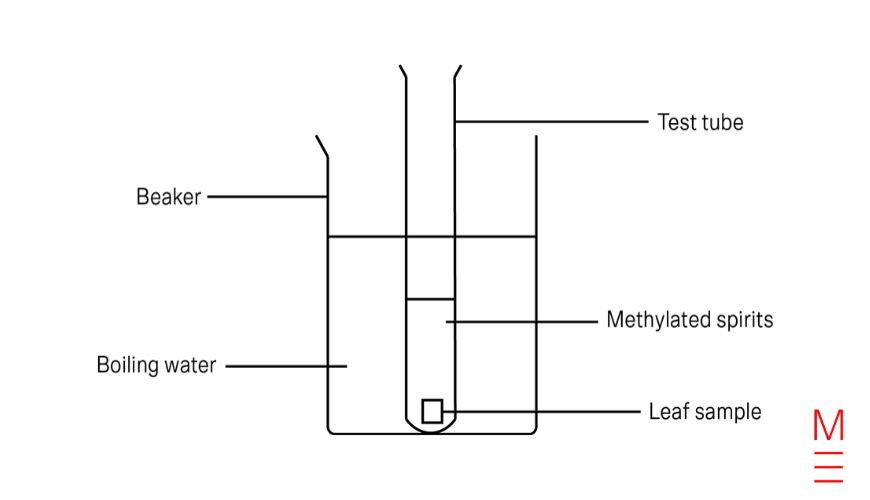
This is where you document the results of your experiment. The data that you record for your experiment will generally be qualitative and/or quantitative.
Qualitative data is data that relates to qualities and is based on observations (qualitative – quality). This type of data is descriptive and is recorded in words. For example, the colour changed from green to orange, or the liquid became hot.
Quantitative data refers to n umerical data (quantitative – quantity). This type of data is recorded using numbers and is either measured or counted. For example, the plant grew 5.2 cm, or there were 5 frogs.
The way you record your results is important. Most of the time, a table is the best way to do this.
Here are some rules to using tables
- Use a pencil and a ruler to draw your table.
- Draw neat and straight lines.
- Ensure that the table is closed (connect all your lines).
- Don’t cross your lines (erase any lines that stick out of the table).
- Use appropriate columns and rows.
- Properly name each column and row (including the units of measurement in brackets).
- Do not write your units in the body of your table (units belong in the header).
- Always include a title.
Note : If your results require calculations, clearly write each step.
Observations of the effects of light on the amount of starch in plant leaves.
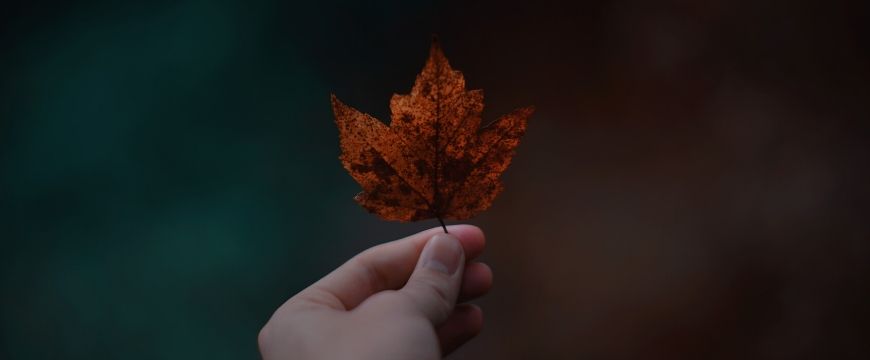
If quantitative data was recorded, the data is often also plotted on a graph.
8. Discussion
The discussion is where you analyse and interpret your results, and identify any experimental errors or possible areas of improvements.
You should divide your discussion as follows.
1. Trend in the results
Describe the ‘trend’ in your results. That is, the relationship you observed between your independent and dependent variables.
The independent variable is the variable that you’re changing in the experiment. In this experiment, it’s the amount of light that the leaves are exposed to.
The dependent variable is the variable that you’re measuring in the experiment. In this experiment, it’s the presence of starch in the leaves.
The presence of starch is indicated when the addition of iodine causes the leaf to turn dark purple. The results show that starch was present in the leaves that were exposed to light, while the leaves that were not exposed to light did not contain starch.
2. Scientific explanation
Give an explanation of the results using scientific knowledge, theories, and any other scientific resources you find.
As starch is produced during photosynthesis, these results show that light plays a key role in photosynthesis.
3. Validity
Validity refers to whether or not your results are valid. This can be done by examining your variables.
VA lidity = VA riables
Identify the independent, dependent, controlled variables, and the control experiment (if you have one).
The controlled variables are the variables that you keep the same across all tests e.g. the size of the leaf sample.
The control experiment is where you don’t apply an independent variable. It is untouched for the whole experiment.
Ensure that you never change more than one variable at a time!
The independent variable of the experiment was the amount of light that the leaves were exposed to (the covered and uncovered geranium leaf), while the dependent variable was the presence of starch. The controlled variables were the size of the leaf sample, the duration of the experiment, the amount of time the solutions were heated, and the amount of iodine solution used.
4. Reliability
Identify how you ensured the reliability of the results.
RE liability = RE petition
Show that you repeated your experiments, cross-checked your results with other groups, or collated your results with the class.
The reliability of the results was ensured by repeating the experiment 5 times and comparing results with other groups. Since other groups obtained comparable results, the results are reliable.
5. Accuracy
You should discuss accuracy if your results are in the form of quantitative data (numerical), and there is an accepted value for the result.
Accuracy wouldn’t be discussed for our photosynthesis experiment as qualitative data was collected. But, it would be discussed if we were measuring gravity using a pendulum:
The measured value of gravity was 9.8 m/s 2 , which is in agreement with the accepted value of 9.8 m/s 2 .
6. Possible improvements
Point out any errors or risks you found in the experiment and suggest a method to improve it.
If there are none, then suggest new ways to improve the experimental design, and/or minimise error and risks.
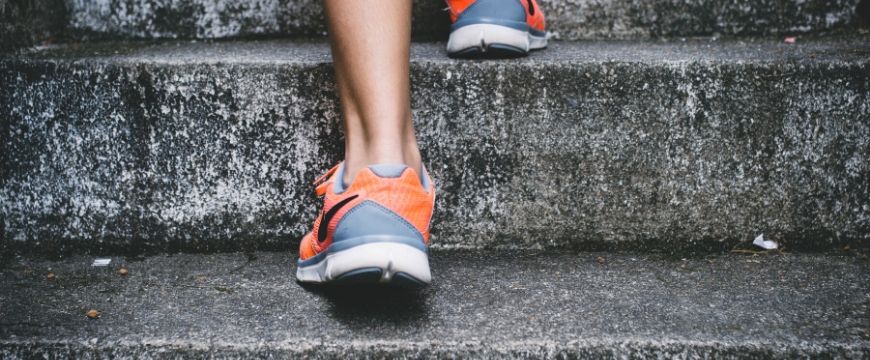
Possible improvements could be made by including control experiments. For example, testing whether the iodine solution turns dark purple when added to water or methylated spirits. This would help to ensure that the purple colour observed in the experiments is due to the presence of starch in the leaves rather than impurities.
9. Conclusion
State whether the aim was achieved and if your hypothesis was supported.
The aim of the investigation was achieved, and it was found that light is required for photosynthesis to occur. This was evidenced by the presence of starch in leaves that had been exposed to light, and the absence of starch in leaves that had been unexposed. These results support the proposed hypothesis.
Learn everything you need to know about Science
Gain the confidence and skills to ace Science!
Learn one term ahead of school with the Subject Matter Experts!
Learn more ?
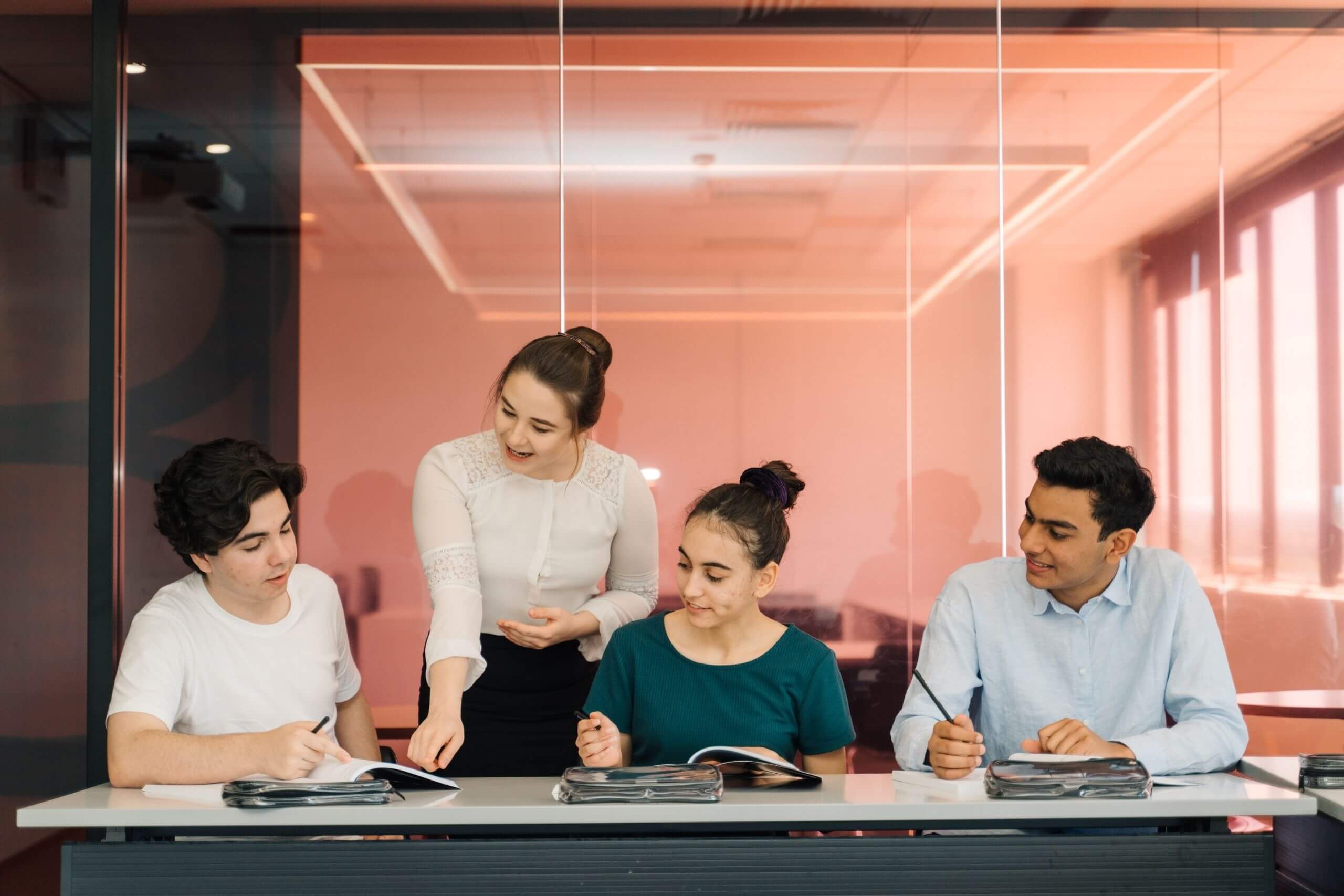
Written by Matrix Science Team
© Matrix Education and www.matrix.edu.au, 2023. Unauthorised use and/or duplication of this material without express and written permission from this site’s author and/or owner is strictly prohibited. Excerpts and links may be used, provided that full and clear credit is given to Matrix Education and www.matrix.edu.au with appropriate and specific direction to the original content.
Year 9 Science tutoring at Matrix is known for helping students build a strong foundation before studying Biology, Chemistry or Physics in senior school.
Learning methods available
Level 9 Science tutoring program
Year 10 Science tutoring at Matrix is known for helping students build a strong foundation before studying Biology, Chemistry or Physics in Year 11 and 12.
Level 10 Science tutoring program for Victorian students
Related articles
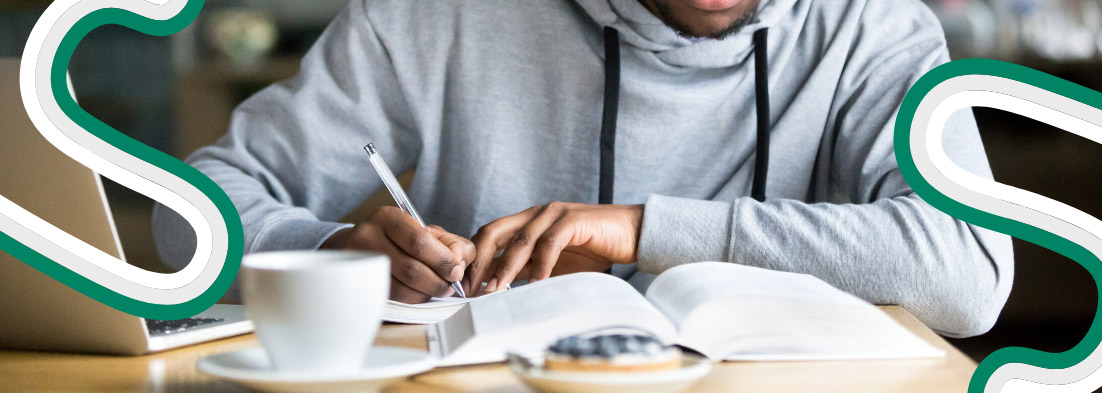
How to Plan Before You Write | Dos and Don’ts for Year 7 & 8 English
Confused about planning your responses? Don't worry, in this article we explain why 7 & 8 students must plan their responses and give you the does and don'ts of how to plan before you write!
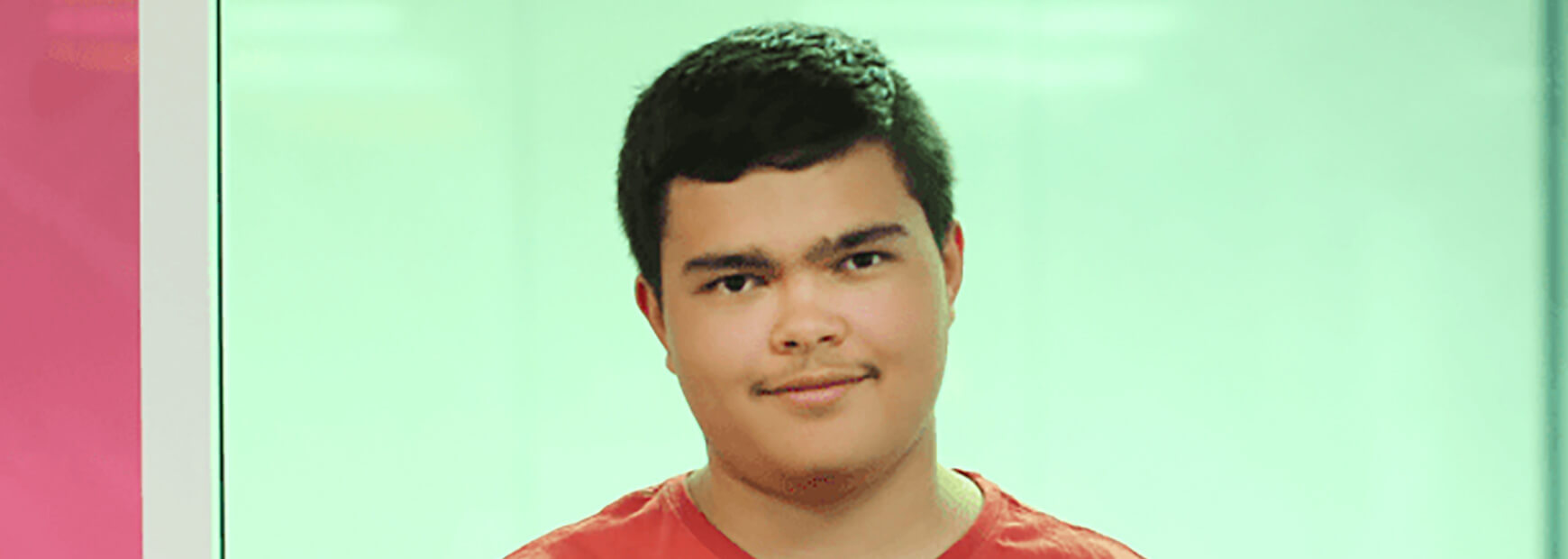
State & National Science Awards Winner – Callum Avery
In this post, Callum relates his experiences with science competitions.
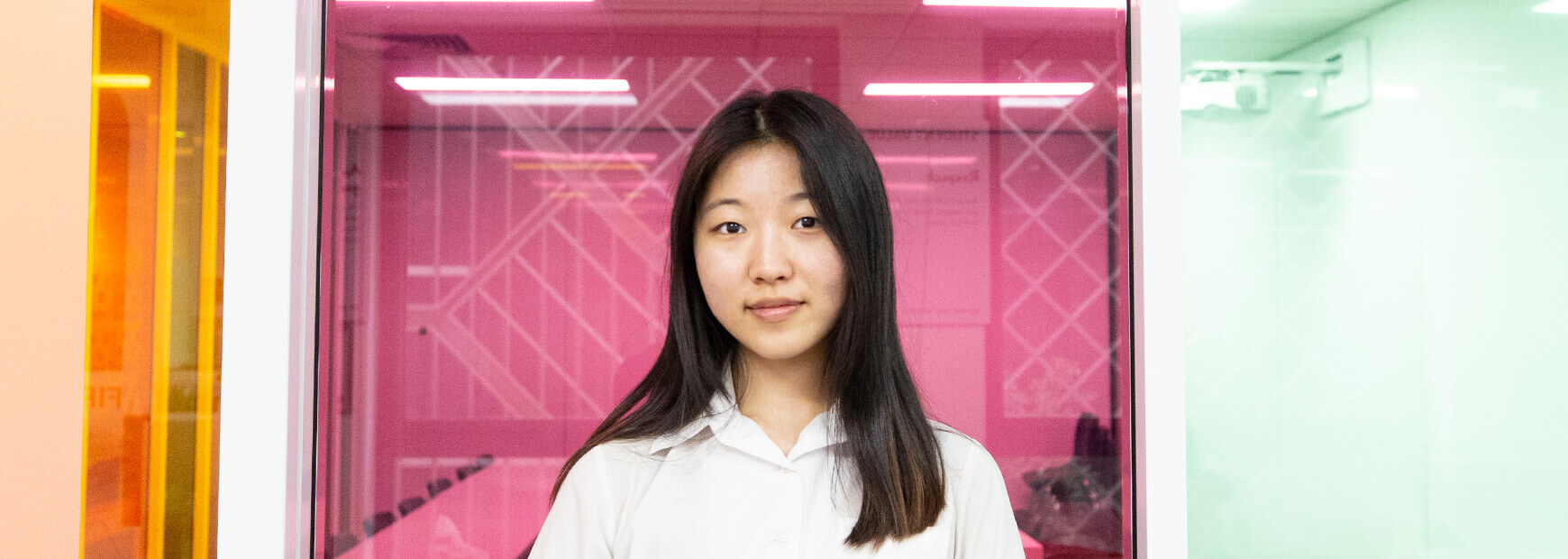
Angela’s Hacks: Get A Head Start On Year 12 By Accelerating Maths
Deciding on taking an accelerated course? Want to improve in Maths? In this post, Angela gives you all the best tips to make the most out of your classes.

- Scientific Lab Reports
- Understanding the Assignment
- Need a Topic?
- Evaluating Sources
- Brainstorming Strategies
- Drafting Strategies
- Thesis Formulation
- Introductions
- Conclusions
- Show Don't Tell
- Expand Your Draft
- Flow & Lexical Coherence
- Revision Checklist
- Introduction to Style and Grammar
- Apostrophes
- Article Usage for ESL Learners
- Capitalization
- Clarity: Get Rid of Nominalizations
- Cohesion: Does my Paragraph Flow?
- Commas and Colons
- Conciseness
- Confusing Words
- Parallel Structure
- Passive Voice
- Quotation Marks
- Run-on Sentences
- Subject-Verb Agreement
- Writing Mechanics
- Other Styles
- Art History This link opens in a new window
- Programming Lab Reports
Writing a Lab Report
Link to other resources.
- Screenwriting
- Publication Opportunities
- Meet with a Tutor This link opens in a new window
Academic Resource Center Hours :
Monday-Thursday: 8:00 AM-6:00 PM
Friday: 8:00 AM-4:00 PM
Phone Number : 310-338-2847
Email : [email protected]
www.lmu.edu/arc
We are located in Daum Hall on the 2nd floor!
Writing a scientific lab report is significantly different from writing for other classes like philosophy, English, and history. The most prominent form of writing in biology, chemistry, and environmental science is the lab report, which is a formally written description of results and discoveries found in an experiment. College lab reports should emulate and follow the same formats as reports found in scholarly journals, such as Nature , Cell , and The American Journal of Biochemistry .
Report Format
Title: The title says what you did. It should be brief (aim for ten words or less) and describe the main point of the experiment or investigation.
- Example: Caffeine Increases Amylase Activity in the Mealworm ( Tenebrio molitar).
- If you can, begin your title using a keyword rather than an article like “The” or “A.”
Abstract: An abstract is a very concise summary of the purpose of the report, data presented, and major conclusions in about 100 - 200 words. Abstracts are also commonly required for conference/presentation submissions because they summarize all of the essential materials necessary to understand the purpose of the experiment. They should consist of a background sentence , an introduction sentence , your hypothesis/purpose of the experiment, and a sentence about the results and what this means.
Introduction: The introduction of a lab report defines the subject of the report, provides background information and relevant studies, and outlines scientific purpose(s) and/or objective(s).
- The introduction is a place to provide the reader with necessary research on the topic and properly cite sources used.
- Summarizes the current literature on the topic including primary and secondary sources.
- Introduces the paper’s aims and scope.
- States the purpose of the experiment and the hypothesis.
Materials and Methods: The materials and methods section is a vital component of any formal lab report. This section of the report gives a detailed account of the procedure that was followed in completing the experiment as well as all important materials used. (This includes bacterial strains and species names in tests using living subjects.)
- Discusses the procedure of the experiment in as much detail as possible.
- Provides information about participants, apparatus, tools, substances, location of experiment, etc.
- For field studies, be sure to clearly explain where and when the work was done.
- It must be written so that anyone can use the methods section as instructions for exact replications.
- Don’t hesitate to use subheadings to organize these categories.
- Practice proper scientific writing forms. Be sure to use the proper abbreviations for units. Example: The 50mL sample was placed in a 5ºC room for 48hrs.
Results: The results section focuses on the findings, or data, in the experiment, as well as any statistical tests used to determine their significance.
- Concentrate on general trends and differences and not on trivial details.
- Summarize the data from the experiments without discussing their implications (This is where all the statistical analyses goes.)
- Organize data into tables, figures, graphs, photographs, etc. Data in a table should not be duplicated in a graph or figure. Be sure to refer to tables and graphs in the written portion, for example, “Figure 1 shows that the activity....”
- Number and title all figures and tables separately, for example, Figure 1 and Table 1 and include a legend explaining symbols and abbreviations. Figures and graphs are labeled below the image while tables are labeled above.
Discussion: The discussion section interprets the results, tying them back to background information and experiments performed by others in the past.This is also the area where further research opportunities shold be explored.
- Interpret the data; do not restate the results.
- Observations should also be noted in this section, especially anything unusual which may affect your results.
For example, if your bacteria was incubated at the wrong temperature or a piece of equipment failed mid-experiment, these should be noted in the results section.
- Relate results to existing theories and knowledge.This can tie back to your introduction section because of the background you provided.
- Explain the logic that allows you to accept or reject your original hypotheses.
- Include suggestions for improving your techniques or design, or clarify areas of doubt for further research.
Acknowledgements and References: A references list should be compiled at the end of the report citing any works that were used to support the paper. Additionally, an acknowledgements section should be included to acknowledge research advisors/ partners, any group or person providing funding for the research and anyone outside the authors who contributed to the paper or research.
General Tips
- In scientific papers, passive voice is perfectly acceptable. On the other hand, using “I” or “we” is not.
Incorrect: We found that caffeine increased amylase levels in Tenebrio molitar. Correct: It was discovered that caffeine increased amylase levels in Tenebrio molitar.
- It is expected that you use as much formal (bland) language and scientific terminology as you can. There should be no emphasis placed on “expressing yourself” or “keeping it interesting”; a lab report is not a narrative.
- In a lab report, it is important to get to the point. Be descriptive enough that your audience can understand the experiment, but strive to be concise.
- << Previous: Programming Lab Reports
- Next: Screenwriting >>
- Last Updated: Nov 7, 2024 12:52 PM
- URL: https://libguides.lmu.edu/writing

IMAGES
VIDEO
COMMENTS
OUR SCHOOL LABORATORY ESSAY. We are living in the world of science and technology. So, the need to understand scientific concepts and theories is extremely great. In response to this dire need, facilities for the study of physical sciences have been given great extension in both the higher and lower centers of education.
These science essays can be used for essay writing, debate, and other related activities at your institution or school. Essay On Science (200 words) Science entails a thorough examination of the behavior of the physical and natural world. Research, experimentation, and observation are used in the study. The scientific disciplines are diverse.
The main purpose of a lab report is to demonstrate your understanding of the scientific method by performing and evaluating a hands-on lab experiment. This type of assignment is usually shorter than a research paper. Lab reports are commonly used in science, technology, engineering, and mathematics (STEM) fields.
The science lab essay is a fundamental component of scientific study, serving as a detailed record of an experiment and its results. It follows a specific structure and format, designed to ensure clarity, consistency, and comprehensibility. This essay will delve into the structure and format of a science lab essay, discussing their importance ...
Dr Peter Judge | Tutorial Essays for Science Subjects 1 Tutorial Essays for Science Subjects This guide is designed to provide help and advice on scientific writing. Although students studying Medical and Life Sciences are most likely to have to write essays for tutorials at Oxford, it is important all scientists
Perhaps you're in the midst of your challenging AP chemistry class in high school, or perhaps college you're enrolled in biology, chemistry, or physics at university.At some point, you will likely be asked to write a lab report. Sometimes, your teacher or professor will give you specific instructions for how to format and write your lab report, and if so, use that.
The science learning goals of laboratory experiences include enhancing mastery of science subject matter, developing scientific reasoning abilities, increasing understanding of the complexity and ambiguity of empirical work, developing practical skills, increasing understanding of the nature of science, cultivating interest in science and science learning, and improving teamwork abilities.
Learning how to write a scientific report is different from writing English essays or speeches. You have to use: Passive voice (which you should avoid when writing for other subjects like English!) ... Learn everything you need to know about Science. Gain the confidence and skills to ace Science! Learn one term ahead of school with the Subject ...
Writing a scientific lab report is significantly different from writing for other classes like philosophy, English, and history. The most prominent form of writing in biology, chemistry, and environmental science is the lab report, which is a formally written description of results and discoveries found in an experiment.
Students tend to approach writing lab reports with confusion and dread. Whether in high school science classes or undergraduate laboratories, experiments are always fun and games until the times comes to submit a lab report. What if we didn't need to spend hours agonizing over this piece of scientific writing?