14.7 Acid-Base Titrations
Learning objectives.
By the end of this section, you will be able to:
- Interpret titration curves for strong and weak acid-base systems
- Compute sample pH at important stages of a titration
- Explain the function of acid-base indicators
As seen in the chapter on the stoichiometry of chemical reactions, titrations can be used to quantitatively analyze solutions for their acid or base concentrations. In this section, we will explore the underlying chemical equilibria that make acid-base titrimetry a useful analytical technique.

Titration Curves
A titration curve is a plot of some solution property versus the amount of added titrant. For acid-base titrations, solution pH is a useful property to monitor because it varies predictably with the solution composition and, therefore, may be used to monitor the titration’s progress and detect its end point. The following example exercise demonstrates the computation of pH for a titration solution after additions of several specified titrant volumes. The first example involves a strong acid titration that requires only stoichiometric calculations to derive the solution pH. The second example addresses a weak acid titration requiring equilibrium calculations.
Example 14.21
Calculating ph for titration solutions: strong acid/strong base.
(a) 0.00 mL
(b) 12.50 mL
(c) 25.00 mL
(d) 37.50 mL
(b) Titrant volume = 12.50 mL. Since the acid sample and the base titrant are both monoprotic and equally concentrated, this titrant addition involves less than a stoichiometric amount of base, and so it is completely consumed by reaction with the excess acid in the sample. The concentration of acid remaining is computed by subtracting the consumed amount from the intial amount and then dividing by the solution volume:
(c) Titrant volume = 25.00 mL. This titrant addition involves a stoichiometric amount of base (the equivalence point ), and so only products of the neutralization reaction are in solution (water and NaCl). Neither the cation nor the anion of this salt undergo acid-base ionization; the only process generating hydronium ions is the autoprotolysis of water. The solution is neutral, having a pH = 7.00.
(d) Titrant volume = 37.50 mL. This involves the addition of titrant in excess of the equivalence point. The solution pH is then calculated using the concentration of hydroxide ion:
pH = 14 − pOH = 14 + log([OH − ]) = 14 + log(0.0200) = 12.30
Check Your Learning
0.00: 1.000; 15.0: 1.5111; 25.0: 7; 40.0: 12.523
Example 14.22
Titration of a weak acid with a strong base.
Calculate the pH of the titration solution after the addition of the following volumes of NaOH titrant:
(b) 25.00 mL
(c) 12.50 mL
K a = [ H 3 O + ] [ CH 3 CO 2 − ] [ CH 3 CO 2 H ] ≈ [ H 3 O + ] 2 [ CH 3 CO 2 H ] 0 , K a = [ H 3 O + ] [ CH 3 CO 2 − ] [ CH 3 CO 2 H ] ≈ [ H 3 O + ] 2 [ CH 3 CO 2 H ] 0 , and [ H 3 O + ] = K a × [ CH 3 CO 2 H ] = 1.8 × 10 −5 × 0.100 = 1.3 × 10 −3 [ H 3 O + ] = K a × [ CH 3 CO 2 H ] = 1.8 × 10 −5 × 0.100 = 1.3 × 10 −3
(b) The acid and titrant are both monoprotic and the sample and titrant solutions are equally concentrated; thus, this volume of titrant represents the equivalence point. Unlike the strong-acid example above, however, the reaction mixture in this case contains a weak conjugate base (acetate ion). The solution pH is computed considering the base ionization of acetate, which is present at a concentration of
Base ionization of acetate is represented by the equation
Assuming x << 0.0500, the pH may be calculated via the usual ICE approach: K b = x 2 0.0500 M K b = x 2 0.0500 M
Note that the pH at the equivalence point of this titration is significantly greater than 7, as expected when titrating a weak acid with a strong base.
(c) Titrant volume = 12.50 mL. This volume represents one-half of the stoichiometric amount of titrant, and so one-half of the acetic acid has been neutralized to yield an equivalent amount of acetate ion. The concentrations of these conjugate acid-base partners, therefore, are equal. A convenient approach to computing the pH is use of the Henderson-Hasselbalch equation:
(pH = p K a at the half-equivalence point in a titration of a weak acid)
(d) Titrant volume = 37.50 mL. This volume represents a stoichiometric excess of titrant, and a reaction solution containing both the titration product, acetate ion, and the excess strong titrant. In such solutions, the solution pH is determined primarily by the amount of excess strong base:
0.00 mL: 2.37; 15.0 mL: 3.92; 25.00 mL: 8.29; 30.0 mL: 12.097
Performing additional calculations similar to those in the preceding example permits a more full assessment of titration curves. A summary of pH/volume data pairs for the strong and weak acid titrations is provided in Table 14.2 and plotted as titration curves in Figure 14.18 . A comparison of these two curves illustrates several important concepts that are best addressed by identifying the four stages of a titration:
initial state (added titrant volume = 0 mL): pH is determined by the acid being titrated; because the two acid samples are equally concentrated, the weak acid will exhibit a greater initial pH
pre-equivalence point (0 mL < V < 25 mL): solution pH increases gradually and the acid is consumed by reaction with added titrant; composition includes unreacted acid and the reaction product, its conjugate base
equivalence point ( V = 25 mL): a drastic rise in pH is observed as the solution composition transitions from acidic to either neutral (for the strong acid sample) or basic (for the weak acid sample), with pH determined by ionization of the conjugate base of the acid
postequivalence point ( V > 25 mL): pH is determined by the amount of excess strong base titrant added; since both samples are titrated with the same titrant, both titration curves appear similar at this stage.
Volume of 0.100 NaOH Added (mL) | Moles of NaOH Added | pH Values 0.100 HCl | pH Values 0.100 CH CO H |
---|---|---|---|
0.0 | 0.0 | 1.00 | 2.87 |
5.0 | 0.00050 | 1.18 | 4.14 |
10.0 | 0.00100 | 1.37 | 4.57 |
15.0 | 0.00150 | 1.60 | 4.92 |
20.0 | 0.00200 | 1.95 | 5.35 |
22.0 | 0.00220 | 2.20 | 5.61 |
24.0 | 0.00240 | 2.69 | 6.13 |
24.5 | 0.00245 | 3.00 | 6.44 |
24.9 | 0.00249 | 3.70 | 7.14 |
25.0 | 0.00250 | 7.00 | 8.72 |
25.1 | 0.00251 | 10.30 | 10.30 |
25.5 | 0.00255 | 11.00 | 11.00 |
26.0 | 0.00260 | 11.29 | 11.29 |
28.0 | 0.00280 | 11.75 | 11.75 |
30.0 | 0.00300 | 11.96 | 11.96 |
35.0 | 0.00350 | 12.22 | 12.22 |
40.0 | 0.00400 | 12.36 | 12.36 |
45.0 | 0.00450 | 12.46 | 12.46 |
50.0 | 0.00500 | 12.52 | 12.52 |
Acid-Base Indicators
Certain organic substances change color in dilute solution when the hydronium ion concentration reaches a particular value. For example, phenolphthalein is a colorless substance in any aqueous solution with a hydronium ion concentration greater than 5.0 × × 10 −9 M (pH < 8.3). In more basic solutions where the hydronium ion concentration is less than 5.0 × × 10 −9 M (pH > 8.3), it is red or pink. Substances such as phenolphthalein, which can be used to determine the pH of a solution, are called acid-base indicators . Acid-base indicators are either weak organic acids or weak organic bases.
The equilibrium in a solution of the acid-base indicator methyl orange, a weak acid, can be represented by an equation in which we use HIn as a simple representation for the complex methyl orange molecule:
The anion of methyl orange, In − , is yellow, and the nonionized form, HIn, is red. When we add acid to a solution of methyl orange, the increased hydronium ion concentration shifts the equilibrium toward the nonionized red form, in accordance with Le Châtelier’s principle. If we add base, we shift the equilibrium towards the yellow form. This behavior is completely analogous to the action of buffers.
The perceived color of an indicator solution is determined by the ratio of the concentrations of the two species In − and HIn. If most of the indicator (typically about 60−90% or more) is present as In − , the perceived color of the solution is yellow. If most is present as HIn, then the solution color appears red. The Henderson-Hasselbalch equation is useful for understanding the relationship between the pH of an indicator solution and its composition (thus, perceived color):
In solutions where pH > p K a , the logarithmic term must be positive, indicating an excess of the conjugate base form of the indicator (yellow solution). When pH < p K a , the log term must be negative, indicating an excess of the conjugate acid (red solution). When the solution pH is close to the indicator pKa, appreciable amounts of both conjugate partners are present, and the solution color is that of an additive combination of each (yellow and red, yielding orange). The color change interval (or pH interval ) for an acid-base indicator is defined as the range of pH values over which a change in color is observed, and for most indicators this range is approximately p K a ± 1.
There are many different acid-base indicators that cover a wide range of pH values and can be used to determine the approximate pH of an unknown solution by a process of elimination. Universal indicators and pH paper contain a mixture of indicators and exhibit different colors at different pHs. Figure 14.19 presents several indicators, their colors, and their color-change intervals.
The titration curves shown in Figure 14.20 illustrate the choice of a suitable indicator for specific titrations. In the strong acid titration, use of any of the three indicators should yield reasonably sharp color changes and accurate end point determinations. For this titration, the solution pH reaches the lower limit of the methyl orange color change interval after addition of ~24 mL of titrant, at which point the initially red solution would begin to appear orange. When 25 mL of titrant has been added (the equivalence point), the pH is well above the upper limit and the solution will appear yellow. The titration's end point may then be estimated as the volume of titrant that yields a distinct orange-to-yellow color change. This color change would be challenging for most human eyes to precisely discern. More-accurate estimates of the titration end point are possible using either litmus or phenolphthalein, both of which exhibit color change intervals that are encompassed by the steep rise in pH that occurs around the 25.00 mL equivalence point.
The weak acid titration curve in Figure 14.20 shows that only one of the three indicators is suitable for end point detection. If methyl orange is used in this titration, the solution will undergo a gradual red-to-orange-to-yellow color change over a relatively large volume interval (0–6 mL), completing the color change well before the equivalence point (25 mL) has been reached. Use of litmus would show a color change that begins after adding 7–8 mL of titrant and ends just before the equivalence point. Phenolphthalein, on the other hand, exhibits a color change interval that nicely brackets the abrupt change in pH occurring at the titration's equivalence point. A sharp color change from colorless to pink will be observed within a very small volume interval around the equivalence point.
- 1 Titration of 25.00 mL of 0.100 M HCl (0.00250 mol of HCI) with 0.100 M NaOH.
- 2 Titration of 25.00 mL of 0.100 M CH 3 CO 2 H (0.00250 mol of CH 3 CO 2 H) with 0.100 M NaOH.
As an Amazon Associate we earn from qualifying purchases.
This book may not be used in the training of large language models or otherwise be ingested into large language models or generative AI offerings without OpenStax's permission.
Want to cite, share, or modify this book? This book uses the Creative Commons Attribution License and you must attribute OpenStax.
Access for free at https://openstax.org/books/chemistry-2e/pages/1-introduction
- Authors: Paul Flowers, Klaus Theopold, Richard Langley, William R. Robinson, PhD
- Publisher/website: OpenStax
- Book title: Chemistry 2e
- Publication date: Feb 14, 2019
- Location: Houston, Texas
- Book URL: https://openstax.org/books/chemistry-2e/pages/1-introduction
- Section URL: https://openstax.org/books/chemistry-2e/pages/14-7-acid-base-titrations
© Jan 8, 2024 OpenStax. Textbook content produced by OpenStax is licensed under a Creative Commons Attribution License . The OpenStax name, OpenStax logo, OpenStax book covers, OpenStax CNX name, and OpenStax CNX logo are not subject to the Creative Commons license and may not be reproduced without the prior and express written consent of Rice University.
Your browser is not supported
Sorry but it looks as if your browser is out of date. To get the best experience using our site we recommend that you upgrade or switch browsers.
Find a solution
- Skip to main content
- Skip to navigation
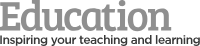
- Back to parent navigation item
- Primary teacher
- Secondary/FE teacher
- Early career or student teacher
- Higher education
- Curriculum support
- Literacy in science teaching
- Periodic table
- Interactive periodic table
- Climate change and sustainability
- Resources shop
- Collections
- Post-lockdown teaching support
- Remote teaching support
- Starters for ten
- Screen experiments
- Assessment for learning
- Microscale chemistry
- Faces of chemistry
- Classic chemistry experiments
- Nuffield practical collection
- Anecdotes for chemistry teachers
- On this day in chemistry
- Global experiments
- PhET interactive simulations
- Chemistry vignettes
- Context and problem based learning
- Journal of the month
- Chemistry and art
- Art analysis
- Pigments and colours
- Ancient art: today's technology
- Psychology and art theory
- Art and archaeology
- Artists as chemists
- The physics of restoration and conservation
- Ancient Egyptian art
- Ancient Greek art
- Ancient Roman art
- Classic chemistry demonstrations
- In search of solutions
- In search of more solutions
- Creative problem-solving in chemistry
- Solar spark
- Chemistry for non-specialists
- Health and safety in higher education
- Analytical chemistry introductions
- Exhibition chemistry
- Introductory maths for higher education
- Commercial skills for chemists
- Kitchen chemistry
- Journals how to guides
- Chemistry in health
- Chemistry in sport
- Chemistry in your cupboard
- Chocolate chemistry
- Adnoddau addysgu cemeg Cymraeg
- The chemistry of fireworks
- Festive chemistry
- Education in Chemistry
- Teach Chemistry
- On-demand online
- Live online
- Selected PD articles
- PD for primary teachers
- PD for secondary teachers
- What we offer
- Chartered Science Teacher (CSciTeach)
- Teacher mentoring
- UK Chemistry Olympiad
- Who can enter?
- How does it work?
- Resources and past papers
- Top of the Bench
- Schools' Analyst
- Regional support
- Education coordinators
- RSC Yusuf Hamied Inspirational Science Programme
- RSC Education News
- Supporting teacher training
- Interest groups
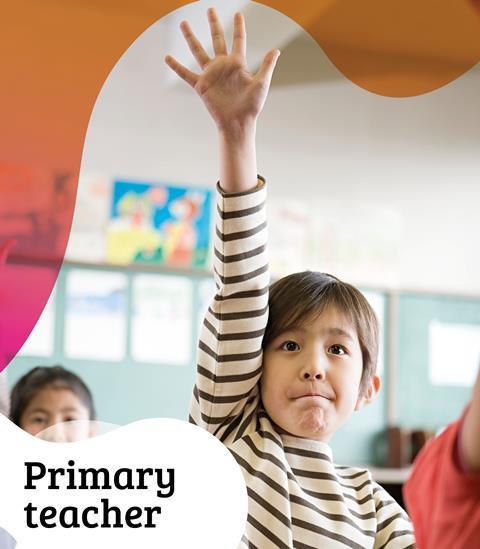
- More navigation items
A microscale acid–base titration
In association with Nuffield Foundation
- Five out of five
Use microscale titration to complete an acid–base neutralisation with sodium hydroxide in this class practical
In this experiment, students use a microscale titration apparatus – prepared from pipettes, a syringe and some rubber or plastic tubing – to carry out a titration, filling the ‘burette’ with hydrochloric acid and placing sodium hydroxide solution in a beaker. Students then try to calculate the exact concentration of the sodium hydroxide solution.
For this microscale technique manipulative skills are important, and students need to be capable of careful manipulation to carry the experiment out successfully. Students also need to be familiar with the concept of the mole, and capable of performing the calculations from the results of the experiment.
On such a small scale, safety issues are minimal. Similarly, the time taken to carry out a titration should be much reduced as the volumes being reacted are so small. It should be possible for a class to carry out the practical work and calculations in a one-hour session.
- Graduated glass pipette, 2 cm 3
- Pipette, 1 cm 3 , and pipette filler to fit (or a 1 cm 3 plastic syringe)
- Plastic syringe, 10 cm 3
- Fine-tip poly(ethene) dropping pipette (see note 6 below)
- Small lengths of rubber, plastic or silicone tubing
- Beakers, 10 cm 3 , x2
- Clamp stand with two bosses and clamps
- Dilute hydrochloric acid, 0.10 M, about 10 cm 3
- Sodium hydroxide solution, approx. 0.1 M (IRRITANT), about 10 cm 3
- Phenolphthalein indicator solution (HIGHLY FLAMMABLE), a few drops
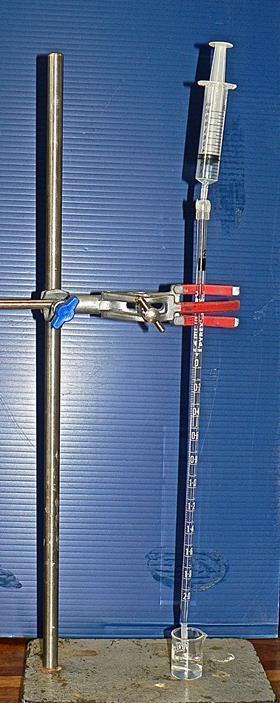
Source: Royal Society of Chemistry
The microscale titration apparatus ready to be used
Health, safety and technical notes
- Read our standard health and safety guidance.
- Wear eye protection throughout.
- Dilute hydrochloric acid, HCl(aq) – see CLEAPSS Hazcard HC047a and CLEAPSS Recipe Book RB043.
- Sodium hydroxide solution, NaOH(aq), (IRRITANT at concentration used) – see CLEAPSS Hazcard HC091a and CLEAPSS Recipe Book RB085. Students are to calculate the concentration of the sodium hydroxide solution so the bottle should not be labelled with the exact concentration.
- Phenolphthalein indicator solution (HIGHLY FLAMMABLE) – see CLEAPSS Hazcard HC032 and CLEAPSS Recipe Book RB000.
- A suitable poly(ethene) dropping pipette would be fine-tip standard, non-sterile, 3.3 cm 3 capacity, such as those available from Sigma-Aldrich.
- Sargent-Welch produce eady-made microscale titration kits.
Preparing the microscale titration apparatus
The microscale titration apparatus replaces the normal burette. To make the microscale titration apparatus, cut the tip end off a fine-tip poly(ethene) dropping pipette and push the tip carefully onto the end of a 2 cm 3 graduated glass pipette. Clamp a plastic syringe, 10 cm 3 capacity, above the adapted pipette, as shown in the picture, and connect the two with rubber, plastic, or silicone tubing. Because the diameters of the syringe nozzle and of the top of the pipette may be quite different, two pieces of tubing, one to fit each end, will probably be needed; these can then be joined by an adaptor. A suitable adaptor can be made by cutting the lower end off a 1 cm 3 plastic syringe, such that the syringe body diameter fits the wider tubing, and the syringe tip fits the narrower tubing. (See the diagram and photograph.)
It is possible for students to build their own microscale titration apparatus from supplied components, but this is likely to take the students more time than the titration itself! For that reason, it is probably preferable to prepare a class set of these in advance.
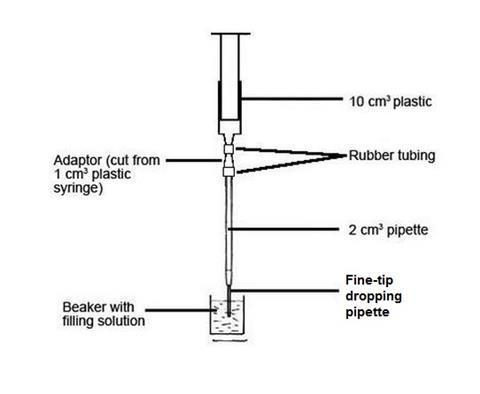
A diagram of the set-up for the microscale titration, illustrating the use of an adaptor joining the two main parts of the apparatus
- Clamp the microscale titration apparatus securely in position as in photograph and push the syringe plunger completely down.
- Fill the apparatus with 0.10 M hydrochloric acid as follows. Put about 5 cm 3 of the acid in a 10 cm 3 beaker and place the tip of the apparatus well down into the solution. Raise the syringe plunger slowly and gently, making sure no air bubbles are drawn in. Fill the pipette exactly to the zero mark. Release the plunger; the level should remain steady.
- Use the 1 cm 3 pipette and pipette filler to transfer exactly 1.0 cm 3 of the sodium hydroxide solution into a clean 10 cm 3 beaker.
- Add one drop (no more!) of phenolphthalein indicator solution to the sodium hydroxide solution.
- Adjust the position of the microscale titration apparatus so that the tip is just below the surface of the sodium hydroxide and indicator solution in the beaker
- Titrate the acid solution into the alkali by pressing down on the syringe plunger very gently, swirling to allow each tiny addition to mix and react before adding more.
- Continue until the colour of the indicator just turns from pink to permanently colourless.
- Record the volume of hydrochloric acid added at that point.
- Repeat the titration until you get reproducible measurements – that is, the volume required is the same in successive titrations.
Calculating the concentration of sodium hydroxide solution
- The equation for the neutralisation reaction is: HCl(aq) + NaOH(aq) → NaCl(aq) + H 2 O(l) From the equation you can see that one mole of hydrochloric acid reacts with one mole of sodium hydroxide.
- What was the reliable value for the volume of hydrochloric acid solution needed? Let us call this value V cm 3 .
- Calculate the number of moles of hydrochloric acid in this volume using the formula: V/1000 x C , where C is the concentration of the hydrochloric acid in M.
- How many moles of sodium hydroxide were therefore present in the original 1 cm 3 of sodium hydroxide solution placed in the beaker?
- Now calculate how many moles of sodium hydroxide would have been present in 1000 cm 3 . This is the concentration of the sodium hydroxide solution in mol dm – 3 .
Teaching notes
This microscale technique minimises apparatus and chemical requirements, and takes less time to perform than titration on the usual scale. Although the solutions used do present minor hazards, the use of such small quantities reduces risks from those hazards to very low levels. Students should nevertheless take all usual precautions in handling these solutions. The main risk is from misuse of the syringe or pipettes, especially if containing hazardous solutions.
The technique also makes the point that quantitative chemical experimentation does not always have to be performed on the traditional ‘bucket’ scale at school level.
Additional information
This is a resource from the Practical Chemistry project , developed by the Nuffield Foundation and the Royal Society of Chemistry. This collection of over 200 practical activities demonstrates a wide range of chemical concepts and processes. Each activity contains comprehensive information for teachers and technicians, including full technical notes and step-by-step procedures. Practical Chemistry activities accompany Practical Physics and Practical Biology .
© Nuffield Foundation and the Royal Society of Chemistry
- 11-14 years
- 14-16 years
- 16-18 years
- Practical experiments
- Acids and bases
- Quantitative chemistry and stoichiometry
Specification
- Use of: titration apparatus including at least class B bulb pipettes and burettes (volume), burette holder/clamp and white tile;
- 2.6.4 carry out acid–base titrations using an indicator and record results to one decimal place, repeating for reliability and calculating the average titre from accurate titrations (details of the practical procedure and apparatus preparation are…
- carry out an acid-base titration to determine the concentration of acid/base, the degree of hydration in a hydrated metal carbonate and the percentage of ethanoic acid in vinegar;
- 1.9.2 demonstrate understanding of the techniques and procedures used when experimentally carrying out acid-base titrations involving strong acid/strong base, strong acid/weak base and weak acid/strong base, for example determining the degree of…
- 8. Investigate reactions between acids and bases; use indicators and the pH scale
- AT d: Use laboratory apparatus for a variety of experimental techniques including: titration, using burette and pipette, distillation and heating under reflux, including setting up glassware using retort stand and clamps, qualitative tests for ions and…
- Titrations of acids with bases.
- 11. be able to calculate solution concentrations, in mol dm⁻³ and g dm⁻³, including simple acid-base titrations using a range of acids, alkalis and indicators. The use of both phenolphthalein and methyl orange as indicators will be expected.
- 6. use acid-base indicators in titrations of weak/strong acids with weak/strong alkalis
- di) use of laboratory apparatus for a variety of experimental techniques including: i) titration, using burette and pipette
- f) use of acid–base indicators in titrations of weak/ strong acids with weak/strong alkalis
- 2a Determination of the reacting volumes of solutions of a strong acid and a strong alkali by titration.
- The volumes of acid and alkali solutions that react with each other can be measured by titration using a suitable indicator.
- Students should be able to: describe how to carry out titrations using strong acids and strong alkalis only (sulfuric, hydrochloric and nitric acids only) to find the reacting volumes accurately
- 3.18 Describe how to carry out an acid-alkali titration, using burette, pipette and a suitable indicator, to prepare a pure, dry salt
- 5.9C Carry out an accurate acid-alkali titration, using burette, pipette and a suitable indicator
- 6 Titration of a strong acid and strong alkali to find the concentration of the acid using an appropriate pH indicator
- C5.4.7 describe and explain the procedure for a titration to give precise, accurate, valid and repeatable results
- C5.3.6 describe and explain the procedure for a titration to give precise, accurate, valid and repeatable results
- PAG 6 Titration of a strong acid and strong alkali to find the concentration of the acid using an appropriate pH indicator
- C5.1b describe the technique of titration
- determining the volumes of acids and alkalis required for neutralisation to occur
- phenolphthalein
- In an acid-base titration, the concentration of the acid or base is determined by accurately measuring the volumes used in the neutralisation reaction. An indicator can be added to show the end-point of the reaction
- titration is used to accurately determine the volumes of solution required to reach the end-point of a chemical reaction.
- (d) the neutralisation of dilute acids with bases (including alkalis) and carbonates
- (e) neutralisation as the reaction of hydrogen ions with hydroxide ions to form water H⁺(aq) + OH⁻(aq) → H₂O(l)
- (j) titration as a method to prepare solutions of soluble salts and to determine relative and actual concentrations of solutions of acids/alkalis
- carrying out and representing mathematical analysis
- (e) neutralisation as the reaction of hydrogen ions with hydroxide ions toform water H⁺(aq) + OH⁻(aq) → H₂O(l)
- (f) acid-base titrations
- (j) concept of stoichiometry and its use in calculating reacting quantities, including in acid-base titrations
Related articles
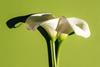
How science can make burial, cremation and memorial greener
2023-11-13T06:00:00Z By Kit Chapman
Does alkaline hydrolysis offer a more sustainable approach?
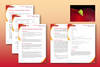
Pesticides and agricultural productivity calculations | 14–16 years
By Harry Lord
Link food security to yield, percentage yield and atom economy
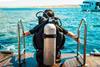
How to teach gases at 14–16
2023-06-06T08:10:00Z By Tim Jolliff
Use these classroom activities and tips to boost your students’ understanding of quantitative chemistry
1 Reader's comment
Only registered users can comment on this article., more experiments.
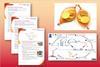
‘Gold’ coins on a microscale | 14–16 years
By Dorothy Warren and Sandrine Bouchelkia
Practical experiment where learners produce ‘gold’ coins by electroplating a copper coin with zinc, includes follow-up worksheet
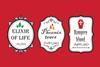
Practical potions microscale | 11–14 years
By Kirsty Patterson
Observe chemical changes in this microscale experiment with a spooky twist.
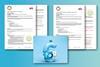
Antibacterial properties of the halogens | 14–18 years
By Kristy Turner
Use this practical to investigate how solutions of the halogens inhibit the growth of bacteria and which is most effective
- Contributors
- Email alerts
Site powered by Webvision Cloud

Want to create or adapt books like this? Learn more about how Pressbooks supports open publishing practices.
9 Acid-Base Titration Curves
To compare and contrast acid-base titration curves for different acid-base titrations.
Learning Outcomes
- Identify the features of different types of acid-base titration curves.

- Select appropriate indicators for use in a titration.
Textbook Reference
Tro, Chemistry: Structure and Properties, Ch. 17.4
The write-up below covers merely the very basics of what the lab entails; more details are covered in your textbook which you are expected to refer to.
Introduction

Acid-base titrations involve the dropwise addition of one solution-phase reagent (using a buret) into a carefully measured amount of another reagent (in an Erlenmeyer flask). There are three main classes of titrations that can be studied:
- Strong acid-strong base
- Weak acid-strong base
- Weak base-strong acid
In this experiment, you will examine the titration curves for each of these types of titrations. In each case, we are studying an acid-base neutralization reaction.
Equivalence Points
One particularly important point in a titration curve is the equivalence point. The equivalence point is the point in the acid-base titration at which each molecule of HA is neutralized by one OH – ion, causing the acid to be converted to its conjugate base. Or, if a base is the analyte, the point where each molecule of the base A – is neutralized by one H 3 O + ion, causing the base to be converted to its conjugate acid.
Types of Titration Curves and its Features
Strong acid-strong base titrations.
When titrating a strong acid, such as hydrochloric acid with sodium hydroxide, you are reacting the HCl directly with NaOH

The titration curve is shown below. In this case, there is a very sharp transition in pH at the equivalence point. Furthermore, as the salt formed in the neutralization reaction is neutral, the solution at the equivalence point is neutral.
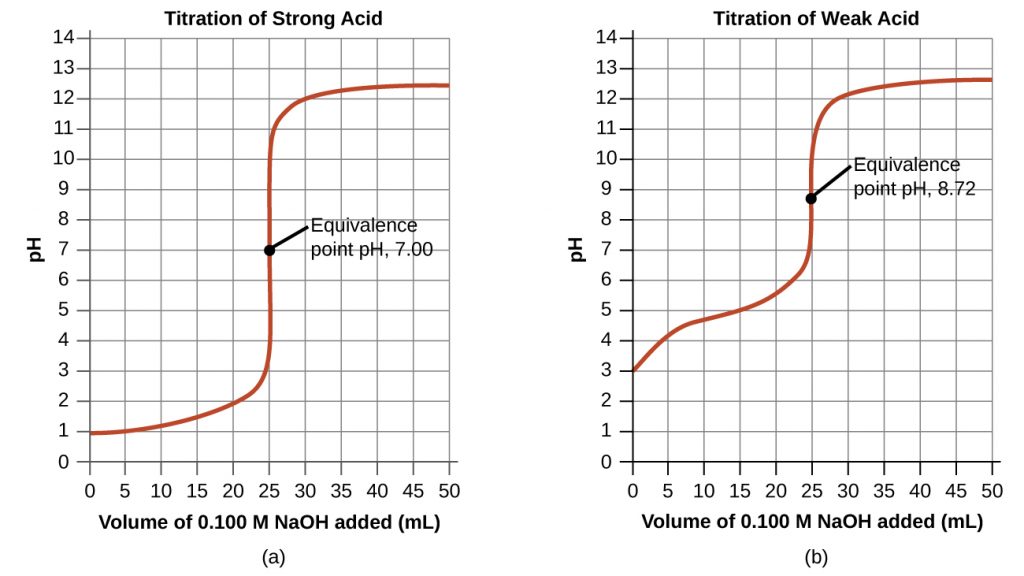
Weak Acid-Strong Base Titrations
An example of such a titration would be acetic acid (CH 3 COOH) with sodium hydroxide. In this case, the reaction is

or, for the net ionic equation,

In this case, there is one important aspect to note. As the neutralization takes place, there will be appreciable amounts of both CH 3 COOH, a weak acid, and CH 3 COO – , its conjugate base, present in the solution. This mixture is a buffer solution . As a result, a gradual transition in the pH is observed in this solution as shown in the figure above, as opposed to the very sharp transition observed for strong acid-strong base solutions.
At the equivalence point, all of the CH 3 COOH is converted to CH 3 COO – , a weak base. Therefore, the solution is basic at the equivalence point.
Beyond this point, as additional OH – is added, a sharp transition in the pH curve is observed.
Importance of the Half-Equivalence Point: Finding
To identify the half-equivalence point of a solution, you start by identifying the equivalence point of the titration curve and then halving the volume. Then, you find the point on the curve where this volume of the titrant was added.

As mentioned before, in this region the mixture behaves as a buffer solution, where we can use the Henderson-Hasselbalch equation
![Rendered by QuickLaTeX.com \begin{equation*} \mbox{pH} = \mbox{p}K_a + \log_{10} \left(\frac{[\ce{A-}]}{[\ce{HA}]}\right) \end{equation*}](https://iu.pressbooks.pub/app/uploads/quicklatex/quicklatex.com-1da79b5e0c39a77cd5f8e976a0d113f9_l3.png)
From above, we saw that the p K a of the analyte in the weak acid-strong base titration curve above is 4.9. Therefore,

Weak Base-Strong Acid Titration Curves
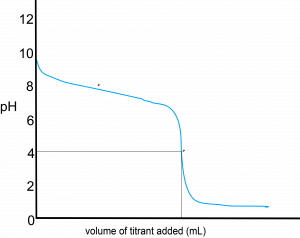
This type of titration would yield a curve with decreasing pH, given that you’re neutralizing base with a strong acid:

where HA is the conjugate acid of the original weak base A – and is therefore a weak base. A few features can be found … the flip side of the weak acid-strong base titration curve:
- At the equivalence point, we have a solution of HA, the weak acid. As a result, you would have an acidic solution (pH < 7) at the equivalence point.
- Before you hit the equivalence point, you would have a mixture of HA and A – . Similarly, you would have a buffer solution in that region, leading to the gradual decrease in pH there.
Similarly, therefore, we can use the half-equivalence point to find the K b of the weak base. In this case, the pH is the p K a of the conjugate acid of the weak base.

Experimental Procedure
- Before doing this experiment, please review the section on Using ChemCollective Virtual Labs .
- Record, in handwriting on your regular lab notebook, your purpose , procedure , and results similar to a face to face experiment.
- Include a set of screenshots as directed below.
- If you think that a change to the procedure would help, then feel free to do so. Just make sure it is documented in your lab notebook. Feel free to consult with your lab instructor if you’re unsure, but remember there are no safety implications.
- A separate report form will be provided in MS Word format.
Strong Acid-Strong Base Titration
You will use the ChemCollective Strong Acid and Base Problems virtual lab to perform this virtual titration. For further details on how to do this on the electronic platform, please see: Using ChemCollective Virtual Labs .
- Obtain a buret (from Glassware > Other ) and the 0.1 M sodium hydroxide.
- Fill the buret with the 0.1 M sodium hydroxide to about the 10.00mL mark. Read and record the volume reading on the buret, paying attention to the (a) direction that the numbers are graduated and (b) the number of significant figures.Data
- Obtain a 0.1 M hydrochloric acid solution, a 10 mL volumetric pipet, and a 250 mL Erlenmeyer flask.
- Withdraw 10.00 mL of the hydrochloric acid solution into the pipet. When this is done, obtain a screenshot of the interface .
- Move the pipet away from the flask of 0.1 M hydrochloric acid solution and put it on top of the empty 250 mL Erlenmeyer flask. Dispense the hydrochloric acid from the pipet into the Erlenmeyer flask.
- Select the Erlenmeyer flask and record the pH of the solution.
- Put the buret on top of the Erlenmeyer flask, and dispense approximately 0.5 mL of the sodium hydroxide solution into the Erlenmeyer flask. Record the volume in the buret. Record the pH of the solution in the Erlenmeyer flask.
- Repeat step 7 until the pH remains relatively constant.
A video demonstration of how to do this experiment (somewhat badly) is shown below:
Data Analysis
To plot the titration curve, first find the total volume of NaOH added at each step of the titration above. Then, plot the pH as a function of the volume of sodium hydroxide added. It’s not critical how the curve is fitted; however, it is important that you have a rather dense set of gridlines. How this can be done with Excel is demonstrated below:
Weak Acid-Strong Base Titration
You will perform an unknown weak acid-strong base titration using the ChemCollective Unknown Acid and Base Problem simulation.
Complete the experiment following the same procedure as for the strong acid-strong base titration, except you need to use the unknown acid instead of hydrochloric acid.
Take a screenshot of your titration setup at some point during the titration procedure (i.e. with the buret above the Erlenmeyer flask).
Make a plot of the acid-base titration curve as above.
Finding the Molarity of the Unknown Solution
To find the molarity of the unknown solution, remember that at the equivalence point the number of moles of acid initially present equals the number of moles of base added for a monoprotic acid. Therefore, you can perform a stoichiometry problem by using the definition of molarity, similar to what is described in Tro, Chemistry – Structures and Properties , 2nd Ed, Ch. 8.7. An example of how this is done is explained in the following video:
https://iu.mediaspace.kaltura.com/id/1_7pl9o19p?width=400&height=285&playerId=26683571
pH at the Equivalence Point
Remember that at the equivalence point, all of the acid would have converted to its conjugate base. We therefore have a solution of the conjugate base of the weak acid. This is therefore a weak base problem.

- M 1 is the molarity of the original unknown solution, found as described above.
- V 1 is the volume of the unknown solution in the Erlenmeyer flask (analyte).
- M 2 is the molarity of the diluted solution
- V 2 is the volume of the mixture in the Erlenmeyer flask including all components, which we will approximate to be the sum of the volumes of acid and base added.

- This then becomes a weak base pH calculation similar to that calculated in the regular course. As a refresher, the following video outlines how to solve this type of problem:
https://iu.mediaspace.kaltura.com/id/1_ldt19iip?width=400&height=285&playerId=26683571
Weak Base-Strong Acid Titration
Using the ChemCollective Unknown Acid and Base Problem simulation that you used for the previous part, repeat the procedure but use the unknown base as the analyte and the 0.1 M HCl as the titrant instead. Similar approaches to find the pH at the equivalence point to the above can be found, except that this would now be an acidic salt.
Virtual Chemistry Experiments Copyright © by Yu Kay Law. All Rights Reserved.
Share This Book
Master Chemistry
Acid base titration-Working principle, Process, types and indicators
Learning objectives.
Table of Contents
In this article, the author has explained Acid base titration, its working principle types and process of acid base titration
Acid-base reactions are used to find the amount of acidic or basic substances. The solution with unknown molarity is the analyte. The analyte will react with the solution with known molarity.
First lets start with a simple definition of titration.
What is titration?
Titration is a chemical analysis method used to determine the concentration of a particular analyte.
Titration, also known as volumetric analysis, is a method in which the titrant is added from a burette until the reaction is complete, and an indicator is usually employed to mark the endpoint of the reaction.
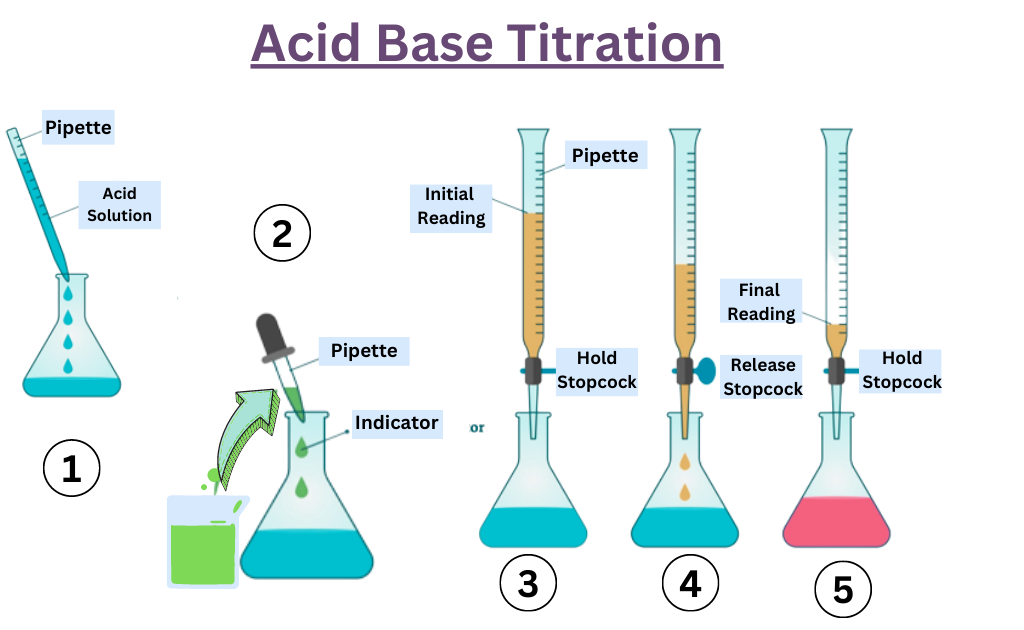
Theory of titration
Titration is a method used to determine the unknown concentration of a substance in a solution. It involves the gradual addition of a known concentration of a solution, called the titrant , to a known volume of the substance being analyzed, called the analyte or titrand. The point at which the two solutions are chemically balanced is called the endpoint of the titration.
Types of titration
There are several types of titrations, including acid-base titrations, redox titrations, and complexometric titrations. Acid-base titrations involve the reaction between an acid and a base to form a salt and water. Redox titrations involve the transfer of electrons from one species to another. Complexometric titrations involve the formation of a complex between the analyte and the titrant.
How endpoint of titration is measured?
The endpoint of the titration can be determined using a variety of methods, including visual indicators, conductivity measurements, or pH measurements. The endpoint is typically indicated by a change in the color of the solution, a change in the electrical conductivity of the solution, or a change in the pH of the solution.
Titrations are widely used in various fields, including chemistry, biology, and environmental science, to determine the concentration of a variety of substances in solutions.
important terms in titration
Term | Definition |
---|---|
Analyte | The substance whose concentration is being determined in a titration. |
Titrant | The solution of known concentration that is added to the analyte in a titration. |
Endpoint | The point at which the chemical reactions between the analyte and the titrant are balanced. This is usually indicated by a change in the color of the solution, a change in the electrical conductivity of the solution, or a change in the pH of the solution. |
Equivalence point | The point at which the number of moles of the titrant added to the analyte is equal to the number of moles of the analyte present. At this point, the volume of titrant added can be used to calculate the concentration of the analyte. |
Burette | A piece of laboratory equipment used to measure and dispense precise volumes of a solution. The volume of titrant added to the analyte can be determined by reading the volume on the burette at the beginning and end of the titration. |
Titration curve | A graph of the volume of titrant added versus the concentration of the analyte. The titration curve typically has a distinct inflection point at the equivalence point, which can be used to determine the concentration of the analyte. |
Indicators | Substances that are used to indicate the endpoint of a titration. Indicators are typically chosen based on their ability to undergo a visible color change at a specific pH. |
I have also written few titration related other articles, I am sure you would learn from these as well.
- What are precautions during titration process in lab
- Uses of titration in industries
- Why is titration important in chemistry?
What is acid-base titration?
An acid-base titration is a quantitative analysis method used to determine the concentration of an acid or base by neutralizing the acid or base with a known concentration standard solution.
The concentration of a solution can be determined by knowing the acid and base dissociation constant. If the solution concentration is known, a titration curve can be used.
Principle of acid-base titration
In the theory of acid-base titration, the principle involves using a burette and pipette to determine the concentration of an acid or basic.
An indicator is a dye added to a solution to change its color. It is dissolved in the sample solution and can be used to detect the end of the titration.
Importance of acid base titration
Acid-base titrations are important because they allow for the precise determination of the concentration of an acid or a base in a solution. This information is useful in a variety of fields, including chemistry, biology, and environmental science.
In chemistry, acid-base titrations are used to determine the concentration of acids and bases in solutions, which is important for understanding chemical reactions and for the preparation of standard solutions.
In biology, acid-base titrations are used to determine the pH of solutions, which is important for understanding the behavior of enzymes and other biological molecules.
In environmental science, acid-base titrations are used to determine the acidity or basicity of water, which is important for understanding the impact of acid rain on aquatic ecosystems.
Overall, acid-base titrations are a powerful tool for understanding and quantifying the concentration of acids and bases in solutions, and they have many important applications in various fields.
Acid base titration and volumetric analysis
Acid-base titrations are a type of volumetric analysis, which is a method of chemical analysis that involves the measurement of volume in order to determine the concentration of a substance in a solution. In an acid-base titration, a solution of known concentration (called the titrant) is gradually added to a known volume of the substance being analyzed (called the analyte). The point at which the two solutions are chemically balanced is called the endpoint of the titration.

Indicators used in acid-base titration:
The acid strength of the indicator is important in determining the pH range. The indicator changes color from acid to base when it’s in the range of pH values.
The acid form can only be seen at the highest pH, the base form can only be seen at the lowest. Since the indicator doesn’t change color at certain pH levels, it’s not sensitive to changes outside of its range.
Selection of indicator in acid base titration
There are several factors to consider when selecting an indicator for an acid-base titration:
- The pH range of the titration: The indicator should have a color change within the pH range of the titration. For example, if the titration involves a strong acid and a strong base, the pH range will be wide, and an indicator with a wide range, such as bromothymol blue, can be used. If the titration involves a weak acid and a strong base, the pH range will be narrow, and an indicator with a narrow range, such as phenolphthalein, can be used.
- The desired endpoint: The indicator should undergo a color change at the desired endpoint of the titration. For example, if the endpoint of the titration is the point at which the acid and base are neutralized, an indicator with a pK value close to 7, such as bromocresol green, can be used. If the endpoint of the titration is the point at which the acid and base are in a specific ratio, an indicator with a pK value close to the desired ratio, such as methyl red, can be used.
- The sensitivity of the indicator: The indicator should undergo a noticeable color change at the endpoint of the titration. Some indicators, such as thymol blue, have a sharp color change at the endpoint, while others, such as methyl orange, have a more gradual color change.
Overall, the selection of an indicator for an acid-base titration is based on the pH range of the titration, the desired endpoint, and the sensitivity of the indicator.
Classification of acid-base titration indicators
Acid-base indicators are generally classified into below listed three groups.
1. The phthaleins and sulphophthaleins: example-phenolphthalein indicator
2. Azo indicators: example- methyl orange indicator
3. Triphenylmethane indicators: for example- malachite green indicator
Specific indicator for different acid-base titration:
Different indicators are used in acid-base titrations. The selection of indicators depends on the type of titration and the range of the reaction.
- Strong acid-strong base: Phenolphthalein is generally preferred due to color change seen more easily.
- Weak acid-strong base: Phenolphthalein is more proffered for this titration because it changes sharply at the equivalence point.
- Strong acid-weak base: Methyl orange is more proffered for this titration because it changes sharply at the equivalence point.
- Weak acid-weak base: Because a vertical portion of the curve above two pH units is required, there is no indication is suitable for this titration.
Types of acid-base titration with their examples:
There are four different types of acid-base titration, which include strong acid-strong base, weak acid-strong base, strong acid-weak base, and weak acid-weak base.
Strong acid-strong base:
It’s one of the easiest titrations to perform among the four forms of acid-base titrations. It involves the dissociation of a strong acid and a strong base in water, which results in a strong acid-strong base neutralization reaction. The equivalency point is reached when the moles of acid and base are the same and the pH is zero.
Weak acid-strong base
The direct transfer of the protons from the weak acid to the hydroxide ion is possible in this type of titration. The acid and base react in a one-to-one ratio when reacting a weak acid with a strong base. At the equivalent point of a weak acid–strong base titration, the pH is larger than 07.
Strong acid-weak base
The acid and base will react to form an acidic solution. A conjugate acid is formed which reacts with water to form a hydronium ion. At the point of a strong acid-weak base titration, the pH is less than 7.
Weak acid-weak base
The shape of a weak acid’s or base’s titration curve depends on the acid’s or base’s identity and the associated acid ionization constant. In the titration of a weak acid or a weak base, the pH gradually changes around the equivalence point, which is greater or less than 7.
Below are examples of these titrations.
- Hydrochloric acid (HCl) and sulphuric acid (H 2 SO 4 ) are two examples of strong acids.
- Acetic acid (CH 3 COOH) and formic acid (CH 2 O 2 ) are two examples of weak acids.
- Sodium hydroxide (NaOH) and potassium hydroxide (KOH) are two examples of strong bases.
- Ammonia and methylamine are two examples of weak bases.
Apparatus and Process of titration
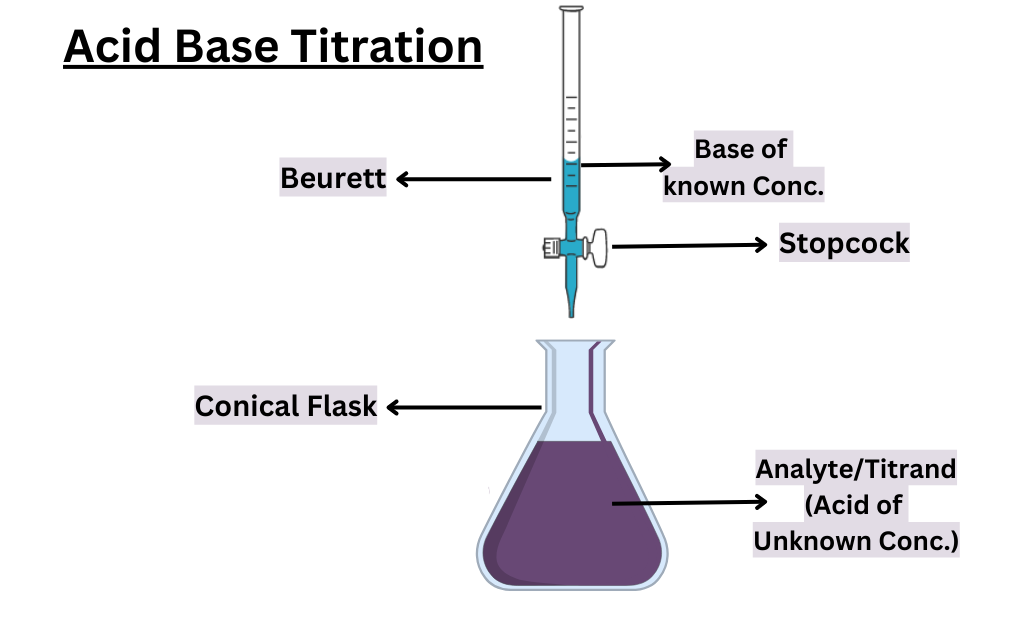
First of all we need to arrange the following apparatus to perform acid-base titration.
- Conical flask
- burette stand
- wash bottle
- unknown solution
- Standard solution
Then we need to clean all the apparatus using distilled water.
After then we need to fill the burette with a standardized solution, accurately measure the volume of the analyte, and add in the conical flask, also add a few drops of indicator using the pipette.
Titrate it with the standardized solution until the indicator changes color. When the indicator permanently changes the color, the endpoint reaches.
Repeat the titration at least three more times and record the initial and final readings in the observation table and calculate the value.
How does titration work?
Titration is a method used to determine the concentration of a substance in a solution. It involves adding a known concentration of a solution (titrant) to a known volume of the substance (analyte) and measuring the volume of titrant at the point of chemical balance (endpoint).
What is the major purpose of acid base titration?
The major purpose of acid-base titration is to determine the concentration of an acid or a base in a solution.
Which indicator is used in acid base titration?
An indicator is chosen for an acid-base titration based on the pH range of the titration, the desired endpoint, and the sensitivity of the indicator. Some commonly used indicators include bromothymol blue, phenolphthalein, bromocresol green, methyl red, thymol blue, and methyl orange.
is titration only for acids and bases?
No, titration can be used to determine the concentration of various substances, including acids, bases, and other types of molecules. There are several types of titrations, including acid-base, redox, and complexometric.
How do acid base indicators work?
Acid-base indicators change color at a specific pH and are used to indicate the endpoint of a titration. They are chosen based on the desired endpoint and the pH range of the titration, and their color change at the endpoint is used to determine the concentration of the acid or base in the solution.
Why is phenolphthalein an appropriate indicator for a weak acid-strong base titration?
Phenolphthalein is a suitable indicator for a weak acid-strong base titration because it has a narrow pH range and undergoes a sharp color change at the endpoint.

In order to continue enjoying our site, we ask that you confirm your identity as a human. Thank you very much for your cooperation.
If you're seeing this message, it means we're having trouble loading external resources on our website.
If you're behind a web filter, please make sure that the domains *.kastatic.org and *.kasandbox.org are unblocked.
To log in and use all the features of Khan Academy, please enable JavaScript in your browser.
Chemistry archive
Course: chemistry archive > unit 12.
- Acid–base titrations
- Worked example: Determining solute concentration by acid–base titration
Titration of a strong acid with a strong base
- Titration of a strong acid with a strong base (continued)
- Titration of a weak acid with a strong base
- Titration of a weak acid with a strong base (continued)
- Titration of a weak base with a strong acid
- Titration of a weak base with a strong acid (continued)
- 2015 AP Chemistry free response 3b
- 2015 AP Chemistry free response 3c
- 2015 AP Chemistry free response 3d
- 2015 AP Chemistry free response 3e
- 2015 AP Chemistry free response 3f
- Titration curves and acid-base indicators
- Redox titrations
- Introduction to titration

Want to join the conversation?
- Upvote Button navigates to signup page
- Downvote Button navigates to signup page
- Flag Button navigates to signup page

Video transcript
Experiment 3 lab.docx - Experiment 3 - Analysis of Antacids...
- University of Toronto, Mississauga
- BarristerProton12460
End of preview
Recently submitted questions.
- Search Menu
- Sign in through your institution
- Chemical Biology and Nucleic Acid Chemistry
- Computational Biology
- Critical Reviews and Perspectives
- Data Resources and Analyses
- Gene Regulation, Chromatin and Epigenetics
- Genome Integrity, Repair and Replication
- Methods Online
- Molecular Biology
- Nucleic Acid Enzymes
- RNA and RNA-protein complexes
- Structural Biology
- Synthetic Biology and Bioengineering
- Advance Articles
- Breakthrough Articles
- Special Collections
- Scope and Criteria for Consideration
- Author Guidelines
- Data Deposition Policy
- Database Issue Guidelines
- Web Server Issue Guidelines
- Submission Site
- About Nucleic Acids Research
- Editors & Editorial Board
- Information of Referees
- Self-Archiving Policy
- Dispatch Dates
- Advertising and Corporate Services
- Journals Career Network
- Journals on Oxford Academic
- Books on Oxford Academic
Article Contents
Introduction, materials and methods, data availability, supplementary data, acknowledgements, nanopores map the acid-base properties of a single site in a single dna molecule.

- Article contents
- Figures & tables
- Supplementary Data
Drew C Smith, Christopher A Thomas, Jonathan M Craig, Henry Brinkerhoff, Sarah J Abell, Michaela C Franzi, Jessica D Carrasco, Shuichi Hoshika, Steven A Benner, Jens H Gundlach, Andrew H Laszlo, Nanopores map the acid-base properties of a single site in a single DNA molecule, Nucleic Acids Research , 2024;, gkae518, https://doi.org/10.1093/nar/gkae518
- Permissions Icon Permissions
Nanopores are increasingly powerful tools for single molecule sensing, in particular, for sequencing DNA, RNA and peptides. This success has spurred efforts to sequence non-canonical nucleic acid bases and amino acids. While canonical DNA and RNA bases have p K a s far from neutral, certain non-canonical bases, natural RNA modifications, and amino acids are known to have p K a s near neutral pHs at which nanopore sequencing is typically performed. Previous reports have suggested that the nanopore signal may be sensitive to the protonation state of an individual moiety. We sequenced ion currents with the MspA nanopore using a single stranded DNA containing a single non-canonical DNA base ( Z ) at various pH conditions. The Z -base has a near-neutral p K a ∼ 7.8. We find that the measured ion current is remarkably sensitive to the protonation state of the Z -base. We demonstrate how nanopores can be used to localize and determine the p K a of individual moieties along a polymer. More broadly, these experiments provide a path to mapping different protonation sites along polymers and give insight in how to optimize sequencing of polymers that contain moieties with near-neutral p K a s.

Nanopores are a powerful tool for investigating single molecules. In the last decade, nanopore technologies have reached major sensing milestones. Nanopores are now regularly used to resolve single nucleotides in DNA and RNA ( 1–3 ) and detect DNA and RNA base modifications such as methylation ( 4 ) and pseudouridine ( 5 ). Nanopores have also been used to decode synthetic polymers designed to store digital data ( 6 ), detect toxins at subnanomolar concentrations and detect pharmaceutical enantiomers ( 7 , 8 ). Recently, nanopores were shown to be sensitive to single amino acid substitutions within a peptide chain, including detection of post-translational modifications on single peptide molecules ( 9 , 10 ). Sequencing and sensing with nanopores are achieved by measuring how the trapped molecule of interest blocks the ion current through the pore. When measuring heterogeneously charged polymers such as peptides, the charge of molecules plays a significant role in the nanopore signal. Because of this, the occupation status of various protonation sites may significantly affect the nanopore signal. The standard bases A, C, G, and T have p K a s > 9, so there has been relatively little investigation of the effect of pH on the nanopore signal ( 11 , 12 ). However, as nanopore sequencing is extended to RNA modifications, peptides, and nonstandard bases that contain moieties with near-neutral p K a s ( 13–15 ), understanding how the change in charge caused by protonation affects the nanopore ion currents will be necessary. Previous nanopore experiments using α-hemolysin nanopores suggested that protonation detection would be possible ( 11 , 12 ). Here, we used the MspA nanopore combined with the motor enzyme Hel308 for translational control, to build on these experiments. We show direct detection of the occupancy of a single protonation site of the non-canonical ‘ Z’ -base, (6-amino-3-(1′-β- d -2′-deoxyribofuranosyl)-5-nitro-1 H -pyridin-2-one, within a single DNA molecule (Figure 1A ) ( 16 , 17 ).

Z base and experimental setup. ( A ) The chemical structure of the Z -base at pH 6 and pH 9. At pH > 7.8, the 3-position of N-H group loses a proton resulting in a net negative charge on Z . ( B ) Schematic of the nanopore experiment with DNA bound to a Hel308 helicase translocating through the pore. As DNA is pulled upward by the helicase, the Z -base moves through the pore constriction, changing the measured ion current depending on the protonation state. ( C ) An ion current trace of the DNA strand at pH 7. The data are filtered and downsampled to 333 Hz for clearer visualization. ( D ) A consensus built from the current levels of many ion current traces; the x-axis is marked by the helicase's half-nucleotide-long steps with the DNA sequence most centered in the MspA constriction indicated. The ion current error bars indicate standard deviations.
Nanopore sequencing experiments are typically performed near neutral pH conditions to enable optimal function of the motor enzyme. Previous nanopore experiments have varied salt concentrations and pH to optimize the signal-to-noise ratio ( 12 , 18 ), but the canonical DNA bases all have p K a far from neutral, preventing demonstration of protonation effects in canonical DNA in typical sequencing configurations. However, the non-canonical Z base (used in expanded genetic alphabets) has a p K a of 7.8 ( 19 , 20 ) at its N–H group at 3-position (Figure 1A ). Therefore, the Z base provides an opportunity to study protonation in a nanopore sequencing setup. Here we observe a DNA strand containing a single Z using the nanopore at pH conditions ranging from pH 6–9. As we increased pH, we directly measured an increase in ion current when the Z base was in the pore constriction.
Protein and enzyme
At each pH condition, a minimum of three separate nanopore experiments were performed to ensure reproducibility (see Supplementary Table S3 for sample sizes). Mycobacterium smegmatis porin A (MspA) is an extremely robust nanopore that maintains its structure over a wide range of salt conditions and pH from 2 to 14 ( 21 ). We used the M2-NNN mutant of MspA (accession number CAB56052.1) that was specifically engineered for nanopore sequencing ( 22 ). MspA has no residues near the constriction with p K as within or near the pH range of 6–9. To control DNA translocation through the pore, we used Hel308 helicase from Thermococcus gammatolerans EJ3 (accession number WP_015858487.1).( 2 )
Pore establishment
Following standard and established procedure( 2 , 23 , 24 ), a single MspA nanopore was inserted in a 1,2-di- O -phytanyl- sn -glycero-3-phosphocholine (DOPHPC) lipid bilayer. Lipids were ordered from Avanti Polar Lipids. Briefly, a DOPHPC bilayer is formed over an aperture via the ‘painting method’( 24 ). 0.2 μl of 60 μM MspA in 0.1% by volume OPOE detergent is then added to and mixed within the cis well. After some time, individual MspA molecules begin inserting into the bilayer as noted by quantized jumps in ion current from zero up to integer multiples of the current characteristic of a single MspA pore. When a single pore is present, the operator then rapidly flushes fresh buffer through the cis compartment removing the excess MspA and leaving a single pore embedded within the bilayer. For more information, please refer to citations 2, 23 and 24.
Read strand: (5′ to 3′)
/phos/TTTTTTTTTTTTCTTAGATTCCAATGTCA Z CGTCACGGCAGACAACTCATGCAGGAAGCATTCTC
Complementary strand: (5′ to 3′)
CCTGCATGAGTTGTCTGCCGTGXXXX/chol/
X = 18-carbon spacer, /chol/= 3′ cholesterol tag, /phos/= 5′ phosphate
The DNA template strand containing the Z base was produced by using AEGIS phosphoramidites (Firebird Biomolecular Sciences LLC, www.firebirdbio.com ) through solid-phase oligonucleotide synthesis on an ABI 394 instrument. Oligonucleotides were purified by 10% denatured PAGE and desalted on a C18 Sep-Pak cartridge ( 14 ).
The template strand and complement were annealed (at 20 μM each) by heating to 96°C for 3 min and then slowly cooling to 4°C at a rate of –10°C/min. The duplex had a 10-base-long ssDNA overhang at the 3′ end and a 33-base-long ssDNA overhang at the 5′ end.
Nanopore experiments
A single MspA nanopore was embedded in a lipid membrane providing the only electrical connection between two reservoirs of electrolyte buffer, the cis and trans reservoirs ( 2 ) (Figure 1B ). A Ag/AgCl electrode in each reservoir applied a voltage across the membrane causing an ion current to flow through the pore, and the resulting ion current was measured.
DNA and Hel308 helicase are added to the cis reservoir at a final concentration of 10 nM and 7μg/ml, respectively. The cholesterol tag on the complementary strand of the DNA inserts into the bilayer, localizing DNA to the bilayer and thereby increasing the rate at which DNA molecules are drawn into the nanopore. The setup was heated to 37°C to promote helicase binding to DNA and to improve helicase velocity and overall event rate.
Hel308 binds to the ssDNA 3′ end of the template strand. The free 5′ single-stranded end is drawn into the pore by the applied voltage. The complementary strand is sheared off by the pore because only ssDNA fits through the pore constriction. The template strand is pulled through the pore until the bound Hel308 lands on the pore rim. Hel308 then draws the DNA out of the pore in discrete half-nucleotide steps, moving the DNA from 3′ to 5′ through MspA’s constriction( 25 , 26 ). The DNA bases within and adjacent to the pore constriction determine the blockage of ion current ( 25 ).
Four different electrolyte buffers were prepared at different pH conditions: pH 6, 7, 8, and 9 (all ± 0.05). In all four buffers, the cis well contained 400 mM KCl, 1000 uM ATP, 10 mM MgCl 2 , 1 mM DTT, and 10 mM buffering agent. The trans well contained 400 mM KCl and 10 mM buffering agent.
The cis well was perfused with fresh buffer and helicase every 45 minutes to prevent ADP accumulation and ATP depletion. Different buffering agents were used for different pH: MES for pH 6, HEPES for pH 7 and pH 8, and CHES for pH 9. These buffering agents are compatible with enzymatic activity; no significant variation in Hel308 activity was observed during experiments. We chose a pH range from 6–9 to isolate the effect of Z protonation, because some of the protonation sites on the canonical bases have p K a ’s above pH 9, for their conjugate assays, and at pH 4–5 ( 27 ).
Data acquisition
Ion currents were measured with an Axopatch 200B amplifier, filtered with a 10 kHz 8-pole Bessel filter, digitized at 50 kHz, and recorded with custom Labview software.
Raw data analysis
Offline, the data were further filtered with a 5 kHz 8-pole Butterworth filter and downsampled to 5 kHz. Figure 1C shows an example ion current trace as a template DNA strand is pulled through the nanopore.
Hel308-controlled DNA translocation events were identified and saved for further analysis. Within these events, discrete current levels were identified using a change-point detection algorithm ( 23 , 28 ). These levels were aligned to a previously measured consensus pattern of current levels ( 2 ) to build unique ion current level consensus sequences for each pH experiment.
Across the experiments, temperature, salt concentration, pore conductance, and electrode offsets varied slightly, resulting in a slightly different overall scale factor between the ion current levels from individual reads. To compensate for these small changes, data of the same pH were calibrated to one another with a linear scale and offset using a section of current levels away from the Z base nucleotides (CTTAGAT) in the template DNA. Using the same section of DNA, consensus data collected at the four pHs were then calibrated to one another. Figure 1D shows the ion current consensus for pH 7. DNA bases most centered within the pore constriction for each ion current level are shown along the x-axis.
Figure 2A shows the consensus ion current pattern with the DNA sequence shown underneath ( Supplementary Figure S1 shows Figure 2A with standard deviation error bars). The base most centered within the pore constriction is shown along the x-axis in the time order that they were observed |$(3^{\prime} \to 5^{\prime})$| . Figure 2B shows the percent difference of the consensus current level sequence at each pH condition compared to the pH 7 consensus. Figure 2C and D compare the measured ion current distributions for six consecutive steps in the region near the Z base and a region containing only canonical bases ( Supplementary Table S1 and Supplementary Text S11 ).
Effect of Z-protonation on ion current. ( A ) Plot of consensus ion current for the template DNA strand at pH 6.00 ± 0.05, 7.00 ± 0.05, 8.00 ± 0.05, and 9.00 ± 0.05. The base centered within the constriction for each current level is displayed along the x-axis in the time order it is read i.e.from 3′ to 5′. Each x-tick corresponds to one of Hel308’s half-nucleotide steps. As the protonation state of Z changes with pH, significant changes in current for ion current steps become apparent when Z is in and around the constriction; little to no changes occur when only canonical bases are in or adjacent to the constriction. Each trace is a consensus of at least 105 reads of the DNA strand at each pH. Each consensus is calibrated by an overall scaling and offset to match a portion of the canonical base region (indicated by the gray bar in panel A) to the pH 8 consensus in order to compensate for small variations in salt concentration, and temperature. Error bars indicate standard errors in the mean ( Supplementary Figure S1 provides the graph with standard deviation). ( B ) Percent difference of ion current from the pH 7 data shown in (A). When Z is centered in the pore there is a ∼10% current increase from pH 6 to 9. ( C ) The ion current PDF of six consecutive steps of Z as it is passed through the pore constriction for each pH condition. Red triangles above and below the sequence indicate which bases are centered in the pore constriction for each graph. ( D ) For comparison, six consecutive steps within the canonical base section of the DNA strand show little pH-dependent variation in measured ion current.
The most notable effect is an increase in ion current when deprotonated Z is centered in the pore constriction. When Z is deprotonated, it is negatively charged (Figure 1A ). An ion current increase due to more negatively charged DNA is consistent with previously published observations of heterogeneously charged polymers within nanopores ( 9 , 18 ), and may be caused by the attraction of current-carrying positive counterions to the high-field constriction of the pore. Modifications of single bases are known to influence multiple current levels ( 1 , 4 , 29 , 30 ). However, the effect can be localized to a single base due to changes in magnitude of the effect. The difference in current due to Z is most clearly observed for about six current levels ( i.e . ∼3 full nucleotide steps) shown in Figures 2B and 3A with Z centered in the pore constriction on the third step ( Z region). It is also observed that, when Z is centered in the pore constriction, the current levels have higher noise, leading to a broader distribution in Figure 2C ( middle ), as compared to when standard bases are within the pore as seen in Figure 2D (see also Supplementary Figure S2 ). This increased noise may be due, in part, to active protonation state switching ( 11 ). The switching rates between protonated and unprotonated are such that individual ion current data points are a time-averaged sampling of the two states, obscuring a bimodal distribution that might be expected if the two states were more long-lived. (see Supplementary Figure S2 for Histograms of the central Z current level raw data and a canonical level).
Current as a function of pH. Each plot shows ion current difference from pH 6, plotted as a function of pH. Plots from left to right are consecutive helicase steps. ( A ) shows ion current differences in the Z region of the DNA strand while ( B ) shows a section of canonical DNA bases. (A) Corresponds to the same current levels plotted in Figure 2C while (B) shows the same current levels as Figure 2D . In (A) column 3, Z is centered in the pore constriction. Fitting a sigmoid to this data (green line), we measure a p K a of 8.05 ± 0.07. This is in agreement with the pK a measured for the Z base in the DNA strand using spectrophotometry of 8.05 ± 0.12 (Figure 4 and Supplementary Table S2 ). Both measurements differ from the previously reported p K a of 7.8( 19 ) for the d Z mononucleotide, which is likely due to interactions with neighboring bases in the DNA strand because the local chemical environment is known to affect the p K a of specific moieties. Lines connecting data points in all other panels are trend lines and not fits.
We also observe changes in ion current when Z is positioned just above or just below the pore constriction, but far enough away that we would not expect Z to contribute directly to the observed ion current. Here, the amplitude of the ion current difference as a function of pH is inverted, with pH 6 now having the highest current. We speculate this effect is also due to the extra negative charge on the Z base when it is deprotonated. The additional charge on deprotonated Z should change the electrophoretic force experienced by the DNA strand and induce a positional shift as it stretches the DNA more. This would in turn alter which DNA bases occupy the pore constriction and result in a change in ion current even when the Z base is not within the pore constriction. This effect has been seen with non-uniformly charged peptides within the pore ( 7 , 9 ) and similar stretching has been observed when the electrostatic force is changed by modulating the applied voltage ( 23 , 31 ).
Across the whole DNA sequence, pH 6 shows a number of small but significant differences from other pH conditions. These pH 6 differences seem to be associated with a physical shift of the DNA upwards within the pore. This can be seen in Figure 2A and B , by noting that in places where the pH 6 consensus is lower compared to other pHs at positions where ion current levels in Figure 2A are increasing and pH 6 ion currents are higher when the ion current levels in Figure 2A are decreasing. The effect leads to a small but noticeable deviation of up to ∼2% for ion current from pH 6 to pH 7. Differences are most significant in locations where there is a large step in current for a single motor enzyme step. We speculate this may be due to a change in length of the DNA template strand at pH 6 or a pH-dependent shift in the way Hel308 rests upon the pore-rim.
Figure 3A shows the change in mean ion current for each level in Figure 2C as a function of pH. When Z was centered in the pore constriction, we observe a sigmoidal change in current going from pH 6–9. The pH dependent probability of deprotonation can be written as:
Because the deprotonation probability describes Z ’s effective charge state, and charge in the nanopore constriction strongly affects the ion current, we can scale this expression assuming a constant, |$a$| , with units of picoampere to yield an expression for the observed ion current change as a function of pH:
Fitting our measurements of ion current differences when Z is centered in the pore constriction (Figure 3A , third from left) to Equation 2, we obtain a measure of Z ’s p K a . Using this method, we find the p K a to be 8.05 ± 0.17. This suggests that at pH 9 a single Z -base within DNA is deprotonated 90% of the time. Because p K a is known to depend on its local environment, we also measured the p K a of Z in the DNA strand using spectrophotometry following established methods ( 27 ) (Figure 4 and Supplementary Table S2 ). In the absorbance spectrum of Z , there is a distinct peak at higher wavelengths than the canonical DNA absorbance spectra. When Z is fully protonated, there is an absorbance peak centered at 385 nm. When Z is deprotonated, this peak shifts to be centered at 400 nm. At intermediate pH, the Z-absorbance spectra is a combination of these two absorbance peaks. We modeled the data at each pH as the sum of two gaussians which individually represent the absorbance spectra of the fully protonated or deprotonated Z bases. The two normalized Gaussian curves are centered at 385 nm and 400 nm ( |${G_{385}}$| and |${G_{400}}$| ), representing the protonated and deprotonated spectra, respectively. Each Gaussian curve is normalized, and the widths ( |${\sigma _1}$| and |${\sigma _2}$| ) are simultaneously fit across all pH conditions. The ratio of the Gaussian amplitudes is determined by the relative difference of pH and the p K a .

Spectrophotometric measurement of Z base. Using a Nanodrop 2000 spectrophotometer we experimentally determined the pKa of the Z base in the context of the template DNA strand used herein. ( A ) The template DNA strand with Z compared to the absorbance spectra of an identical template DNA strand with the Z substituted for a T. We can subtract the T-strand spectra from the template strand spectra to isolate the absorbance peak associated with the single Z base which is centered at 385 nm when protonated and at 400 nm when deprotonated. ( B ) The ratio between the 385nm and 400nm absorbance peaks is determined by the pH and the pK a . ( C – F ) Shows the absorbance spectra for the template DNA strand at pH 6, 7, 8, and 9, respectively.
We simultaneously fit the p K a and the widths ( |${\sigma _1}$| and |${\sigma _2}$| ) across all four equations while |${A_i}$| is a scaling parameter to fit the overall magnitude of the absorbance peaks, for each pH condition. The best fit values for the parameters are listed below in Supplementary Table S2 .
From these fits, we determined that the Z base has a p K a of 8.05 ± 0.12 within this sequence context (Figure 4 ). This result is in good agreement with our nanopore measurement.
This study illustrates the sensitivity of the nanopore to detect single elementary charges transiently binding to trapped molecules, allowing us to determine the location and occupation of a single protonation site within a polymer. A series of pH experiments like the one in this work could be used to map the locations and p K a s of multiple different protonation sites along a polymer using the nanopore.
These experiments also provide some insight regarding the local pH in the pore environment compared to the bulk solution. pH is inherently a bulk descriptor of a solution that can vary significantly near surfaces. However, the agreement of the spectrophotometric measurement of Z-base p K a with the result from the nanopore experiments supports the idea that the pH in the M2-MspA constriction is similar to the bulk solution for the pH range of 6–9. This conclusion is consistent with the fact that we use a mutated MspA, which replaces three aspartic acid residues within the constriction with aspargines ( 22 ) to achieve a neutral charge near the pore constriction.
The effect of additional negative charge in the nanopore has been previously shown to result in increased ion current ( 9 ). This ion current increase is generally attributed to counterion condensation which effectively increases the local cation concentration, increasing pore conductivity. Understanding the influence of molecules’ p K a and protonation state on ion current signal, will be informative to efforts to expand the set of molecules that are sequenced or analyzed using nanopores. These include RNA modifications, some of which are known to have p K a s near neutral ( 13 ) and peptides ( 32 ). RNA modifications with known p K a s could be sequenced by conducting two experiments above and below the p K a . Additionally, an understanding of how pH affects the nanopore ion current signal enables bespoke optimization of nanopore sequencing experiments, since conditions can be rationally selected to optimize the signal or the behavior of the trapped molecule, depending on the p K a s of groups on the molecules being analyzed.
The data underlying this article are available in FigShare, at https://dx.doi.org/10.6084/m9.figshare.25668666 .
Supplementary Data are available at NAR Online.
Author contributions : Experimental design: A.H.L. J.M.C., H.B. D.C.S., C.A.T., J.H.G.; Performed experiments: D.C.S., M.C.F., S.J.A., J.D.C.; Contributed reagents and analytic tools: D.C.S., J.M.C., C.A.T., A.H.L., H.B., S.H., S.A.B.; Analyzed data: D.C.S., A.H.L. Wrote paper/significant edits: D.C.S., A.H.L., J.H.G., C.A.T., H.B., S.A.B., S.H.
D.C.S., C.A.T., S.H., S.J.A., M.C.F., J.D.C., J.H.G., S.A.B. and A.H.L. were supported by an Opportunity Fund through the Technology Development Coordinating Center at Jackson Laboratories (National Human Genome Research Institute [U24HG011735]); the Z -base chemistry was developed under a grant from the National Science Foundation [MCB-1939086]; National Institutes of General Medical Sciences [1R01GM141391-01A1]; J.M.C. was supported by the National Institutes of Health, National Human Genome Research Institute [R01HG005115]. Funding for open access charge: National Institutes of Health, National Human Genome Research Institute [R01HG005115].
Conflict of interest statement . The authors declare the following competing financial interest(s): S.A.B. owns Firebird Biomolecular Sciences, which makes various hachimoji reagents available for sale. The authors declare no other competing financial interests.
Manrao E.A. , Derrington I.M. , Laszlo A.H. , Langford K.W. , Hopper M.K. , Gillgren N. , Pavlenok M. , Niederweis M. , Gundlach J.H. Reading DNA at single-nucleotide resolution with a mutant MspA nanopore and phi29 DNA polymerase . Nat. Biotechnol. 2012 ; 30 : 349 – 353 .
Google Scholar
Thomas C.A. , Craig J.M. , Hoshika S. , Brinkerhoff H. , Huang J.R. , Abell S.J. , Kim H.C. , Franzi M.C. , Carrasco J.D. , Kim H.-J. et al. . Assessing readability of an 8-letter expanded deoxyribonucleic acid alphabet with nanopores . J. Am. Chem. Soc. 2023 ; 145 : 8560 – 8568 .
Workman R.E. , Tang A.D. , Tang P.S. , Jain M. , Tyson J.R. , Razaghi R. , Zuzarte P.C. , Gilpatrick T. , Payne A. , Quick J. et al. . Nanopore native RNA sequencing of a human poly(A) transcriptome . Nat. Methods . 2019 ; 16 : 1297 – 1305 .
Laszlo A.H. , Derrington I.M. , Brinkerhoff H. , Langford K.W. , Nova I.C. , Samson J.M. , Bartlett J.J. , Pavlenok M. , Gundlach J.H. Detection and mapping of 5-methylcytosine and 5-hydroxymethylcytosine with nanopore MspA . Proc. Natl. Acad. Sci. U.S.A. 2013 ; 110 : 18904 – 18909 .
Burrows C.J. , Fleming A.M. Bisulfite and nanopore sequencing for pseudouridine in RNA . Acc. Chem. Res. 2023 ; 56 : 2740 – 2751 .
Cao C. , Krapp L.F. , Ouahabi A. , A. K. , N.F. C. , N. R. , A. L. , Peraro M.D Aerolysin nanopores decode digital information stored in tailored macromolecular analytes . Sci. Adv. 2020 ; 6 : eabc2661 .
Jia W. , Hu C. , Wang Y. , Liu Y. , Wang L. , Zhang S. , Zhu Q. , Gu Y. , Zhang P. , Ma J. et al. . Identification of single-molecule catecholamine enantiomers using a programmable nanopore . ACS Nano . 2022 ; 16 : 6615 – 6624 .
Wang Y. , Montana V. , Grubišić V. , Stout R.F. , Parpura V. , Gu L.-Q. Nanopore sensing of botulinum toxin type B by discriminating an enzymatically cleaved peptide from a synaptic protein synaptobrevin 2 derivative . ACS Appl. Mater. Interfaces . 2015 ; 7 : 184 – 192 .
Nova I.C. , Ritmejeris J. , Brinkerhoff H. , Koenig T.J.R. , Gundlach J.H. , Dekker C. Detection of phosphorylation post-translational modifications along single peptides with nanopores . Nat. Biotechnol. 2023 ; 42 : 710 – 714 .
Ensslen T. , Sarthak K. , Aksimentiev A. , Behrends J.C. Resolving isomeric posttranslational modifications using a biological nanopore as a sensor of molecular shape . J. Am. Chem. Soc. 2022 ; 144 : 16060 – 16068 .
Kasianowicz J.J. , Bezrukov S.M. Protonation dynamics of the alpha-toxin ion channel from spectral analysis of pH-dependent current fluctuations . Biophys. J. 1995 ; 69 : 94 – 105 .
Franceschini L. , Mikhailova E. , Bayley H. , Maglia G. Nucleobase recognition at alkaline pH and apparent pKa of single DNA bases immobilised within a biological nanopore . Chem. Commun. Camb. Engl. 2012 ; 48 : 1520 – 1522 .
Jones E.L. , Mlotkowski A.J. , Hebert S.P. , Schlegel H.B. , Chow C.S. Calculations of pKa values for a series of naturally occurring modified nucleobases . J. Phys. Chem. A . 2022 ; 126 : 1518 – 1529 .
Hoshika S. , Leal N.A. , Kim M.-J. , Kim M.-S. , Karalkar N.B. , Kim H.-J. , Bates A.M. , Watkins N.E. , SantaLucia H.A. , Meyer A.J. et al. . Hachimoji DNA and RNA: a genetic system with eight building blocks . Science . 2019 ; 363 : 884 – 887 .
Pogostin B.H. , Malmendal A. , Londergan C.H. , Åkerfeldt K.S. pKa determination of a histidine residue in a short peptide using raman spectroscopy . Molecules . 2019 ; 24 : 405 .
Kawabe H. , Thomas C.A. , Hoshika S. , Kim M.-J. , Kim M.-S. , Miessner L. , Kaplan N. , Craig J.M. , Gundlach J.H. , Laszlo A.H. et al. . Enzymatic synthesis and nanopore sequencing of 12-letter supernumerary DNA . Nat. Commun. 2023 ; 14 : 6820 .
Ledbetter M.P. , Craig J.M. , Karadeema R.J. , Noakes M.T. , Kim H.C. , Abell S.J. , Huang J.R. , Anderson B.A. , Krishnamurthy R. , Gundlach J.H. et al. . Nanopore sequencing of an expanded genetic alphabet reveals high-fidelity replication of a predominantly hydrophobic unnatural base pair . J. Am. Chem. Soc. 2020 ; 142 : 2110 – 2114 .
Nova I.C. , Derrington I.M. , Craig J.M. , Noakes M.T. , Tickman B.I. , Doering K. , Higinbotham H. , Laszlo A.H. , Gundlach J.H. Investigating asymmetric salt profiles for nanopore DNA sequencing with biological porin MspA . PLoS One . 2017 ; 12 : e0181599 .
Jerome C.A. , Hoshika S. , Bradley K.M. , Benner S.A. , Biondi E. In vitro evolution of ribonucleases from expanded genetic alphabets . Proc. Natl. Acad. Sci. U.S.A. 2022 ; 119 : e2208261119 .
Hutter D. , Benner S.A. Expanding the genetic alphabet: non-epimerizing nucleoside with the pyDDA hydrogen-bonding pattern . J. Org. Chem. 2003 ; 68 : 9839 – 9842 .
Heinz C. , Engelhardt H. , Niederweis M. The core of the tetrameric mycobacterial porin MspA is an extremely stable β-sheet domain* . J. Biol. Chem. 2003 ; 278 : 8678 – 8685 .
Butler T.Z. , Pavlenok M. , Derrington I.M. , Niederweis M. , Gundlach J.H. Single-molecule DNA detection with an engineered MspA protein nanopore . Proc. Natl. Acad. Sci. U.S.A. 2008 ; 105 : 20647 – 20652 .
Noakes M.T. , Brinkerhoff H. , Laszlo A.H. , Derrington I.M. , Langford K.W. , Mount J.W. , Bowman J.L. , Baker K.S. , Doering K.M. , Tickman B.I. et al. . Increasing the accuracy of nanopore DNA sequencing using a time-varying cross membrane voltage . Nat. Biotechnol. 2019 ; 37 : 651 – 656 .
Laszlo A.H. , Derrington I.M. , Gundlach J.H. MspA nanopore as a single-molecule tool: from sequencing to SPRNT . Methods . 2016 ; 105 : 75 – 89 .
Craig J.M. , Laszlo A.H. , Nova I.C. , Brinkerhoff H. , Noakes M.T. , Baker K.S. , Bowman J.L. , Higinbotham H.R. , Mount J.W. , Gundlach J.H. Determining the effects of DNA sequence on Hel308 helicase translocation along single-stranded DNA using nanopore tweezers . Nucleic Acids Res. 2019 ; 47 : 2506 – 2513 .
Craig J.M. , Laszlo A.H. , Brinkerhoff H. , Derrington I.M. , Noakes M.T. , Nova I.C. , Tickman B.I. , Doering K. , de Leeuw N.F. , Gundlach J.H. Revealing dynamics of helicase translocation on single-stranded DNA using high-resolution nanopore tweezers . Proc. Natl. Acad. Sci. U.S.A. 2017 ; 114 : 11932 – 11937 .
Thaplyal P. , Bevilacqua P.C. Experimental approaches for measuring pKa's in RNA and DNA . Methods Enzymol. 2014 ; 549 : 189 – 219 .
LaMont C.H. , Wiggins P.A. The development of an information criterion for change-point analysis . Neural Comput. 2016 ; 28 : 594 – 612 .
Wescoe Z.L. , Schreiber J. , Akeson M. Nanopores discriminate among five C5-cytosine variants in DNA . J. Am. Chem. Soc. 2014 ; 136 : 16582 – 16587 .
Schreiber J. , Wescoe Z.L. , Abu-Shumays R. , Vivian J.T. , Baatar B. , Karplus K. , Akeson M. Error rates for nanopore discrimination among cytosine, methylcytosine, and hydroxymethylcytosine along individual DNA strands . Proc. Natl. Acad. Sci. U.S.A. 2013 ; 110 : 18910 – 18915 .
Derrington I.M. , Craig J.M. , Stava E. , Laszlo A.H. , Ross B.C. , Brinkerhoff H. , Nova I.C. , Doering K. , Tickman B.I. , Ronaghi M. et al. . Subangstrom single-molecule measurements of motor proteins using a nanopore . Nat. Biotechnol. 2015 ; 33 : 1073 – 1075 .
Larsen P.O. Miflin B.J. 6 - Physical and chemical properties of amino acids . Amino Acids and Derivatives . 1980 ; Academic Press 225 – 269 .
Google Preview
Email alerts
Citing articles via.
- Editorial Board
Affiliations
- Online ISSN 1362-4962
- Print ISSN 0305-1048
- Copyright © 2024 Oxford University Press
- About Oxford Academic
- Publish journals with us
- University press partners
- What we publish
- New features
- Open access
- Institutional account management
- Rights and permissions
- Get help with access
- Accessibility
- Advertising
- Media enquiries
- Oxford University Press
- Oxford Languages
- University of Oxford
Oxford University Press is a department of the University of Oxford. It furthers the University's objective of excellence in research, scholarship, and education by publishing worldwide
- Copyright © 2024 Oxford University Press
- Cookie settings
- Cookie policy
- Privacy policy
- Legal notice
This Feature Is Available To Subscribers Only
Sign In or Create an Account
This PDF is available to Subscribers Only
For full access to this pdf, sign in to an existing account, or purchase an annual subscription.
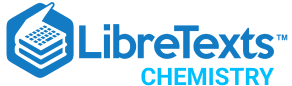
- school Campus Bookshelves
- menu_book Bookshelves
- perm_media Learning Objects
- login Login
- how_to_reg Request Instructor Account
- hub Instructor Commons
Margin Size
- Download Page (PDF)
- Download Full Book (PDF)
- Periodic Table
- Physics Constants
- Scientific Calculator
- Reference & Cite
- Tools expand_more
- Readability
selected template will load here
This action is not available.
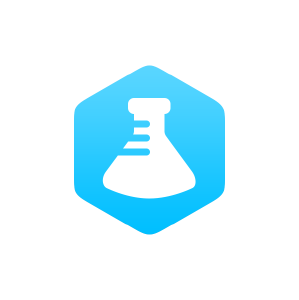
Lab 6: Acid/base titration
- Last updated
- Save as PDF
- Page ID 402771
\( \newcommand{\vecs}[1]{\overset { \scriptstyle \rightharpoonup} {\mathbf{#1}} } \)
\( \newcommand{\vecd}[1]{\overset{-\!-\!\rightharpoonup}{\vphantom{a}\smash {#1}}} \)
\( \newcommand{\id}{\mathrm{id}}\) \( \newcommand{\Span}{\mathrm{span}}\)
( \newcommand{\kernel}{\mathrm{null}\,}\) \( \newcommand{\range}{\mathrm{range}\,}\)
\( \newcommand{\RealPart}{\mathrm{Re}}\) \( \newcommand{\ImaginaryPart}{\mathrm{Im}}\)
\( \newcommand{\Argument}{\mathrm{Arg}}\) \( \newcommand{\norm}[1]{\| #1 \|}\)
\( \newcommand{\inner}[2]{\langle #1, #2 \rangle}\)
\( \newcommand{\Span}{\mathrm{span}}\)
\( \newcommand{\id}{\mathrm{id}}\)
\( \newcommand{\kernel}{\mathrm{null}\,}\)
\( \newcommand{\range}{\mathrm{range}\,}\)
\( \newcommand{\RealPart}{\mathrm{Re}}\)
\( \newcommand{\ImaginaryPart}{\mathrm{Im}}\)
\( \newcommand{\Argument}{\mathrm{Arg}}\)
\( \newcommand{\norm}[1]{\| #1 \|}\)
\( \newcommand{\Span}{\mathrm{span}}\) \( \newcommand{\AA}{\unicode[.8,0]{x212B}}\)
\( \newcommand{\vectorA}[1]{\vec{#1}} % arrow\)
\( \newcommand{\vectorAt}[1]{\vec{\text{#1}}} % arrow\)
\( \newcommand{\vectorB}[1]{\overset { \scriptstyle \rightharpoonup} {\mathbf{#1}} } \)
\( \newcommand{\vectorC}[1]{\textbf{#1}} \)
\( \newcommand{\vectorD}[1]{\overrightarrow{#1}} \)
\( \newcommand{\vectorDt}[1]{\overrightarrow{\text{#1}}} \)
\( \newcommand{\vectE}[1]{\overset{-\!-\!\rightharpoonup}{\vphantom{a}\smash{\mathbf {#1}}}} \)
Experiment 6: My First Acid - Base Titration
Learning Outcomes
Upon completion of this lab, the student will be able to:
Start preparing for emergencies in the chemical lab
Set-up a titration stand
Prepare primary standard solutions of Potassium Hydrogen Phthalate (KHP) solutions
Standardize a solution of Sodium Hydroxide (NaOH)
Perform a fast titration and a slow titration
Reagents and Supplies
Approximate 1.0 M aqueous sodium hydroxide (NaOH), potassium hydrogen phthalate (KHP), and a phenolphthalein solution.
Burette, materials from your drawer
(See posted SDS, Safety Data Sheets)
Introduction
Titration is an analytical quantitative technique used to determine the concentration of a solute; a pH-titration is used to determine the concentration of an acid or a base. Titrations play an important role in determining amount and purity in many manufacturing processes. These include food processing, textile, wood product manufacturing, petroleum, pharmaceuticals and chemical manufacturing. Titrations play a critical role in the manufacturing of biodiesel, water purification and waste water treatment and even in the production of various products in the dairy industry.
Here are some examples from pharmaceutical manufacturing. The active ingredient in many cough syrups, ephedrine, is tested for purity through titration with perchloric acid. Some drugs, such as the antifungal, clotrimazole, are end products of titration reactions. Titrations are even used to determine the amount of non-pharmacologically active ingredients, such as the binding substances that make up medicine tablets.
A titration involves two solutions: the titrant and the analyte. Typically, the titrant is slowly added from a burette to the flask containing the analyte until you reach the endpoint. At the endpoint, you know the initial volume of analyte (how much you added to our flask) and the volume of added titrant (by reading off the burette). The titrant is a solution of known concentration and you figure out the concentration of the analyte.
Consider the reaction between aqueous solutions of sulfuric acid and potassium hydroxide. The balanced chemical equation for the reaction is shown below:
\[\ce{H2SO4(aq) + 2 KOH(aq) -> K2SO4(aq) + 2 H2O(l)}\tag{1}\]
Assume that the molar concentration of \(\ce{KOH}\) is 0.100 M and that of \(\ce{H2SO4}\) is unknown.
For this example, \(\ce{KOH}\) was slowly titrated to 10.00 mL of \(\ce{H2SO4}\). The volume of 13.75 mL of \(\ce{KOH}\) was needed to completely react with the \(\ce{H2SO4}\). The concentration of the sulfuric acid (in the flask, at the beginning of the titration) is calculated as follows:
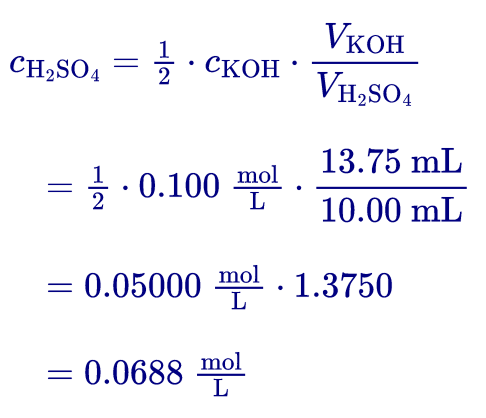
For an interactive version with more explanation, follow this link or the QR code above.
Two takeaways from this calculation:
As seen from the above calculation, the stoichiometric ratio between the two reactants is the key to the determination of the concentration of the unknown solution. ( You need a balanced equation).
Determining the unknown concentration requires meticulous determination of the volume of the titrant.
In order to conduct the above experiment, typically the \(\ce{H2SO4}\) is in an Erlenmeyer flask, and the \(\ce{KOH}\) is in a burette. The \(\ce{KOH}\) is added one drop at a time from the burette into the acid solution with constant stirring to ensure that the reagents combine and react.
The equivalence point of an acid/base reaction
The equivalence point is defined as that point in the titration when stoichiometrically equal amounts of acid and base are present. In the \(\ce{H2SO4}\)/\(\ce{KOH}\) example shown previously (eq 1), that would be when two moles of \(\ce{KOH}\) have been added to one mole of \(\ce{H2SO4}\). Therefore, the equivalence point depends on the reaction stoichiometry .
At the beginning of the titration, the solution in the Erlenmeyer flask is acidic. As the base is added, it completely reacts with the acid and the solution in the Erlenmeyer flask continues to be acidic. But, at the equivalence point, the acid has completely reacted with the base. If even one tiny drop of base is added beyond that needed to arrive at the equivalence point, the solution in the Erlenmeyer flask is basic. This difference in the acid/base property of the solution in the Erlenmeyer flask is used to visually determine the end of the titration.
Using color to visualize the equivalence point
An indicator is a chemical substance whose color depends on the acid/base property of the medium it is present in. Phenolphthalein is an indicator, which is colorless in an acidic medium and has a pink color in a basic medium.
In this titration, a few drops of phenolphthalein should be added to the acid in the Erlenmeyer flask. The solution will remain colorless until the equivalence point.
When the equivalence point has been crossed and the solution becomes basic, the phenolphthalein will take on a pink color. This is the reason to add the base drop by drop, so that even though the equivalence point will be crossed, the titration can be stopped at the appearance of the first permanent pale pink color.
This point in the titration when the indicator changes color is referred to as the End Point . Note, the End Point of a titration is slightly beyond the Equivalence Point. In the case of the phenolphthalein, the intensity of the pink color increases as the solution becomes more and more basic. Therefore, it is important to stop the titration at the appearance of a permanent pale pink color . In order to easily observe the color changes in the solution, it is a good idea to place a sheet of plain white paper beneath the flask.
My first titration
Today we will be learning the basics of acid-base titrations. We will be going over the following techniques:
Assemble a burette stand
Properly condition the burette for use
Accurately measure the volume of the burette
Dispense titrant using a ‘stop-cock’
Determine the end point of the titration
Use and dispose of waste
Analyze titration data
Today, we will determine the exact concentration of a solution of sodium hydroxide. In order to accomplish this, we will titrate the sodium hydroxide (NaOH) solution with standardized solution of KHP of known concentration.
Sodium hydroxide is an extremely hygroscopic solid. When solid sodium hydroxide is weighed using an electronic balance it tends to absorb moisture from the atmosphere; this leads to inaccuracies in the mass of the sodium hydroxide and thereby inaccuracies in its molarity calculations.
In order to circumvent the above problem, a sodium hydroxide solution of approximate concentration is prepared first. This solution is titrated with a primary acid standard . The concentration of this acid is known with greater accuracy. The data from this titration is then used to calculate a more accurate value for the concentration of the sodium hydroxide solution. A commonly used primary standard for titration with sodium hydroxide solution is the weak acid potassium hydrogen phthalate or KHP (\(\ce{C8H5O4K}\)).
The reaction between KHP and NaOH is given below:
\[\ce{HC8H4O4K + NaOH -> C8H4O4KNa + H2O}\]
____ mole(s) of NaOH must be added for every _____ mole(s) of \(\ce{C8H5O4K}\) in solution
The titration of NaOH with KHP involves adding NaOH from the burette to a known mass of KHP in solution. The data from the titration is then used to calculate the molarity of the NaOH.
Titrations as a technique
Today you will be performing two types of titrations:
In a fast titration, the titrant (solution in the burette) is released quickly into the solution in the flask below, hence fast. The goal of this titration is to determine the approximate volume of titrant needed to induce the change of color (determine the end point). This titration is not quantitative; it will not give an accurate determination of the unknown concentration.
In contrast, a slow titration will give you an accurate determination of the unknown concentration; however, the titrant is released very slowly into the solution in the flask below . This is especially true as you near the end point. Remember, it is important to stop the titration at the appearance of a permanent pale pink color. This slow, methodical process will yield an accurate measurement of volume of titrant.
Record all your calculations, measurements, and observations into your notebook, and then fill in your Worksheet .
Task 1: Safety Video on Preparing for Emergencies
Please watch video #6 in the ACS lab safety series.
As you are watching, think of which emergencies could have happened in previous sessions already (with the materials and substances we have been using), and how we prepared for them. When you are done, answer the questions on the handout. Today's lab involves acids and bases. Listen carefully as your instructor explains the hazards associated with them.
Task 2: Standardization of the Sodium Hydroxide (NaOH) Solution
Preparing khp solution (in flask).
Calculate the mass of KHP needed to prepare 25.00 mL of 0.100 M KHP and write this calculation in your notebook. Check your calculation with your instructor before moving to Step 2.
Weigh an amount of KHP as close as possible to the calculated mass in step 1 and record the exact mass of KHP measured.
Transfer the solid KHP into a 125-mL Erlenmeyer flask.
Add 25 mL of deionized water into the 125-mL Erlenmeyer flask containing the solid KHP.
Add one drop of phenolphthalein solution into the Erlenmeyer flask and swirl the flask to mix.
Preparing NaOH Solution (in Burette)
- Calculate the volume of approximately 1.0 M aqueous sodium hydroxide solution needed to prepare 250-mL of an approximately 0.10 M aqueous sodium hydroxide solution. Write your calculations in your lab notebook. Check your calculations with the lab instructor before proceeding to the next step.
Measure the volume of 1.0M aqueous sodium hydroxide solution calculated in step 3 using the 25-mL graduated cylinder located in the fume hood. Pour this volume into your Florence flask and dilute the solution with enough deionized water to make a total volume of 250-mL. Remember this 0.1M is only an approximate concentration.
Label the flask with your name, date, lab section, and 0.1M aqueous sodium hydroxide (do not use chemical symbols) with a Sharpie.
Clamp a burette to the burette stand.
Condition the burette by filling with water. Allow the water to drain. Water can be discarded in sink. Rinse the burette with a few mL of NaOH solution and then discard this into the waste container. Then fill the burette slightly above the "zero" mark with NaOH solution.
Drain some of the NaOH solution so that there are no air bubbles in the tip of the burette AND so that the level of NaOH falls between 0.00mL and 1.00mL. Record the initial burette reading, which will NOT be at 0.00mL.
Fast titration (perform once)
- Titrate NaOH into the KHP solution until a faint but permanent pale pink color is obtained. Make sure to swirl the Erlenmeyer flask thoroughly to ensure mixing of the reagents. In case any NaOH solution falls on the side of the Erlenmeyer flask, rinse the sides of the flask with deionized water. Record the volume of NaOH used to reach the endpoint.
Slow titration (perform three times)
Set up another fresh KHP solution in a clean Erlenmeyer flask with indicator (see steps 1-5).
Titrate with NaOH slowly. If the level of NaOH is close to 50 mL (i.e. bottom of the scale), write down the value, fill the burette with more diluted NaOH, and write down the new value. This allows you to calculate the total amount dispensed from the burette. Don't let the level of the NaOH go below the 50.00mL line on the burette or you won't be able to determine the volume dispensed and will need to start the titration over.
Remember to slow down to a drip at least 1-2 mL before the recorded volume you observed in the fast titration . Make sure to swirl the Erlenmeyer flask thoroughly to ensure mixing of the reagents. Stop when a sustained faint pink color is observed.
Repeat steps 13 – 15 to conduct three total slow titrations.
Task 3: Calculate the concentration of NaOH
17. Using the average data from the slow titrations, calculate the concentration of NaOH in units of mol/L.
18. Cover the remaining NaOH solution with parafilm and store your labeled Florence flask on the side counter.
19. Check with your instructor before cleaning up. Dispose all waste into appropriate waste disposal containers as instructed by your instructor. Clean and return all glassware. Place the burette upside down on the stand and turn the stop-cock to the open position to allow it to drain and dry.
Experiment 1 (Titration of Amino Acids) Updated Protocol (24M)

COMMENTS
Experiment 1 Acid-Base Titrations Discussion Volumetric procedures are among the most common and convenient methods of analysis. The preparation of a reactive solution of accurately known concentration is fundamental to these methods, and the exercise serves as an introduction to the techniques of solution preparation and titration.
pH Titrations. In a pH titration you measure the pH as a function of the volume of titrant added and determine the equivalence point as the point in where there is an inflection in the slope of the curve. Figure 7.2.2 shows the four common types of titrations. Initially the pH is that of the pure analyte.
A titration is an experiment where a volume of a solution of known concentration is added to a volume of another solution in order to determine its concentration. Many titrations are acid-base neutralization reactions, though other types of titrations can also be performed. In order to perform an acid-base titration, the chemist must have a way ...
Acid-Base Titrations. Acid-Base titrations are usually used to find the amount of a known acidic or basic substance through acid base reactions. The analyte (titrand) is the solution with an unknown molarity. The reagent (titrant) is the solution with a known molarity that will react with the analyte.
At the end-point, read the final volume of base in the buret and record the volume of base used (SUBTRACT) in the Data section. You will also want to record the molarity of the base which is 0.20 Molar for all titrations in this experiment. 8. After the first titration, wash out the flask again, rinse down the sides with distilled water, and
5.2 ACIDS, BASES AND SALTS (j) titration as a method to prepare solutions of soluble salts and to determine relative and actual concentrations of solutions of acids/alkalis; A/AS level. WJEC Chemistry. Unit 1: THE LANGUAGE OF CHEMISTRY, STRUCTURE OF MATTER AND SIMPLE REACTIONS. 1.7 Simple equilibria and acid-base reactions (f) acid-base titrations
Calculating pH for Titration Solutions: Strong Acid/Strong Base A titration is carried out for 25.00 mL of 0.100 M HCl (strong acid) with 0.100 M of a strong base NaOH (the titration curve is shown in Figure 14.18). Calculate the pH at these volumes of added base solution: (a) 0.00 mL (b) 12.50 mL (c) 25.00 mL (d) 37.50 mL. Solution
8 ∙ Acid-base titration 8‐2 Experiment 8 Acid-base titration Mathematical development Acids and bases are indispensable fixtures of daily life. Acids can be recognized by their sharp taste. The tartness of lemon juice is caused by citric acid, the sourness of wine by tartaric acid and malonic acid. Bases, on
Procedure. Clamp the microscale titration apparatus securely in position as in photograph and push the syringe plunger completely down. Fill the apparatus with 0.10 M hydrochloric acid as follows. Put about 5 cm 3 of the acid in a 10 cm 3 beaker and place the tip of the apparatus well down into the solution. Raise the syringe plunger slowly and ...
Experiment 8 - Acid/Base Titrations Discussion This experiment demonstrates an analytical technique known as titration, where a solution is delivered from a buret until it completely consumes another solution in a flask. Consider the following: Acid-base titrations are an example of volumetric analysis, a
Experiment 1: Acid-Base Titration Report Due Date : 29 October 2021 Lecturer's Name : Dr Showkat Ahmad Bhawani ... Discussion 14-Questions and answers 21-Conclusion; Attachments 24- ... In an acid-base titration analysis, there should be a sudden change in pH when the reaction is complete. For example, if the sample being titrated is an acid ...
Pipette 25 mL of standard sodium carbonate solution into a 250 mL conical flask, add 2 drops of methyl orange. Titrate with the given hydrochloric acid until the solution starts becoming red. Repeat the titration, this time immediately add the acid until it is short of the titer value obtained in (ii) by 0.5 mL.
Figure 16.5.2 The Titration of (a) a Strong Acid with a Strong Base and (b) a Strong Base with a Strong Acid (a) As 0.20 M NaOH is slowly added to 50.0 mL of 0.10 M HCl, the pH increases slowly at first, then increases very rapidly as the equivalence point is approached, and finally increases slowly once more.
4 0. Discussion. The goal of the volumetric glassware calibration experiment is to calibra te a specific. measurement using volumetric glassware. The mass of water contained in or supplied by the. glassware was measured for calibration. T o conv0ert mass into volume, the density of water. at a specific temperature is determined.
of the setup for. a titration. Acid-base titrations involve the dropwise addition of one solution-phase reagent (using a buret) into a carefully measured amount of another reagent (in an Erlenmeyer flask). There are three main classes of titrations that can be studied: Strong acid-strong base. Weak acid-strong base. Weak base-strong acid.
Acid and Base Titration Experiment Discussion: Part 1: Acid and Base titrations are used to determine the concentration of a solution with unknown concentration. This method is made possible by stoichiometry and background knowledge about the reactions between acids and bases. When an acid reacts with a base, they neutralize the individual acid and base properties and produce salt and water.
Equivalence point: point in titration at which the amount of titrant added is just enough to completely neutralize the analyte solution. At the equivalence point in an acid-base titration, moles of base = moles of acid and the solution only contains salt and water. Acid-base titrations are monitored by the change of pH as titration progresses.
Principle of acid-base titration. In the theory of acid-base titration, the principle involves using a burette and pipette to determine the concentration of an acid or basic. An indicator is a dye added to a solution to change its color. It is dissolved in the sample solution and can be used to detect the end of the titration.
13. In this experiment, the ratio of base to acid is 1:1, so for every mole of base used, one mole of acid is used. ... Discussion Questions. ... Acid-Base Titration Lab Related Study Materials.
Titration of a strong acid with a strong base. In a strong acid-strong base titration, the acid and base will react to form a neutral solution. At the equivalence point of the reaction, hydronium (H+) and hydroxide (OH-) ions will react to form water, leading to a pH of 7. This is true of all strong acid-strong base titrations.
There are four parts to the titration curve of a weak acid (analyte) with a strong base (titrant). Buffer Equation (Henderson Hasselbach Eq.) Figure17.3.3: Four parts of the titration curve for a weak acid being titrated with a strong base. Notice that two parts are points (1 & 3) and two parts are regions (2 & 4).
Experiment 12 - Acid/Base Titrations Discussion This experiment demonstrates an analytical technique known as titration, where a solution is delivered from a buret until it completely consumes another solution in a flask. Consider the following: Acid-base titrations are an example of volumetric analysis, a
Experiment 3 - Analysis of Antacids by Acid-Base Titration Procedure: 1. 2 diff brand antacid tablets and record from lab presentation a. Brand name b. Number of tablets in bottle c. Cost per bottle d. Mass of active ingredient in each tablet 2. WEIGH and RECORD mass of tablet - then grind one tablet with mortar and pestle to find powder a. WEIGH approx 0.2g of powder into each of 2 125mL ...
The most notable effect is an increase in ion current when deprotonated Z is centered in the pore constriction. When Z is deprotonated, it is negatively charged (Figure 1A).An ion current increase due to more negatively charged DNA is consistent with previously published observations of heterogeneously charged polymers within nanopores (9, 18), and may be caused by the attraction of current ...
Titration is an analytical quantitative technique used to determine the concentration of a solute; a pH-titration is used to determine the concentration of an acid or a base. Titrations play an important role in determining amount and purity in many manufacturing processes. These include food processing, textile, wood product manufacturing ...
In this experiment, you will titrate the amino acid glycine and an unknown amino acid. Using the collected data, you will then plot titration curves for each of the tested amino acids. The titration curve shows how the pH changes as a result of the addition of a volume of base. An example of a typical titration curve is shown in Figure 1.