Featured Topics
Featured series.
A series of random questions answered by Harvard experts.

Explore the Gazette
Read the latest.
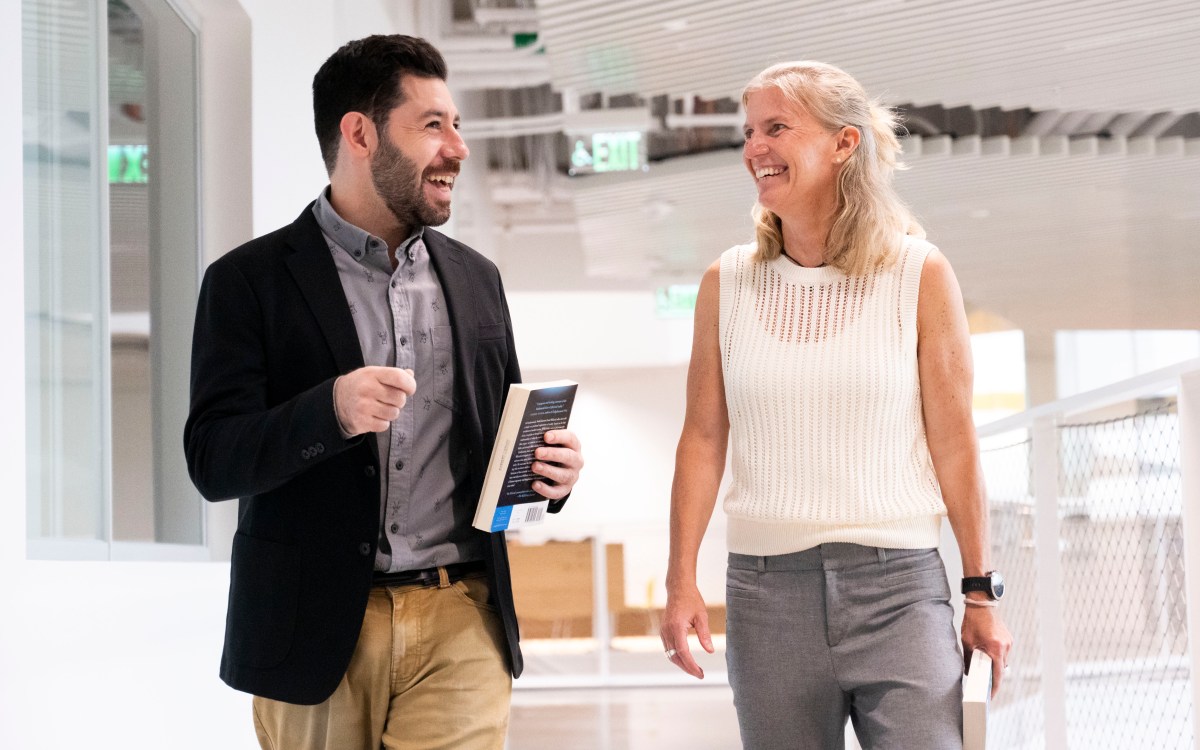
Professor tailored AI tutor to physics course. Engagement doubled.
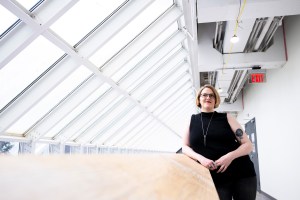
Did lawmakers know role of fossil fuels in climate change during Clean Air Act era?
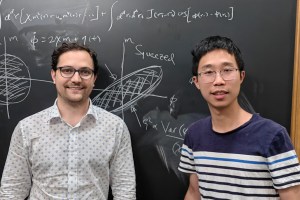
Spin squeezing for all
The down side to wind power.
Leah Burrows
SEAS Communications
Wind farms will cause more environmental impact than previously thought
When it comes to energy production, there’s no such thing as a free lunch, unfortunately.
As the world begins its large-scale transition toward low-carbon energy sources, it is vital that the pros and cons of each type are well understood and the environmental impacts of renewable energy, small as they may be in comparison to coal and gas, are considered.
In two papers — published today in the journals Environmental Research Letters and Joule — Harvard University researchers find that the transition to wind or solar power in the U.S. would require five to 20 times more land than previously thought, and, if such large-scale wind farms were built, would warm average surface temperatures over the continental U.S. by 0.24 degrees Celsius.
“Wind beats coal by any environmental measure, but that doesn’t mean that its impacts are negligible,” said David Keith, the Gordon McKay Professor of Applied Physics at the Harvard John A. Paulson School of Engineering and Applied Sciences (SEAS) and senior author of the papers. “We must quickly transition away from fossil fuels to stop carbon emissions. In doing so, we must make choices between various low-carbon technologies, all of which have some social and environmental impacts.”
Keith is also professor of public policy at the Harvard Kennedy School.
One of the first steps to understanding the environmental impact of renewable technologies is to understand how much land would be required to meet future U.S. energy demands. Even starting with today’s energy demands, the land area and associated power densities required have long been debated by energy experts.
In previous research, Keith and co-authors modeled the generating capacity of large-scale wind farms and concluded that real-world wind power generation had been overestimated because they neglected to accurately account for the interactions between turbines and the atmosphere.
“The direct climate impacts of wind power are instant, while the benefits of reduced emissions accumulate slowly.” David Keith
In 2013 research, Keith described how each wind turbine creates a “wind shadow” behind it where air has been slowed down by the turbine’s blades. Today’s commercial-scale wind farms carefully space turbines to reduce the impact of these wind shadows, but given the expectation that wind farms will continue to expand as demand for wind-derived electricity increases, interactions and associated climatic impacts cannot be avoided.
What was missing from this previous research, however, were observations to support the modeling. Then, a few months ago, the U.S. Geological Survey released the locations of 57,636 wind turbines around the U.S. Using this data set, in combination with several other U.S. government databases, Keith and postdoctoral fellow Lee Miller were able to quantify the power density of 411 wind farms and 1,150 solar photovoltaic plants operating in the U.S. during 2016.
“For wind, we found that the average power density — meaning the rate of energy generation divided by the encompassing area of the wind plant — was up to 100 times lower than estimates by some leading energy experts,” said Miller, who is the first author of both papers. “Most of these estimates failed to consider the turbine-atmosphere interaction. For an isolated wind turbine, interactions are not important at all, but once the wind farms are more than five to 10 kilometers deep, these interactions have a major impact on the power density.”
The observation-based wind power densities are also much lower than important estimates from the U.S. Department of Energy and the Intergovernmental Panel on Climate Change.
For solar energy, the average power density (measured in watts per meter squared) is 10 times higher than wind power, but also much lower than estimates by leading energy experts.
This research suggests that not only will wind farms require more land to hit the proposed renewable energy targets but also, at such a large scale, would become an active player in the climate system.
The next question, as explored in the journal Joule, was how such large-scale wind farms would impact the climate system.
“If your perspective is the next 10 years, wind power actually has — in some respects — more climate impact than coal or gas. If your perspective is the next thousand years, then wind power has enormously less climatic impact than coal or gas.” David Keith
To estimate the impacts of wind power, Keith and Miller established a baseline for the 2012‒2014 U.S. climate using a standard weather-forecasting model. Then, they covered one-third of the continental U.S. with enough wind turbines to meet present-day U.S. electricity demand. The researchers found this scenario would warm the surface temperature of the continental U.S. by 0.24 degrees Celsius, with the largest changes occurring at night when surface temperatures increased by up to 1.5 degrees. This warming is the result of wind turbines actively mixing the atmosphere near the ground and aloft while simultaneously extracting from the atmosphere’s motion.
This research supports more than 10 other studies that observed warming near operational U.S. wind farms. Miller and Keith compared their simulations to satellite-based observational studies in North Texas and found roughly consistent temperature increases.
Miller and Keith are quick to point out the unlikeliness of the U.S. generating as much wind power as they simulate in their scenario, but localized warming occurs in even smaller projections. The follow-on question is then to understand when the growing benefits of reducing emissions are roughly equal to the near-instantaneous impacts of wind power.
The Harvard researchers found that the warming effect of wind turbines in the continental U.S. was actually larger than the effect of reduced emissions for the first century of its operation. This is because the warming effect is predominantly local to the wind farm, while greenhouse gas concentrations must be reduced globally before the benefits are realized.
Miller and Keith repeated the calculation for solar power and found that its climate impacts were about 10 times smaller than wind’s.
“The direct climate impacts of wind power are instant, while the benefits of reduced emissions accumulate slowly,” said Keith. “If your perspective is the next 10 years, wind power actually has — in some respects — more climate impact than coal or gas. If your perspective is the next thousand years, then wind power has enormously less climatic impact than coal or gas.
“The work should not be seen as a fundamental critique of wind power,” he said. “Some of wind’s climate impacts will be beneficial — several global studies show that wind power cools polar regions. Rather, the work should be seen as a first step in getting more serious about assessing these impacts for all renewables. Our hope is that our study, combined with the recent direct observations, marks a turning point where wind power’s climatic impacts begin to receive serious consideration in strategic decisions about decarbonizing the energy system.”
This research was funded by the Fund for Innovative Climate and Energy Research.
Share this article
You might like.
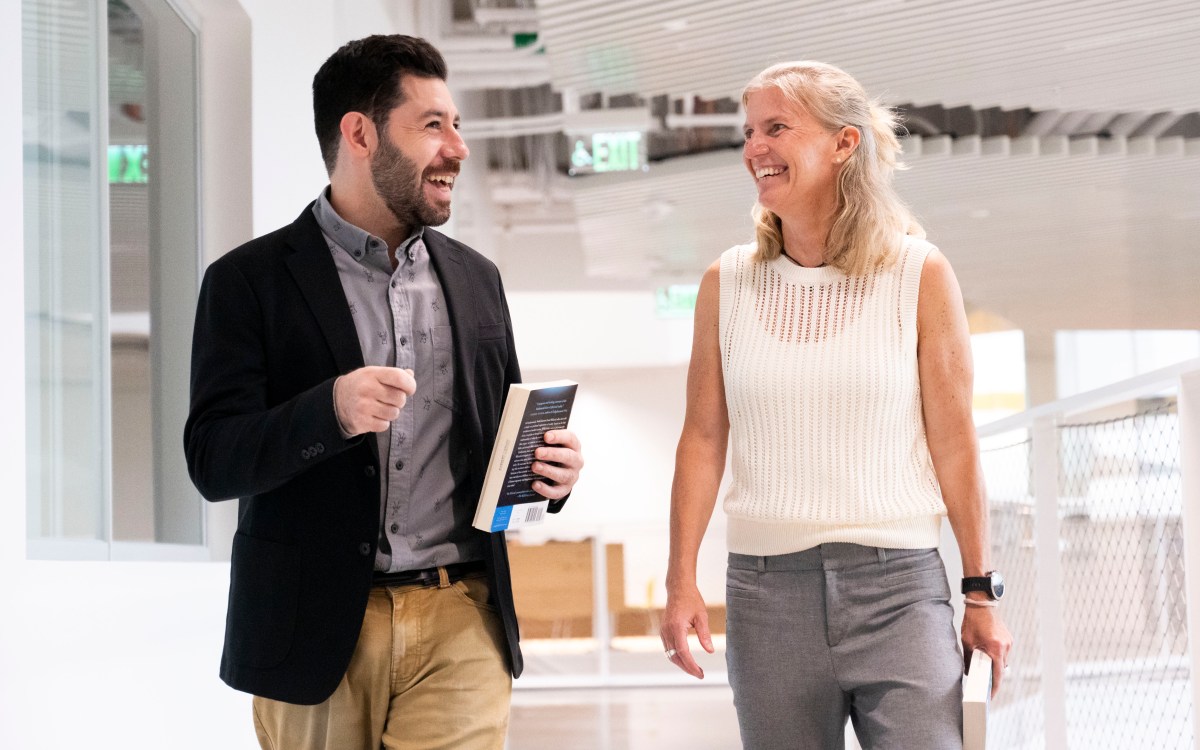
Preliminary findings inspire other large Harvard classes to test approach this fall
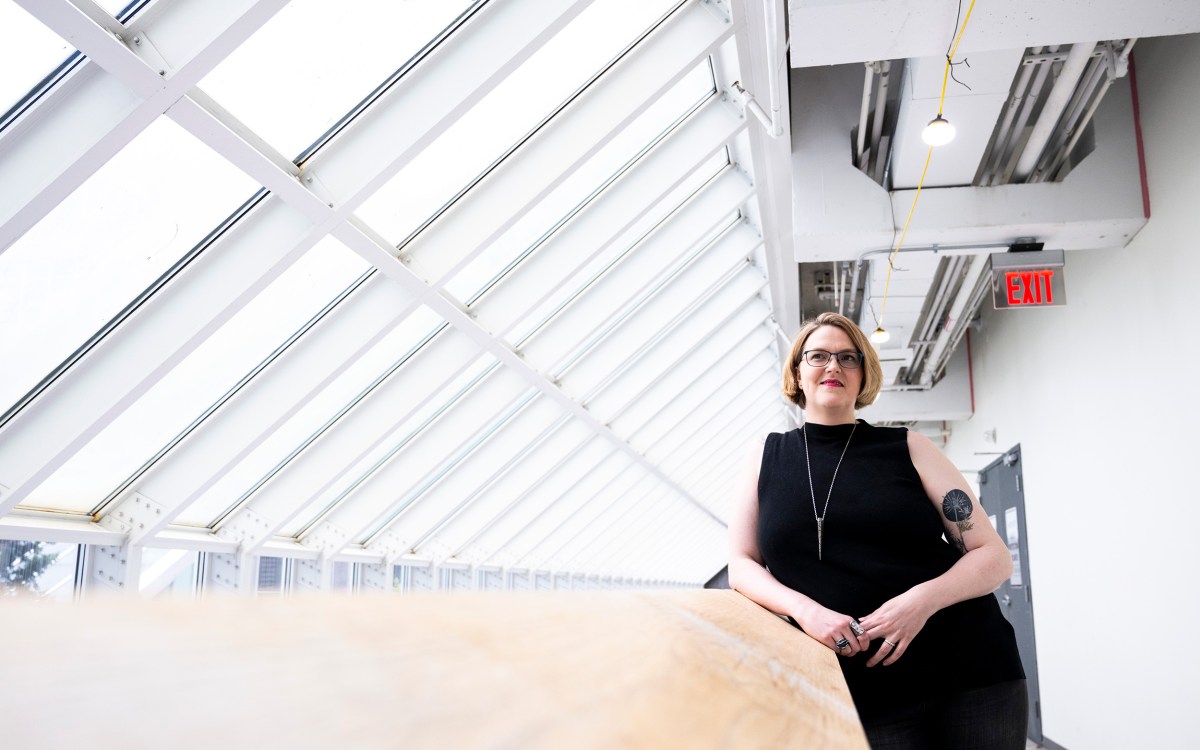
New study suggests they did, offering insight into key issue in landmark 2022 Supreme Court ruling on EPA
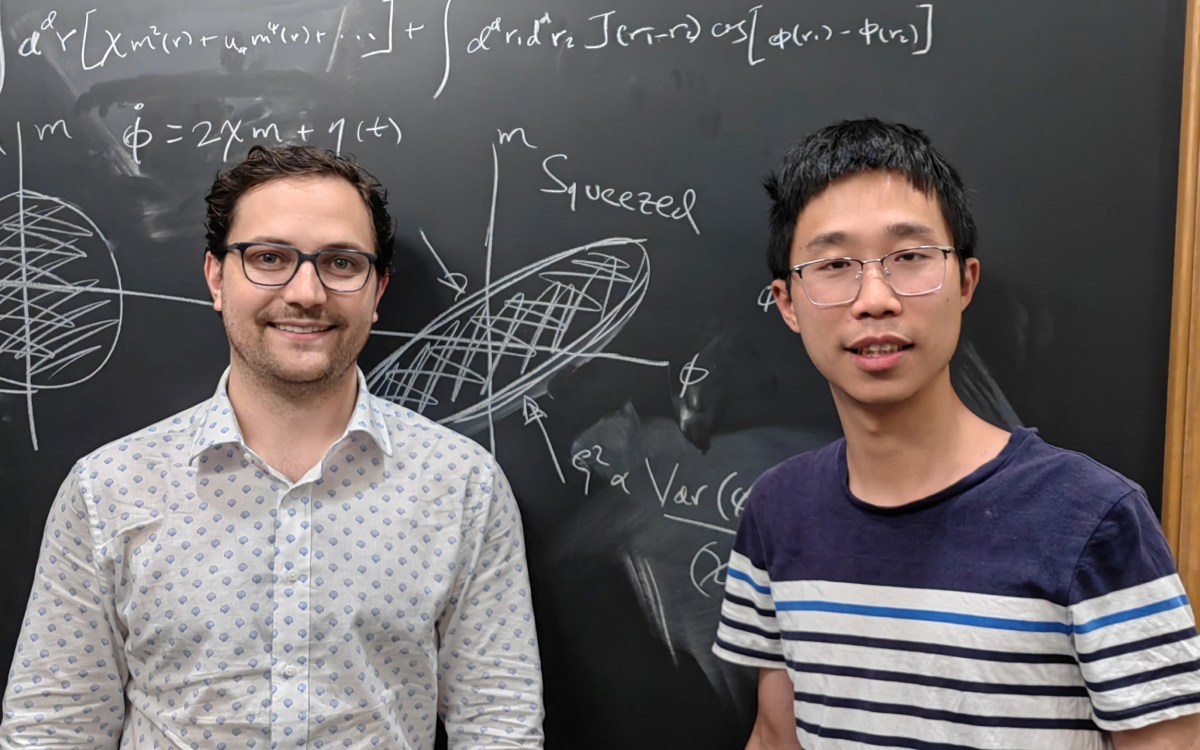
Physicists ease path to entanglement for quantum sensing
Billions worldwide deficient in essential micronutrients
Inadequate levels carry risk of adverse pregnancy outcomes, blindness
You want to be boss. You probably won’t be good at it.
Study pinpoints two measures that predict effective managers
Weight-loss drug linked to fewer COVID deaths
Large-scale study finds Wegovy reduces risk of heart attack, stroke
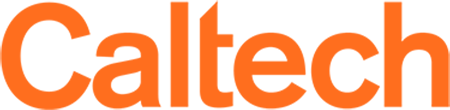
What Is the Future of Wind Energy?
Humans have used windmills to capture the force of the wind as mechanical energy for more than 1,300 years . Unlike early windmills, however, modern wind turbines use generators and other components to convert energy from the spinning blades into a smooth flow of AC electricity.
In the video below, Resnick Sustainability Institute researcher John Dabiri discusses the future of wind energy technology.
How much of global electricity demand is met by wind energy?
Wind energy is a small but fast-growing fraction of electricity production. It accounts for 5 percent of global electricity production and 8 percent of the U.S. electricity supply.
Globally, wind energy capacity surpasses 743 gigawatts , which is more than is available from grid-connected solar energy and about half as much as hydropower can provide. Nearly three-quarters of that 651 gigawatts comes from wind farms in five countries: China, the U.S., Germany, India, and Spain. Wind energy capacity in the Americas has tripled over the past decade.
In the U.S., wind is now a dominant renewable energy source , with enough wind turbines to generate more than 100 million watts, or megawatts, of electricity, equivalent to the consumption of about 29 million average homes.
The cost of wind energy has plummeted over the past decade. In the U.S., it is cost-competitive with natural gas and solar power.
Wind energy and solar energy complement each other, because wind is often strongest after the sun has heated the ground for a time. Warm air rises from the most heated areas, leaving a void where other air can rush in, which produces horizontal wind currents . We can draw on solar energy during the earlier parts of the day and turn to wind energy in the evening and night. Wind energy has added value in areas that are too cloudy or dark for strong solar energy production, especially at higher latitudes.
How big are wind turbines and how much electricity can they generate?
Typical utility-scale land-based wind turbines are about 250 feet tall and have an average capacity of 2.55 megawatts, each producing enough electricity for hundreds of homes. While land-based wind farms may be remote, most are easy to access and connect to existing power grids.
Smaller turbines, often used in distributed systems that generate power for local use rather than for sale, average about 100 feet tall and produce between 5 and 100 kilowatts.
One type of offshore wind turbine currently in development stands 853 feet tall, four-fifths the height of the Eiffel Tower, and can produce 13 megawatts of power. Adjusted for variations in wind, that is enough to consistently power thousands of homes. While tall offshore turbines lack some of the advantages of land-based wind farms, use of them is burgeoning because they can capture the energy of powerful, reliable winds high in the air near coastlines, where most of the largest cities in the world are located.
What are some potential future wind technologies other than turbines?
Engineers are in the early stages of creating airborne wind turbines , in which the components are either floated by a gas like helium or use their own aerodynamics to stay high in the air, where wind is stronger. These systems are being considered for offshore use, where it is expensive and difficult to install conventional wind turbines on tall towers.
Trees, which can withstand gale forces and yet move in response to breezes from any direction, also are inspiring new ideas for wind energy technology. Engineers speculate about making artificial wind-harvesting trees . That would require new materials and devices that could convert energy from a tree's complex movements into the steady rotation that traditional generators need. The prize is wind energy harvested closer to the ground with smaller, less obtrusive technologies and in places with complex airflows, such as cities.
What are the challenges of using wind energy?
Extreme winds challenge turbine designers. Engineers have to create systems that will start generating energy at relatively low wind speeds and also can survive extremely strong winds. A strong gale contains 1,000 times more power than a light breeze, and engineers don't yet know how to design electrical generators or turbine blades that can efficiently capture such a broad range of input wind power. To be safe, turbines may be overbuilt to withstand winds they will not experience at many sites, driving up costs and material use. One potential solution is the use of long-term weather forecasting and AI to better predict the wind resources at individual locations and inform designs for turbines that suit those sites.
Climate change will bring more incidents of unusual weather, including potential changes in wind patterns . Wind farms may help mitigate some of the harmful effects of climate change. For example, turbines in cold regions are routinely winterized to keep working in icy weather when other systems may fail, and studies have demonstrated that offshore wind farms may reduce the damage caused by hurricanes . A more challenging situation will arise if wind patterns shift significantly. The financing for wind energy projects depends critically on the ability to predict wind resources at specific sites decades into the future. One potential way to mitigate unexpected, climate-change-related losses or gains of wind is to flexibly add and remove groups of smaller turbines, such as vertical-axis wind turbines , within existing large-scale wind farms.
Wind farms do have environmental impacts . The most well-known is harm to wildlife, including birds and bats . Studies are informing wind farm siting and management practices that minimize harm to wildlife , and Audubon, a bird conservation group, now supports well-planned wind farms. The construction and maintenance of wind farms involves energy-intensive activities such as trucking, road-building, concrete production, and steel construction. Also, while towers can be recycled, turbine blades are not easily recyclable. In hopes of developing low-to-zero-waste wind farms, scientists aim to design new reuse and disposal strategies , and recyclable plastic turbine blades. Studies show that wind energy's carbon footprint is quickly offset by the electricity it generates and is among the lowest of any energy source .
Dive Deeper
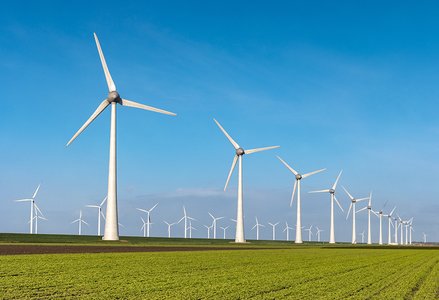
- Utility Menu

The Down Side to Wind Power

Research led by David Keith finds wind farms cause more environmental impact than previously thought
SEAS Communications
By Leah Burrows, SEAS Communications
When it comes to energy production, there's no such thing as a free lunch, unfortunately.
As the world begins its large-scale transition toward low-carbon energy sources, it is vital that the pros and cons of each type are well understood and the environmental impacts of renewable energy, small as they may be in comparison to coal and gas, are considered.
In two papers — published today in the journals Environmental Research Letters and Joule — Harvard University researchers find that the transition to wind or solar power in the U.S. would require five to 20 times more land than previously thought, and, if such large-scale wind farms were built, would warm average surface temperatures over the continental U.S. by 0.24 degrees Celsius.
"Wind beats coal by any environmental measure, but that doesn't mean that its impacts are negligible," said David Keith, the Gordon McKay Professor of Applied Physics at the Harvard John A. Paulson School of Engineering and Applied Sciences (SEAS) and senior author of the papers. "We must quickly transition away from fossil fuels to stop carbon emissions. In doing so, we must make choices between various low-carbon technologies, all of which have some social and environmental impacts."
Keith is also professor of public policy at the Harvard Kennedy School.
One of the first steps to understanding the environmental impact of renewable technologies is to understand how much land would be required to meet future U.S. energy demands. Even starting with today's energy demands, the land area and associated power densities required have long been debated by energy experts.
In previous research, Keith and co-authors modeled the generating capacity of large-scale wind farms and concluded that real-world wind power generation had been overestimated because they neglected to accurately account for the interactions between turbines and the atmosphere.
"The direct climate impacts of wind power are instant, while the benefits of reduced emissions accumulate slowly."
— david keith.
In 2013 research, Keith described how each wind turbine creates a "wind shadow" behind it where air has been slowed down by the turbine's blades. Today's commercial-scale wind farms carefully space turbines to reduce the impact of these wind shadows, but given the expectation that wind farms will continue to expand as demand for wind-derived electricity increases, interactions and associated climatic impacts cannot be avoided.
What was missing from this previous research, however, were observations to support the modeling. Then, a few months ago, the U.S. Geological Survey released the locations of 57,636 wind turbines around the U.S. Using this data set, in combination with several other U.S. government databases, Keith and postdoctoral fellow Lee Miller were able to quantify the power density of 411 wind farms and 1,150 solar photovoltaic plants operating in the U.S. during 2016.
"For wind, we found that the average power density — meaning the rate of energy generation divided by the encompassing area of the wind plant — was up to 100 times lower than estimates by some leading energy experts," said Miller, who is the first author of both papers. "Most of these estimates failed to consider the turbine-atmosphere interaction. For an isolated wind turbine, interactions are not important at all, but once the wind farms are more than five to 10 kilometers deep, these interactions have a major impact on the power density."
The observation-based wind power densities are also much lower than important estimates from the U.S. Department of Energy and the Intergovernmental Panel on Climate Change.
For solar energy, the average power density (measured in watts per meter squared) is 10 times higher than wind power, but also much lower than estimates by leading energy experts.
This research suggests that not only will wind farms require more land to hit the proposed renewable energy targets but also, at such a large scale, would become an active player in the climate system.
The next question, as explored in the journal Joule, was how such large-scale wind farms would impact the climate system.
"If your perspective is the next 10 years, wind power actually has — in some respects — more climate impact than coal or gas. If your perspective is the next thousand years, then wind power has enormously less climatic impact than coal or gas."
To estimate the impacts of wind power, Keith and Miller established a baseline for the 2012ÔÇÆ2014 U.S. climate using a standard weather-forecasting model. Then, they covered one-third of the continental U.S. with enough wind turbines to meet present-day U.S. electricity demand. The researchers found this scenario would warm the surface temperature of the continental U.S. by 0.24 degrees Celsius, with the largest changes occurring at night when surface temperatures increased by up to 1.5 degrees. This warming is the result of wind turbines actively mixing the atmosphere near the ground and aloft while simultaneously extracting from the atmosphere's motion.
This research supports more than 10 other studies that observed warming near operational U.S. wind farms. Miller and Keith compared their simulations to satellite-based observational studies in North Texas and found roughly consistent temperature increases.
Miller and Keith are quick to point out the unlikeliness of the U.S. generating as much wind power as they simulate in their scenario, but localized warming occurs in even smaller projections. The follow-on question is then to understand when the growing benefits of reducing emissions are roughly equal to the near-instantaneous impacts of wind power.
The Harvard researchers found that the warming effect of wind turbines in the continental U.S. was actually larger than the effect of reduced emissions for the first century of its operation. This is because the warming effect is predominantly local to the wind farm, while greenhouse gas concentrations must be reduced globally before the benefits are realized.
Miller and Keith repeated the calculation for solar power and found that its climate impacts were about 10 times smaller than wind's.
"The direct climate impacts of wind power are instant, while the benefits of reduced emissions accumulate slowly," said Keith. "If your perspective is the next 10 years, wind power actually has — in some respects — more climate impact than coal or gas. If your perspective is the next thousand years, then wind power has enormously less climatic impact than coal or gas.
"The work should not be seen as a fundamental critique of wind power," he said. "Some of wind's climate impacts will be beneficial — several global studies show that wind power cools polar regions. Rather, the work should be seen as a first step in getting more serious about assessing these impacts for all renewables. Our hope is that our study, combined with the recent direct observations, marks a turning point where wind power's climatic impacts begin to receive serious consideration in strategic decisions about decarbonizing the energy system."
This research was funded by the Fund for Innovative Climate and Energy Research.
Filter by Type
- Faculty in the News (149) Apply Faculty in the News filter
- General (86) Apply General filter
- HUCE Headlines (10) Apply HUCE Headlines filter
Research Areas
- Architecture & Environmental Design (14) Apply Architecture & Environmental Design filter
- Arts & Humanities (27) Apply Arts & Humanities filter
- Business, Law & Policy (81) Apply Business, Law & Policy filter
- Ecology & Biodiversity (60) Apply Ecology & Biodiversity filter
- Climate Change (107) Apply Climate Change filter
- Energy (42) Apply Energy filter
- Human Health (41) Apply Human Health filter
- Social Sciences (26) Apply Social Sciences filter
- Faculty of Arts and Sciences (67) Apply Faculty of Arts and Sciences filter
- Harvard Paulson School of Engineering and Applied Sciences (53) Apply Harvard Paulson School of Engineering and Applied Sciences filter
- Harvard Kennedy School (38) Apply Harvard Kennedy School filter
- Harvard T.H. Chan School of Public Health (25) Apply Harvard T.H. Chan School of Public Health filter
- Harvard Law School (20) Apply Harvard Law School filter
- Harvard Business School (17) Apply Harvard Business School filter
- Graduate School of Design (12) Apply Graduate School of Design filter
- University-Wide (11) Apply University-Wide filter
- Harvard Medical School (9) Apply Harvard Medical School filter
- Harvard Divinity School (6) Apply Harvard Divinity School filter
- Harvard Extension School (5) Apply Harvard Extension School filter
- Harvard Graduate School of Education (5) Apply Harvard Graduate School of Education filter
- Radcliffe Institute for Advanced Study (1) Apply Radcliffe Institute for Advanced Study filter

- Wind Energy Technologies Office
- Wind Energy Career Map
- WETO Budget
- WETO Organization & Contacts
- Atmosphere to Electrons
- Distributed Wind
- Environmental Impacts & Siting
- Next-Generation Wind Technology
- Demonstration
- Floating Offshore Wind Shot
- Market Acceleration
- R&D Consortium
- Renewable Systems Integration
- Resource Assessment & Characterization
- Testing & Certification
- Drivetrains
- Infrastructure & Logistics
- Wind Turbine Radar Interference
- Wind Turbine Sustainability
- Workforce Development & Education
- History of Wind Energy
- How Distributed Wind Works
- How Wind Turbines Work
- WINDExchange
- Small Wind Systems FAQs
- WETO Peer Reviews
- Wind Energy FAQs
- Wind Energy Market Reports
- Wind Energy Projects Map
- Related Opportunities
- Wind Energy Technologies Office Updates
- Subscribe to our Newsletters
- Wind R&D Newsletter
Wind energy offers many advantages, which explains why it's one of the fastest-growing energy sources in the world. To further expand wind energy’s capabilities and community benefits, researchers are working to address technical and socio-economic challenges in support of a decarbonized electricity future.
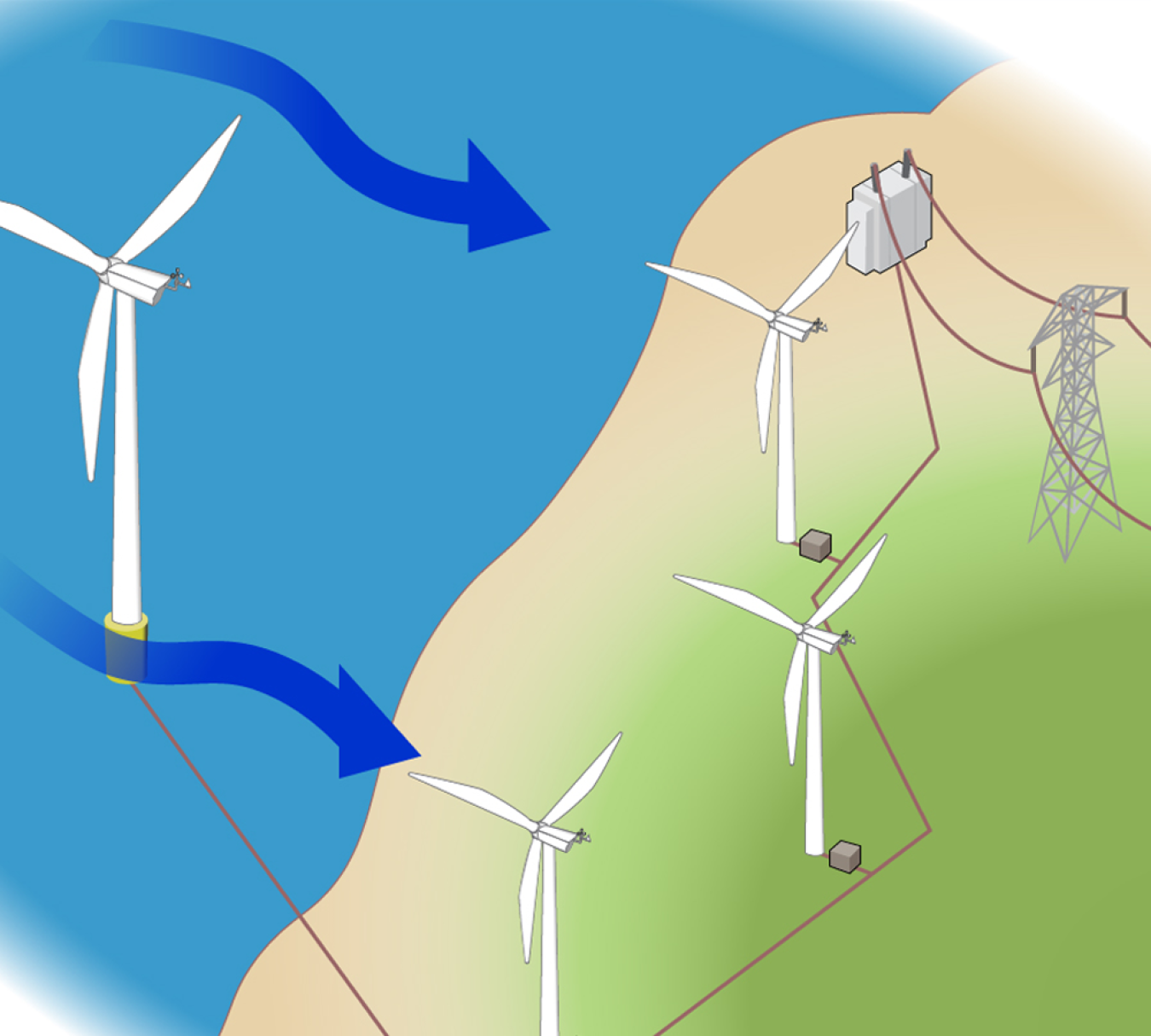
Learn more about ongoing research to take advantage of these benefits and tackle wind energy challenges.
Advantages of Wind Power
- Wind power creates good-paying jobs. There are over 125,000 people working in the U.S. wind industry across all 50 states, and that number continues to grow. According to the U.S. Bureau of Labor Statistics , wind turbine service technicians are the fastest growing U.S. job of the decade. Offering career opportunities ranging from blade fabricator to asset manager, the wind industry has the potential to support hundreds of thousands of more jobs by 2050.
- Wind power is a domestic resource that enables U.S. economic growth. In 2022, wind turbines operating in all 50 states generated more than 10% of the net total of the country’s energy . That same year, investments in new wind projects added $20 billion to the U.S. economy.
- Wind power is a clean and renewable energy source. Wind turbines harness energy from the wind using mechanical power to spin a generator and create electricity. Not only is wind an abundant and inexhaustible resource, but it also provides electricity without burning any fuel or polluting the air. Wind energy in the United States helps avoid 336 million metric tons of carbon dioxide emissions annually —equivalent to the emissions from 73 million cars.
- Wind power benefits local communities. Wind projects deliver an estimated $2 billion in state and local tax payments and land-lease payments each year. Communities that develop wind energy can use the extra revenue to put towards school budgets, reduce the tax burden on homeowners, and address local infrastructure projects.
- Wind power is cost-effective. Land-based, utility-scale wind turbines provide one of the lowest-priced energy sources available today. Furthermore, wind energy’s cost competitiveness continues to improve with advances in the science and technology of wind energy.
- Wind turbines work in different settings. Wind energy generation fits well in agricultural and multi-use working landscapes. Wind energy is easily integrated in rural or remote areas, such as farms and ranches or coastal and island communities, where high-quality wind resources are often found.
Challenges of Wind Power
- Wind power must compete with other low-cost energy sources. When comparing the cost of energy associated with new power plants , wind and solar projects are now more economically competitive than gas, geothermal, coal, or nuclear facilities. However, wind projects may not be cost-competitive in some locations that are not windy enough. Next-generation technology , manufacturing improvements , and a better understanding of wind plant physics can help bring costs down even more.
- Ideal wind sites are often in remote locations. Installation challenges must be overcome to bring electricity from wind farms to urban areas, where it is needed to meet demand. Upgrading the nation’s transmission network to connect areas with abundant wind resources to population centers could significantly reduce the costs of expanding land-based wind energy. In addition, offshore wind energy transmission and grid interconnection capabilities are improving.
- Turbines produce noise and alter visual aesthetics. Wind farms have different impacts on the environment compared to conventional power plants, but similar concerns exist over both the noise produced by the turbine blades and the visual impacts on the landscape .
- Wind plants can impact local wildlife. Although wind projects rank lower than other energy developments in terms of wildlife impacts, research is still needed to minimize wind-wildlife interactions . Advancements in technologies, properly siting wind plants, and ongoing environmental research are working to reduce the impact of wind turbines on wildlife.
- Wind Energy Advantages and Disadvantages
- Energy 101: Resources to Help Understand Energy
- Energy Innovation
Wind energy advantages and disadvantages are important considerations when making decisions about energy with the environment in mind. A cleaner future will involve a mix of energy sources, including those that are renewable like wind power.
Wind is produced as the sun heats the earth unevenly due to the earth’s rotation and geographic features. Warm air rises to create low-pressure areas, while cold air sinks to create high-pressure areas. Air molecules typically move from high pressure areas to low ones, creating the phenomenon we experience as wind.
Harnessing wind as energy is an idea that is thousands of years old. Because it does not involve burning limited fossil fuels and because using wind energy does not decrease the amount of wind, it is considered a renewable energy source. While there are wind power advantages and disadvantages, wind energy has a valuable role to play in a climate-friendly power grid.
Advantages of Wind Energy
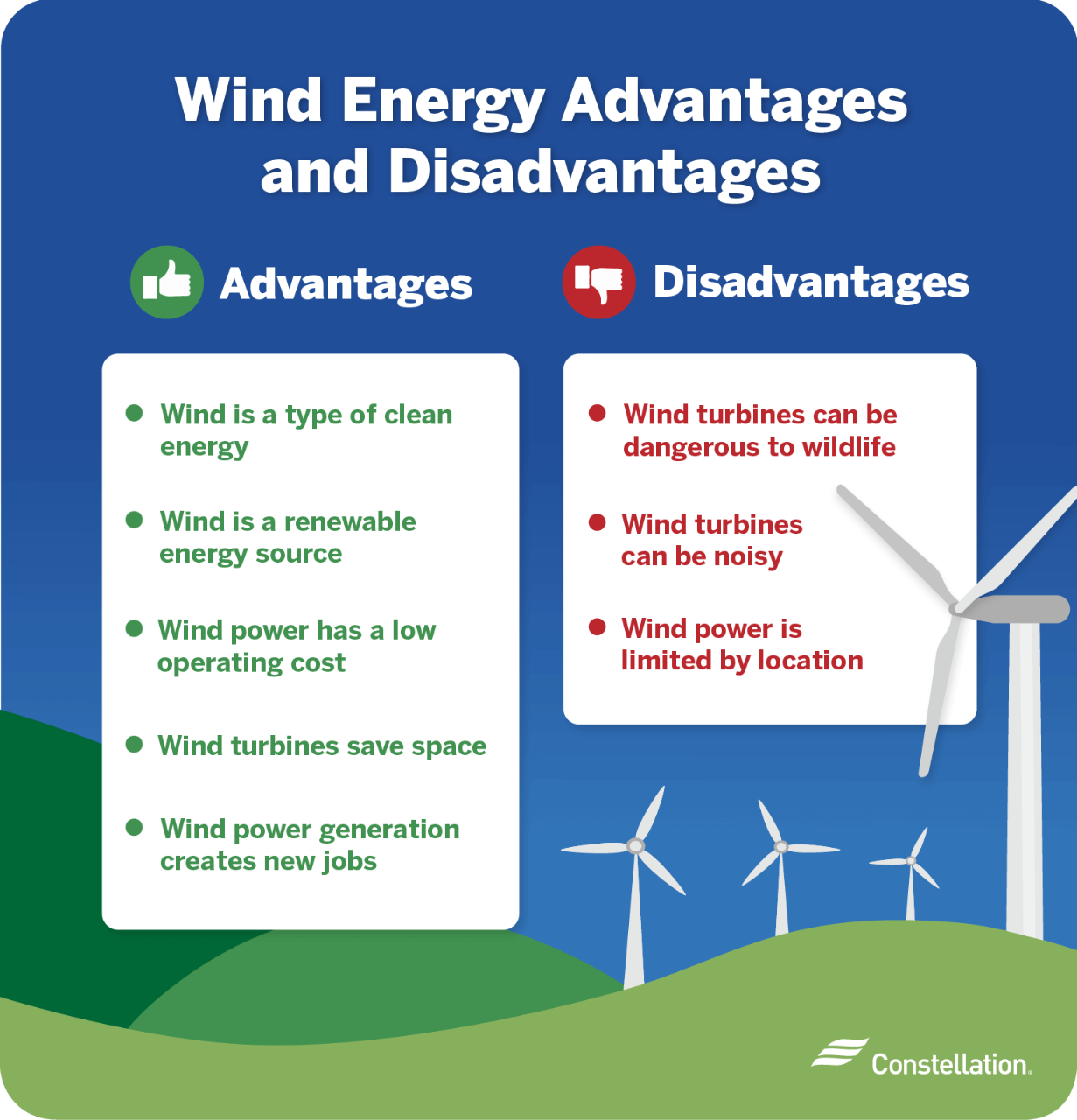
In considering wind power pros and cons, the advantages of wind energy are many. These are the reasons for investing in wind power generating capacity.
1. Wind is a type of clean energy
First off, how does wind energy work? It starts with a turbine that the wind turns as it blows. The wind’s kinetic energy turns a generator in the structure that creates electricity. Modern wind turbines are extremely efficient at turning even light breezes into electricity.
One of the advantages of wind energy is that it is clean energy , meaning that it doesn’t emit greenhouse gasses when generating electricity. Your carbon footprint is the total of all gasses, like carbon dioxide, methane, and nitrous oxide, that result from burning fossil fuels. If you can burn less fossil fuel for energy, replacing it with clean, renewable energy like from wind, you reduce your carbon footprint .
2. Wind is a renewable energy source
Another advantage of wind energy is that it is renewable energy. It comes from wind, which is a naturally occurring resource that doesn’t get used up. How is green energy different from renewable energy? In addition to coming from an unlimited resource, it does not produce greenhouse gasses. In these ways, wind energy is similar to solar energy .
3. Wind power has a low operating cost
Because wind power is a renewable energy source , there is no ongoing expense to acquire fuel. Once the wind turbine is installed, the only real cost is maintenance. As the world decarbonizes electricity generation in the future, wind is a clean, renewable, and low-cost option.
4. Wind turbines save space
Another factor in the wind power pros and cons equation is the fact that it is space efficient and fits well with other land uses. Wind turbines can be spread across fields with enough space between them to be productive. Because they are elevated off the ground the space below them is open to other uses, like farming.
5. Wind power generation promotes domestic economic growth
Harnessing wind power is economically beneficial beyond wind energy being inexpensive to produce. It relies on a local resource and creates local jobs . Investment in wind power is growing. In the last 10 years, wind generation capacity has increased 30 percent. Utility-scale wind plants across 41 states have created more than 100,000 jobs in the U.S.
Disadvantages of Wind Energy
We must consider both wind energy advantages and disadvantages when weighing the benefits of this renewable energy source. Indeed, there are disadvantages to wind power.
1. Wind turbines can be dangerous to some wildlife
Wind turbines can be fatal to wildlife. Birds and bats collide with them and turbines interfere with bat sonar navigation. Certain species with low reproductive rates are more impacted, as are migratory birds, but researchers are investigating innovative ways to reduce the danger to wildlife.
2. Wind turbines can be noisy
Wind turbines create both aerodynamic noise of the blades slicing through the air and mechanical noise of the power generating machinery in them. The noise can affect wildlife but is generally not a factor unless you are standing nearby.
3. Wind power is limited by location
Wind power won’t work everywhere. You need areas where wind blows strong and steady to make the investment worthwhile. Even with ideal locations in coastal areas, hills, and open fields where the wind is especially strong, it doesn’t blow all the time. Energy generation slows or stops when the wind slows or stops, a concept known as “intermittency.”
Learn More about Wind Energy from Constellation
As you make your home more sustainable , consider the residential renewable plans available in many areas, including wind energy plans. You can compare renewable energy rates and choose the option that best fit your needs. With an appreciation of wind energy advantages and disadvantages, you can make choices that benefit the environment.
Zip Code is not valid
Environmental Impacts of Wind Power
Published Mar 5, 2013

How Wind Energy Works
Harnessing power from the wind is one of the cleanest and most sustainable ways to generate electricity as it produces no toxic pollution or global warming emissions. Wind is also abundant, inexhaustible, and affordable, which makes it a viable and large-scale alternative to fossil fuels.
Despite its vast potential, there are a variety of environmental impacts associated with wind power generation that should be recognized and mitigated.
The land use impact of wind power facilities varies substantially depending on the site: wind turbines placed in flat areas typically use more land than those located in hilly areas. However, wind turbines do not occupy all of this land; they must be spaced approximately 5 to 10 rotor diameters apart (a rotor diameter is the diameter of the wind turbine blades). Thus, the turbines themselves and the surrounding infrastructure (including roads and transmission lines) occupy a small portion of the total area of a wind facility.
A survey by the National Renewable Energy Laboratory of large wind facilities in the United States found that they use between 30 and 141 acres per megawatt of power output capacity (a typical new utility-scale wind turbine is about 2 megawatts). However, less than 1 acre per megawatt is disturbed permanently and less than 3.5 acres per megawatt are disturbed temporarily during construction [ 1 ]. The remainder of the land can be used for a variety of other productive purposes, including livestock grazing, agriculture, highways, and hiking trails [ 2 ]. Alternatively, wind facilities can be sited on brownfields (abandoned or underused industrial land) or other commercial and industrial locations, which significantly reduces concerns about land use [ 3 ].
Offshore wind facilities require larger amounts of space because the turbines and blades are bigger than their land-based counterparts. Depending on their location, such offshore installations may compete with a variety of other ocean activities, such as fishing, recreational activities, sand and gravel extraction, oil and gas extraction, navigation, and aquaculture. Employing best practices in planning and siting can help minimize potential land use impacts of offshore and land-based wind projects [ 4 ].
Wildlife and habitat
The impact of wind turbines on wildlife, most notably on birds and bats, has been widely document and studied. A recent National Wind Coordinating Committee (NWCC) review of peer-reviewed research found evidence of bird and bat deaths from collisions with wind turbines and due to changes in air pressure caused by the spinning turbines, as well as from habitat disruption. The NWCC concluded that these impacts are relatively low and do not pose a threat to species populations [ 5 ].
Additionally, research into wildlife behavior and advances in wind turbine technology have helped to reduce bird and bat deaths. For example, wildlife biologists have found that bats are most active when wind speeds are low. Using this information, the Bats and Wind Energy Cooperative concluded that keeping wind turbines motionless during times of low wind speeds could reduce bat deaths by more than half without significantly affecting power production [ 6 ]. Other wildlife impacts can be mitigated through better siting of wind turbines. The U.S. Fish and Wildlife Services has played a leadership role in this effort by convening an advisory group including representatives from industry, state and tribal governments, and nonprofit organizations that made comprehensive recommendations on appropriate wind farm siting and best management practices [ 7 ].
Offshore wind turbines can have similar impacts on marine birds, but as with onshore wind turbines, the bird deaths associated with offshore wind are minimal. Wind farms located offshore will also impact fish and other marine wildlife. Some studies suggest that turbines may actually increase fish populations by acting as artificial reefs. The impact will vary from site to site, and therefore proper research and monitoring systems are needed for each offshore wind facility [ 8 ].
Public health and community
Sound and visual impact are the two main public health and community concerns associated with operating wind turbines. Most of the sound generated by wind turbines is aerodynamic, caused by the movement of turbine blades through the air. There is also mechanical sound generated by the turbine itself. Overall sound levels depend on turbine design and wind speed.
Some people living close to wind facilities have complained about sound and vibration issues, but industry and government-sponsored studies in Canada and Australia have found that these issues do not adversely impact public health [ 9 ]. However, it is important for wind turbine developers to take these community concerns seriously by following “good neighbor” best practices for siting turbines and initiating open dialogue with affected community members. Additionally, technological advances, such as minimizing blade surface imperfections and using sound-absorbent materials can reduce wind turbine noise [ 10 ].
Under certain lighting conditions, wind turbines can create an effect known as shadow flicker. This annoyance can be minimized with careful siting, planting trees or installing window awnings, or curtailing wind turbine operations when certain lighting conditions exist [ 11 ].
The Federal Aviation Administration (FAA) requires that large wind turbines, like all structures over 200 feet high, have white or red lights for aviation safety. However, the FAA recently determined that as long as there are no gaps in lighting greater than a half-mile, it is not necessary to light each tower in a multi-turbine wind project. Daytime lighting is unnecessary as long as the turbines are painted white.
When it comes to aesthetics, wind turbines can elicit strong reactions. To some people, they are graceful sculptures; to others, they are eyesores that compromise the natural landscape. Whether a community is willing to accept an altered skyline in return for cleaner power should be decided in an open public dialogue [ 12 ].
There is no water impact associated with the operation of wind turbines. As in all manufacturing processes, some water is used to manufacture steel and cement for wind turbines.
Life-cycle global warming emissions
While there are no global warming emissions associated with operating wind turbines, there are emissions associated with other stages of a wind turbine’s life-cycle, including materials production, materials transportation, on-site construction and assembly, operation and maintenance, and decommissioning and dismantlement.
Estimates of total global warming emissions depend on a number of factors, including wind speed, percent of time the wind is blowing, and the material composition of the wind turbine [ 13 ]. Most estimates of wind turbine life-cycle global warming emissions are between 0.02 and 0.04 pounds of carbon dioxide equivalent per kilowatt-hour. To put this into context, estimates of life-cycle global warming emissions for natural gas generated electricity are between 0.6 and 2 pounds of carbon dioxide equivalent per kilowatt-hour and estimates for coal-generated electricity are 1.4 and 3.6 pounds of carbon dioxide equivalent per kilowatt-hour [ 14 ].
References:
[1] Denholm, P., M. Hand, M. Jackson, and S. Ong. 2009. Land-use requirements of modern wind power plants in the United States . Golden, CO: National Renewable Energy Laboratory.
[2] National Renewable Energy Laboratory (NREL). 2012. Renewable Electricity Futures Study . Hand, M.M.; Baldwin, S.; DeMeo, E.; Reilly, J.M.; Mai, T.; Arent, D.; Porro, G.; Meshek, M.; Sandor, D. eds. 4 vols. NREL/TP-6A20-52409. Golden, CO: National Renewable Energy Laboratory.
[3] National Renewable Energy Laboratory (NREL). June 14, 2010. Brownfields' Bright Spot: Solar and Wind Energy . Online at http://www.nrel.gov/news/features/feature_detail.cfm/feature_id=1530
[4] Michel, J.; Dunagan, H.; Boring, C.; Healy, E.; Evans, W.; Dean, J.; McGillis, A.; Hain, J. 2007. Worldwide Synthesis and Analysis of Existing Information Regarding Environmental Effects of Alternative Energy Uses on the Outer Continental Shelf . MMS 2007-038. Prepared by Research Planning and ICF International. Herndon, VA: U.S. Department of the Interior, Minerals Management Service.
[5] National Wind Coordinating Committee (NWCC). 2010. Wind turbine interactions with birds, bats, and their habitats: A summary of research results and priority questions.
[6] Arnett, E.B., M.M.P. Huso, J.P. Hayes, and M. Schirmacher. 2010. Effectiveness of changing wind turbine cut-in speed to reduce bat fatalities at wind facilities . A final report submitted to the Bats and Wind Energy Cooperative. Austin, TX: Bat Conservation International.
[7] Fish and Wildlife Service (FSW). 2010. Recommendations of the wind turbine guidelines advisory committee .
[8] Michel, et al. 2007.
[9] Chief Medical Officer of Heath of Ontario. 2010. The potential health impact of wind turbines . Toronto, Ontario: Ontario Ministry of Health and Long Term Care.
American Wind Energy Association (AWEA) and the Canadian Wind Energy Association (CanWEA). 2009. Wind turbine sound and health effects: An expert panel review .
National Health and Medical Research Council (NHMRC). 2010. Wind turbines and health: A rapid review of the evidence . Canberra, Australia: National Health and Medical Research Council.
[10] Bastasch, M.; van Dam, J.; Søndergaard, B.; Rogers, A. 2006. Wind Turbine Noise – An Overview . Canadian Acoustics (34:2), 7–15.
[11] National Renewable Energy Laboratory (NREL). 2012. Renewable Electricity Futures Study .
[12] Union of Concerned Scientists. Tapping into Wind .
[13] National Academy of Sciences. 2010. Electricity from Renewable Resources: Status, Prospects, and Impediments .
[14] IPCC, 2011: I PCC Special Report on Renewable Energy Sources and Climate Change Mitigation . Prepared by Working Group III of the Intergovernmental Panel on Climate Change [O. Edenhofer, R. Pichs-Madruga, Y. Sokona, K. Seyboth, P. Matschoss, S. Kadner, T. Zwickel, P. Eickemeier, G. Hansen, S. Schlömer, C. von Stechow (eds)]. Cambridge University Press, Cambridge, United Kingdom and New York, NY, USA, 1075 pp. (Chapter 7 & 9).
Related resources
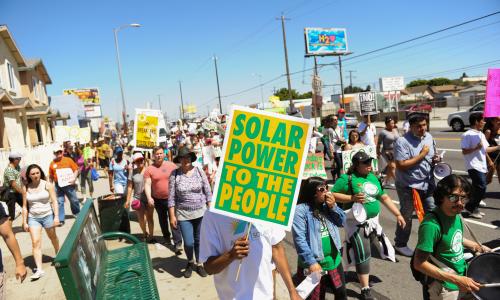
The Electric Utility Toolkit

Feet To The Fire
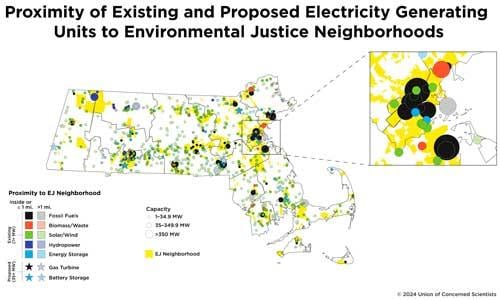
Siting for a Cleaner, More Equitable Grid in Massachusetts

Barriers and Solutions to Building Clean Energy in California
We use cookies to improve your experience. By continuing, you accept our use of cookies. Learn more .
Support our work
Other ways to give.
- Honor & memory
- Become a member
- Give monthly
- Make a planned gift
- Gift memberships
Your donation at work
Large-scale wind power would require more land and cause more environmental impact than previously thought
Wind beats coal by any environmental measure, but that doesn't mean that its impacts are negligible.
When it comes to energy production, there’s no such thing as a free lunch, unfortunately.
As the world begins its large-scale transition towards low-carbon energy sources, it is vital that the pros and cons of each type are well understood and the environmental impacts of renewable energy, small as they may be in comparison to coal and gas, are considered.
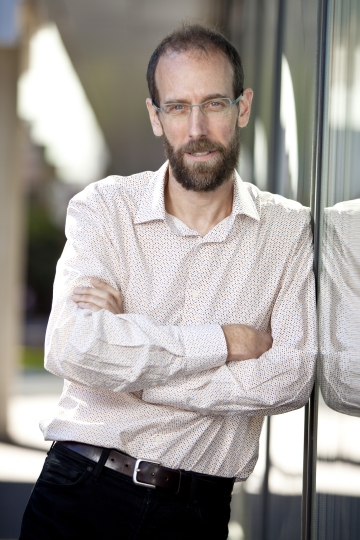
David Keith, the Gordon McKay Professor of Applied Physics at the Harvard John A. Paulson School of Engineering and Applied Sciences (Image courtesy of Eliza Grinnell/Harvard SEAS)
In two papers — published today in Environmental Research Letters and Joule — Harvard University researchers find that the transition to wind or solar power in the United States would require five to 20 times more land area than previously thought, and if such large-scale wind farms were built, would warm average surface temperatures over the continental United States by 0.24 degrees Celsius.
"Wind beats coal by any environmental measure, but that doesn't mean that its impacts are negligible," said David Keith , the Gordon McKay Professor of Applied Physics at the Harvard John A. Paulson School of Engineering and Applied Sciences (SEAS) and senior author of the papers. "We must quickly transition away from fossil fuels to stop carbon emissions. In doing so, we must make choices between various low-carbon technologies, all of which have some social and environmental impacts."
Keith is also Professor of Public Policy at the Harvard Kennedy School.
One of the first steps to understanding the environmental impact of renewable technologies is to understand how much land area would be required to meet future U.S. energy demands. Even starting with today’s energy demands, the land area and associated power densities required have long been debated by energy experts.
In previous research, Keith and co-authors modeled the generating capacity of large-scale wind farms and concluded that real-world wind power generation had been overestimated because they neglected to accurately account for the interactions between turbines and the atmosphere. In 2013 research, Keith described how each wind turbine creates a "wind shadow" behind it where air has been slowed down by the turbine's blades. Today’s commercial-scale wind farms carefully space turbines to reduce the impact of these wind shadows, but given the expectation that wind farms will continue to expand as demand for wind-derived electricity increases, interactions and associated climatic impacts cannot be avoided.
What was missing from this previous research, however, were observations to support the modeling. Then, a few months ago, the United States Geological Survey released the location of 57,636 wind turbines around the U.S. Using this dataset, in combination with several other US government databases, Keith and postdoctoral fellow Lee Miller were able to quantify the power density of 411 wind farms and 1,150 solar photovoltaic plants operating in the U.S. during 2016.
“For wind, we found that the average power density — meaning the rate of energy generation divided by the encompassing area of the wind plant — was up to 100-times lower than estimates by some leading energy experts,” said Miller, who is the first author of both papers. “Most of these estimates failed to consider the turbine-atmosphere interaction. “For an isolated wind turbine, interactions are not important at all, but once the wind farms are more than five to 10 kilometers deep, these interactions have a major impact on the power density.”
The observation-based wind power densities are also much lower than important estimates from the US Department of Energy (DOE) and the Intergovernmental Panel on Climate Change (IPCC).
For solar energy, the average power density (measured in watts per meter squared) is 10 times higher than wind power, but also much lower than estimates by leading energy experts.
This research suggests that not only will wind farms require more land area to hit the proposed renewable energy targets but also, at such large scale, would become an active player in the climate system.
The next question, as explored in the journal Joule, was how would such large-scale wind farms impact the climate system.

Graph of large-scale direct observation of wind energy’s power density is consistent with physically-based models and inconsistent with wind resource estimates that ignore interactions between wind turbines and the atmosphere (Image courtesy of Lee Miller/David Keith)
To estimate the impacts of wind power, Keith and Miller established a baseline for the 2012-2014 U.S. climate using a standard weather forecasting model. Then, they covered one third of the continental U.S. with enough wind turbines to meet present-day U.S. electricity demand. The researchers found this scenario would warm the surface temperature of the continental U.S. by 0.24 degrees Celsius, with the largest changes occurring at night when surface temperatures increased by up to 1.5ºC. This warming is the result of wind turbines actively mixing the atmosphere near the ground and aloft while simultaneously extracting from the atmosphere's motion.
This research supports more than ten other studies that observed warming near operational US wind farms. Miller and Keith compared their simulations to satellite-based observational studies in North Texas and found roughly consistent temperature increases.
Miller and Keith are quick to point out the unlikeliness of the U.S. generating as much wind power as they simulate in their scenario, but localized warming occurs in even smaller projections. The follow-on question is then to understand when the growing benefits of reducing emissions are roughly equal to the near-instantaneous impacts of wind power.
The Harvard researchers found that the warming effect in the continental U.S. caused by wind turbines is actually larger than the effect of reduced emissions for the first century of its operation. This is because the warming effect is predominantly local to the wind farm, while greenhouse gas concentrations must be reduced globally before the benefits are realized.
Miller and Keith repeated the calculation for solar power and found that its climate impacts are about ten times smaller than wind’s.
"The direct climate impacts of wind power are instant, while the benefits of reduced emissions accumulate slowly," says Keith. "If your perspective is the next 10 years, wind power actually has--in some respects--more climate impact than coal or gas. If your perspective is the next thousand years, then wind power has enormously less climatic impact than coal or gas."
"The work should not be seen as a fundamental critique of wind power,” said Keith. “Some of wind's climate impacts will be beneficial — several global studies show that wind power cools polar regions. Rather, the work should be seen as a first step in getting more serious about assessing these impacts for all renewables. Our hope is that our study, combined with the recent direct observations, marks a turning point where wind power's climatic impacts begin to receive serious consideration in strategic decisions about decarbonizing the energy system."
This research was funded by the Fund for Innovative Climate and Energy Research.
Topics: Climate
Cutting-edge science delivered direct to your inbox.
Join the Harvard SEAS mailing list.
Scientist Profiles
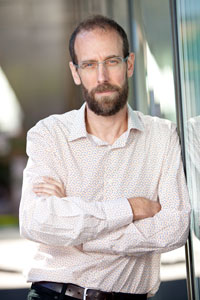
David Keith
Associate in Environmental Science and Engineering
Press Contact
Leah Burrows | 617-496-1351 | [email protected]
Related News
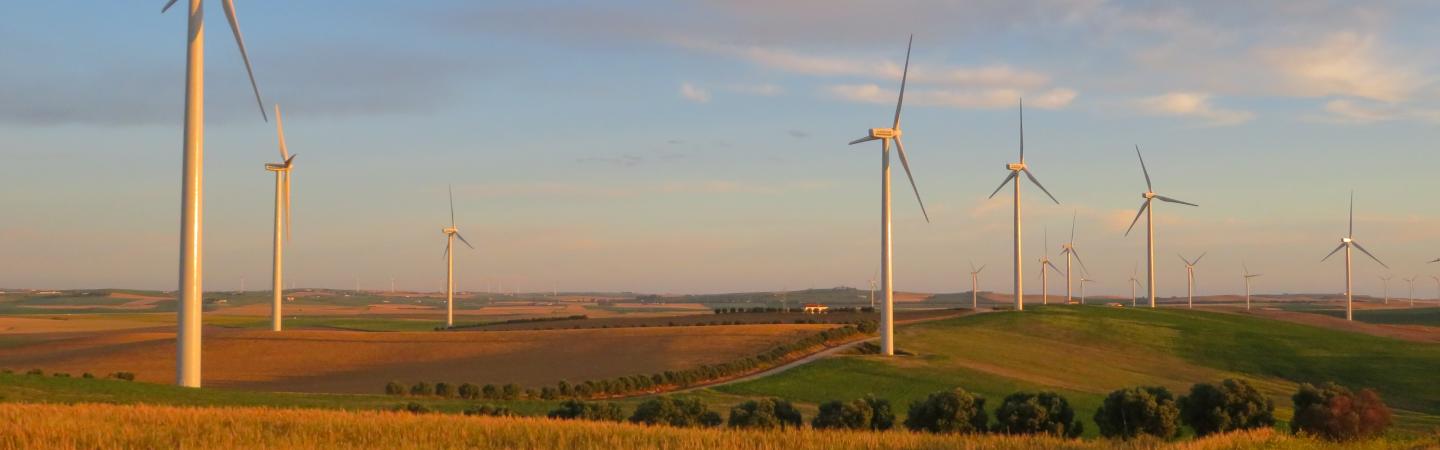
New to Climate Change?
Wind energy.
Wind energy is a form of renewable energy , typically powered by the movement of wind across enormous fan-shaped structures called wind turbines. Once built, these turbines create no climate-warming greenhouse gas emissions , making this a “carbon-free” energy source that can provide electricity without making climate change worse. Wind energy is the third-largest source of carbon-free electricity in the world (after hydropower and nuclear ) 1 and the second-fastest-growing (after solar ). 2
Cheap, clean energy
The major reason for wind energy’s success is that it’s cheap. In fact, the International Energy Agency estimates that an onshore wind farm built today will make electricity at a lower average cost than any other form of new-built energy. 3 We can thank recent advances in wind turbine technology, and economies of scale from its rapid growth, for this ultra-cheap energy. 4 Wind turbines aren’t “pushed” like sails catching the wind: they actually work more like airplane wings, with blades shaped so that wind flows unequally fast above and below them. This creates an area of high pressure on one side and low pressure on the other, which “lifts” the blades toward the low-pressure area and makes them turn, powering a generator that makes electricity. Over the past 40 years, turbine blades have become longer and lighter, letting them turn faster with less wind. Modern turbines also pivot automatically to catch the wind at the best angle. These and other advances have led the price of wind energy to fall almost 95% since 1980. 5 Wind energy is also remarkably clean, even compared to other types of carbon-free energy like solar and hydropower. Building new wind turbines does create some greenhouse gas emissions—from making the steel for their towers and fiberglass for their blades, and mining the rarer minerals sometimes used in their generators. But even factoring that in, a wind turbine creates only around a quarter of the greenhouse gas emissions of a solar panel for every kilowatt of electricity, and only a little over 1% the greenhouse gas emissions of a coal-fired power plant. 6 And future innovations could make wind energy even cheaper and cleaner. Researchers are experimenting with new materials and construction techniques, as well as designs very different from the familiar “horizontal axis turbine” with its three blades rotating like a pinwheel. “Vertical axis turbines” spin instead like a carousel, while “airborne wind energy” looks more like a kite or plane tethered to a generator on the ground.
Wind power in the larger energy system
Wind energy is “variable”: how much electricity it produces depends on how much wind is blowing. In any energy system that relies partly on wind, other energy sources have to be ramped up when winds are low. Energy storage (saving some energy for later when wind turbines are over-producing) and long-distance transmission (moving electricity from places with lots of wind to places with lots of demand) can help the energy system rely more heavily on wind power around the clock. Wind energy also needs wide stretches of open space. The average wind turbine in the U.S. is around 300 feet tall, and its blades span a circle over 400 feet wide—longer than a football field. 7 These turbines are spaced far apart, sometimes by half a mile or more, so they won’t compete for wind. If you include the entire area of a wind farm in its land footprint, wind farms can take up tens of thousands of acres and make less electricity per acre than any other energy source except bioenergy . 8 However, if you only include the land directly affected by the footprint of each turbine, wind power consumes much less land. Wind energy is unique in how easily it can share land with other uses. In the U.S., around 90% of wind turbines are built on cropland or rangeland for grazing animals, most of it actively used. 9 In this sense, wind energy “takes up” hardly any land at all. Wind turbines can also be built offshore, sharing space with fishing and shipping. Offshore wind is more expensive than onshore wind, but it takes advantage of stronger, more consistent wind to provide reliable electricity, and is less visible to people living nearby. 10 For built-up coastal regions like the northeastern U.S., where energy demand is high and open land is scarce, offshore wind may be the best way to make clean, renewable energy at a large scale.
Published May 22, 2023.
1 International Energy Agency: Electricity . (Updated February 16, 2023.)
2 International Energy Agency: Renewables 2021: Executive Summary .
3 International Energy Agency: Projected Costs of Generating Electricity 2020 .
4 Wiser, Ryan, et al . " Expert elicitation survey predicts 37% to 49% declines in wind energy costs by 2050 ." Nature Energy 6, 2021, doi:10.1038/s41560-021-00810-z.
5 U.S. Department of Energy Office of Energy Efficiency and Renewable Energy: " U.S. Department of Energy's Wind Energy Technologies Office—Lasting Impressions ." January 2021.
6 Intergovernmental Panel on Climate Change: "Climate Change 2014: Mitigation of Climate Change. Contribution of Working Group III to the Fifth Assessment Report of the Intergovernmental Panel on Climate Change ." Annex III: Technology-Specific Cost and Performance Parameters . 2014.
7 U.S. Department of Energy Office of Energy Efficiency and Renewable Energy: " Wind Turbines: The Bigger, the Better ." August 16, 2022.
8 Lovering, Jessica, et al. " Land-use intensity of electricity production and tomorrow's energy landscape ." PLoS One 17(7), July 2022, doi:10.1371/journal.pone.0270155.
9 U.S. Department of Agriculture: " Wind Energy Land Distribution in the United States of America ." July 2017.
10 U.S. Department of the Interior Bureau of Ocean Energy Management: Renewable Energy on the Outer Continental Shelf . Accessed May 22, 2023.
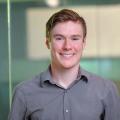
More Resources for Learning
Want to learn more.
Listen to this episode of MIT's "Today I Learned: Climate" podcast on wind and solar power.
Keep exploring
With more Explainers from our library:
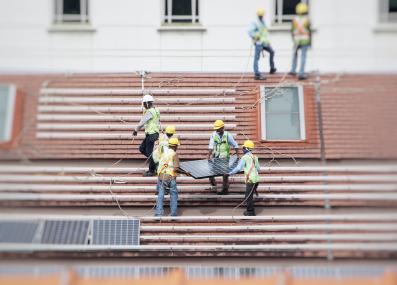
Renewable Energy
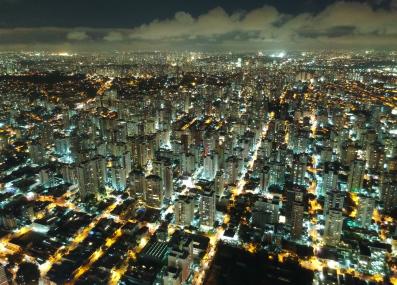
Energy Storage

The Electric Grid
Mit climate news in your inbox.
February 14, 2014
Wind Power Found to Affect Local Climate
Wind farms can alter the nearby rainfall and temperature, suggesting a need for more comprehensive studies of future energy systems
By Daniel Kirk-Davidoff & The Conversation
Editor's note: The following essay is reprinted with permission from The Conversation , an online publication covering the latest research.
Wind turbines take energy from the atmosphere and turn it into electricity: so we know they must have some impact on the atmosphere’s flow. With industrial grade turbines being built at a terrific rate, scientists have been trying to assess exactly what the effects are both at local and at global levels.
New research using a finely resolved model which focuses on Europe indicates that the effects of doubling the present wind capacity by 2020 are at the level of a couple of percent changes in rain and a few tenths of a degree of temperature. The study by Vautard and colleagues shows that these effects include a mix of more rain and less rain, and warming and cooling, depending on where you are around Europe. But a key policy question left unresolved by this paper is: to what should these changes be compared?
On supporting science journalism
If you're enjoying this article, consider supporting our award-winning journalism by subscribing . By purchasing a subscription you are helping to ensure the future of impactful stories about the discoveries and ideas shaping our world today.
Measuring impact There are two main ways of estimating the climate impact of wind turbines. One is to compare the real climate of a region before and after wind farms are installed there. This approach is limited by the availability of observations – modern wind farms are a relatively new phenomenon so there is not much data to compare the climate after wind farms are installed with the years before. Ruling out other reasons for the climate changes we observe is also a challenge.
Using this observational approach, researchers have found that the climate around a large wind farm in Texas was affected by the presence of the turbines. Taking the ground temperatures measured by satellites, they detected a warming of 0.5°C at night in the region directly under the farm. This warming effect was local and small – the nighttime warming did not extend beyond the farm’s immediate neighborhood.
But not many people live right among the wind turbines. To look at smaller effects far from the wind farms, another approach is to simulate the earth’s climate with computer models. Using climate history data, projections are made that account for motion, thermodynamics, chemistry and radiation in the atmosphere, land and sea. Years of simulations are run with and without wind farms installed.
This method has the advantage of being able to detect very small climate change signals, since the model can be run for a long time, and natural variability gets smaller as you average over more and more years. Also, all factors besides wind that might cause climate changes can be held constant. The disadvantage is that the model of climate behavior may not correspond exactly to what happens in reality.
This approach was first attempted by David Keith and colleagues in 2004. When they compared the climate in their model with and without extremely large wind farms (large enough to generate about twice the world’s total present electrical demand), they found that in addition to climate effects in the immediate vicinity of the wind farms, there were changes in climate all around the world.
There were regions of warming and cooling of about 0.5°C, and increases and decreases in precipitation by a few percent. A followup paper showed that these changes were mostly a result of changes in wind direction caused by the wind turbines. The model winds tended to shy away from the wind farms a little, so that downstream from the farms there would be regions of extra wind from the south, that would tend to be warmer, and regions of extra wind from the north, that would tend to be colder.
Vautard’s study agrees with this earlier work, in finding that the climate impacts of wind farms extend beyond the farms themselves and are caused by changes in the flow of the atmosphere that bring warming and cooling to different regions around the wind farms.
What’s the right comparison? So how should we understand these results? Keith and colleagues compared the climate changes caused by wind turbines with the climate changes that would have been caused by fossil fuel burned to make the same amount of electricity. But because carbon dioxide lasts for centuries or millennia in the atmosphere (unless we suck it out somehow), it’s hard to know over how many years to calculate the avoided carbon dioxide emissions. For the 20-year life of the turbines? Or for the few hundred years of supply of fossil fuels that could be burnt?
Now that the impacts of wind turbines on climate are becoming better understood, more comprehensive studies of complete future energy systems are needed. We need to ask, what combination of wind power, solar power, nuclear power and fossil fuel power, together with what combination of measures to remove carbon from the atmosphere, will result in the lowest overall environmental and social costs.
Daniel Kirk-Davidoff works for a company (MDA Information Systems LLC) that provides wind generation forecasts for energy industry clients including wind farm owners.
This article was originally published on The Conversation . Read the original article .
- Skip to main content
- Keyboard shortcuts for audio player
Misinformation is derailing renewable energy projects across the United States
Julia Simon

A wind turbine is silhouetted against the sky at sunset near Ellsworth, Kansas. The 300-foot-tall turbine is among the 134 units that make up the Post Rock Wind Farm. Charlie Riedel/AP hide caption
A wind turbine is silhouetted against the sky at sunset near Ellsworth, Kansas. The 300-foot-tall turbine is among the 134 units that make up the Post Rock Wind Farm.
On a winter night in early 2016, Jeremy Kitson gathered in his buddy's large shed with some neighbors to plan their fight against a proposed wind farm in rural Van Wert County, Ohio. The project would be about a mile from his home.
From the beginning, Kitson — who teaches physics and chemistry at the local high school — knew he didn't want the turbines anywhere near him. He had heard from folks who lived near another wind project about 10 miles away that the turbines were noisy and that they couldn't sleep.
"There were so many people saying that it's horrible, you do not want to live under these things,'" Kitson says.
He and his neighbors went on the offensive. "I was just like, there's got to be a way to beat 'em," he says of the developer, Apex Clean Energy. "You got to outsmart them. You got to figure out the science. You got to figure out the economic arguments. You got to figure out what they're going to say and figure out how to counter it."
At the shed, according to Kitson, they agreed that part of their outreach would involve posting information on a Facebook community page called "Citizens for Clear Skies," which ultimately grew to more than 770 followers.
In between posts selling anti-wind yard signs and posts about public meetings opposing local wind projects, there were posts that spread false, misleading and questionable information about wind energy. Links to stories about wind turbine noise causing birth defects in Portuguese horses . Posts about the health effects of low frequency infrasound , also called wind turbine syndrome . Posts about wind energy not actually reducing carbon dioxide emissions . Photos of wind turbines breaking, burning and falling — some in nearby counties and states, but some in Germany and New Zealand . According to 2014 data from the Department of Energy, the most recent available, out of the then-40,000 turbines in the U.S., there had been fewer than 40 incidents.

An anti-wind turbine sign stands in the front yard of a farmhouse in Glenville, Minn., in January 2018. Opponents of wind power are successfully stalling or rejecting wind farm projects across the country. Charlie Neibergall/AP hide caption
An anti-wind turbine sign stands in the front yard of a farmhouse in Glenville, Minn., in January 2018. Opponents of wind power are successfully stalling or rejecting wind farm projects across the country.
Kitson, the administrator of the Facebook page, says he knows that these accidents aren't typical. "Those events are not likely. We know that," Kitson says. But Kitson has seen a broken piece of a fallen turbine blade himself, which got him worrying about how the fiberglass might affect the integrity of the soil and the crops. So he posts the photos and articles, many of which he receives from an anti-wind email list. "I do that just to try to show people what's possible."
Kitson's group is one of dozens in the United States and abroad that oppose utility-scale wind and solar projects. Researchers say that in many groups, misinformation is raising doubts about renewable energy and slowing or derailing projects.
The opposition comes at a time when climate scientists say the world must shift quickly away from fossil fuels to avoid the worst impacts of climate change . But 60% of U.S. electricity still comes from carbon-based fuels.
For the Biden administration to hit its target of an electricity sector free of fossil fuels by 2035, the country has to double or triple the wind and solar power capacity it installs over the next few years and maintain that higher level of deployments for about a decade, says Kelly Speakes-Backman, who leads the Energy Department's Office of Energy Efficiency and Renewable Energy.

President Biden takes notes during a meeting to discuss clean energy efforts with CEOs of U.S. electric utility companies at the White House in February 2022. Anna Moneymaker/Getty Images hide caption
President Biden takes notes during a meeting to discuss clean energy efforts with CEOs of U.S. electric utility companies at the White House in February 2022.
Yet every single rural utility-scale wind and solar project needs local or state approval to get built, says Sarah Mills , who researches rural renewable energy at the University of Michigan. And she says it's in those often-fractious discussions about approval that misinformation is sometimes halting and stalling the installation of the renewables the climate needs. "At the end of the day, if local governments are not setting rules that allow for the infrastructure to be sited, those policies cannot be achieved," Mills says.
Misinformation gets mixed up in decisions over renewable projects
Last year, a Department of Energy study found that setback regulations now represent the single-greatest barrier to securing locations for wind projects in the U.S. Setbacks limit how close wind projects can be to buildings, and Mills says they often make sense to reduce things such as noise and "shadow flicker," the moving shadows and strobing sunlight that turbines can cast onto buildings. But she says misinformation can fuel setbacks that are more stringent than needed and sometimes act as outright bans on renewable energy.
In Ohio, setbacks and other rules associated with renewable projects have historically been set at the state level. But in October, a new law, SB 52, went into effect giving counties the ability to make exclusion zones with no utility-scale wind and solar projects.
Kitson, the science teacher, testified in support of the zones , arguing that turbines negatively affect property values. He pointed to his group's analysis comparing the lower property values in the one local township that has wind turbines to the higher average property values in the greater county.
But Ben Hoen, a researcher at the Lawrence Berkeley National Laboratory, says his more than 15 years of research has shown that wind turbines have little to no impact on nearby property values. Hoen says, "We have not found evidence of property value impacts despite studying it over multiple periods of time."

Wind turbines dot the countryside in Okarche, Okla., in 2021. Sue Ogrocki/AP hide caption
Wind turbines dot the countryside in Okarche, Okla., in 2021.
Hoen does say that studies in the Netherlands and United Kingdom have found some effects on property values, but they were far smaller than Kitson's reference to studies showing a 20%-40% depreciation.
In about half of states, regulations around how and whether to build rural utility-scale solar and wind are determined on the local level, Mills says. "These local officials are not necessarily experts in energy," she says. "And so when you have people coming and stating things as facts, especially if there's nobody fact-checking everything, right, it's difficult. They're certainly making decisions based on what they're hearing."
Facebook groups spread misleading content
In recent years, some of the misinformation about renewable energy has come from former President Donald Trump, who frequently makes misleading and false anti-wind claims at his rallies and media appearances, including the untrue idea that wind turbine noise causes cancer. Earlier this month, when asked about the unfolding Ukraine crisis on a podcast, Trump immediately responded by listing untrue ideas about wind energy.
Other misleading ideas about renewable energy come from groups with ties to the fossil fuel industry, like the Texas Public Policy Foundation. The foundation recently released a film trailer for an anti-offshore wind group in Massachusetts that features multiple falsehoods, including the untrue statement that the proposed project didn't do any environmental impact assessments and the incorrect idea that offshore wind projects "haven't worked anywhere in the world." The Texas Public Policy Foundation did not respond to a request for comment.
But Facebook is one of the biggest drivers of misleading content about renewable energy, says Josh Fergen, a researcher at the University of Minnesota Duluth. Last fall, Fergen and his colleagues published a paper looking at the Facebook posts of Kitson's group and another large wind opposition group, about 90 kilometers east, fighting the Republic Wind Farm.
Fergen's paper concluded that posts in the two Facebook pages were "increasing perceptions of human health and public safety risks related to wind by sharing news of disasters and misinformation over health assessment risks." In June, the Ohio Power Siting Board, whose approval was needed for the site, rejected the Republic Wind Farm proposal citing geological concerns and the local opposition.
NPR reviewed dozens of posts from anti-wind and anti-solar groups. While some posts about climate change denial, lithium mining , and a quote misattributed to Winston Churchill were marked as inaccurate, there were dozens of posts with misleading information about renewable energy that were not tagged.
NPR sent Facebook a sampling of the posts from anti-renewable community pages. Facebook spokesman Kevin McAlister said in an emailed statement, "We take action against content that our fact-checking partners rate false as part of our comprehensive strategy to keep viral, provably false claims from spreading on our apps. The examples shared with us don't appear to meet that threshold as they have only even been shared a handful of times over a period of several years."
But Fergen says that these same types of misleading and false posts about wind and solar energy pop up in a network of Facebook groups around the country, feeding a conflict between rural communities and energy developers.
Leah Stokes, an associate professor of political science at the University of California, Santa Barbara, says as resistance to wind and solar projects spreads on social media, the dangers of misinformation from these anti-renewable Facebook groups is growing.
" It can really slow down the clean energy transition, and that has just as dire life and death consequences, not just in terms of climate change, but also in terms of air pollution, which overwhelmingly hits communities of color."

Lobstermen and their families attend a 2021 rally to protest Maine Gov. Janet Mills' support for offshore wind projects in Augusta, Maine. Robert F. Bukaty/AP hide caption
Lobstermen and their families attend a 2021 rally to protest Maine Gov. Janet Mills' support for offshore wind projects in Augusta, Maine.
"It's about who you trust"
Speakes-Backman says the Department of Energy is trying to do more outreach to local communities about inaccurate ideas surrounding utility-scale solar and wind, especially around land use and environmental effects. "We want to make sure that we are counteracting the misinformation that may be out there," she says.
But Dahvi Wilson, vice president of public affairs for Apex Clean Energy, says her company is finding that across the country, local engagement is becoming increasingly difficult given community suspicions of renewable energy.
"I think for a long time, and maybe still in some places, developers thought, 'Well, we just need to give better information. We just need to give more information.' And it's like, 'it's so not about that at all!'" Wilson says. "It's about who you trust and if anybody's going to believe you if you're a company."
Hoen of the Lawrence Berkeley National Laboratory says the perception of the community engagement process regarding renewables has real implications — even on human health. He gives the example of shadow flicker, the moving shadows from turbines, which opposition groups often cite when critiquing wind. A few states and several counties have regulations limiting shadow flicker on habited structures, commonly to about 30 hours per year, but most do not, and wind opposition groups argue that the strobing shadows can cause agitation, headaches, or even seizures in some individuals.
Hoen says to date they have not found any evidence of shadow flicker causing seizures in research they've reviewed, but they have found its relation to annoyance and stress. So Hoen's group did a study asking: "As the number of shadow flicker hours decrease, is there less annoyance? Are people less bothered by it?"
"What we found, interestingly, is that the individuals that were annoyed by shadow flicker did not necessarily have a higher level of shadow flicker at their home than those that weren't annoyed," Hoen says. Another study found stress symptoms were correlated with how people felt about the fairness of the project's roll-out. "And, in fact, what led to that annoyance, it appears, is their perception of the planning process, how they felt like that development got rolled out in their community."
Given the importance of community engagement in the process of locating wind and solar, Mills says renewable proponents need to be careful that they aren't romanticizing the projects or providing misleading information themselves, for example, by saying that a wind or solar plant will bring lots of jobs to an area.
"There are a lot of jobs in renewable energy. Many, many of the jobs in renewable energy are in construction trades. And so once the project is built, there's not tons of jobs associated with the project," Mills says. "I think in all of this, it's important to not sugarcoat."
Anti-renewable groups have internal disputes over use of misinformation
In the last few months, more states — Washington, Iowa and Kansas — have proposed bills to restrict rural utility-scale wind and solar. In Kansas, these bills were proposed by state Sen. Mike Thompson, who also introduced a bill to shut down existing renewable projects.
Thompson, a former meteorologist, is a longtime critic of renewable energy. One of his anti-wind videos even popped up on Kitson's anti-wind Facebook group .

Kansas Republican state Sen. Mike Thompson (left) during a 2022 meeting at the Statehouse in Topeka. Thompson denies the link between human activity and climate change and has a key role in energy policy as the state Senate's utilities committee chairman. John Hanna/AP hide caption
Kansas Republican state Sen. Mike Thompson (left) during a 2022 meeting at the Statehouse in Topeka. Thompson denies the link between human activity and climate change and has a key role in energy policy as the state Senate's utilities committee chairman.
In one video on the anti-solar group's YouTube channel , Thompson calls climate change "one of the biggest scams out there" and says "carbon dioxide has no correlation with the temperature on this planet whatsoever." That statement is false: The vast majority of scientists agree that the climate crisis stems from greenhouse gas emissions generated by human activity. Thompson did not respond to requests for comment.
YouTube spokesperson Elena Hernandez said in an emailed statement, "In general, we don't recommend or prominently surface content that includes climate change misinformation. Our systems are trained to raise videos from authoritative sources, like news outlets and experts, in search results for certain queries related to climate change and renewable energy."
Barbara Kerr is a professor of psychology at the University of Kansas and she's a founding member of that anti-solar group in Kansas, which opposes NextEra Energy's proposed utility-scale solar plant in Douglas and Johnson counties. Kerr says she knows the videos that have been featured by the group she co-founded have misinformation.
"Just horrible," Kerr says of the videos. "They are just counterfactual and not something we should have on the website."
But despite Kerr's objections, her group decided in January to keep the videos online. "It is important to not judge, and censor utility-scale solar content/opinions contributed by citizens. If we become judge and jury, we are headed down the wrong path," the group said in an emailed statement.
Kerr says that while she disagrees with the misinformation used by some in her group, she says the anti-solar coalition makes for "strange bedfellows." "Sometimes you have to compromise," she says. "I don't want to alienate these people. They go to the meetings in Douglas County and Johnson County."
But Dan Reuman, professor of ecology and evolutionary biology at the University of Kansas, says he worries about the role misinformation could play in the decision-making over the solar project, which he supports. He says that while he is sympathetic to those in his county who don't want to live near a large-scale solar plant, he also thinks their concerns need to be weighed against the need to mitigate climate change.
"I just find it upsetting," Reuman says. "I hope that the government doesn't make a compromise between a scientifically based position and a misinformation-based position. Because if you're compromising with misinformation, then there's sort of no limit to that, right?"

A windfarm near Velva, N.D. The 213-foot wind towers are owned by Global Renewable Energy Partners and Acciona Energia and purchased by Xcel Energy, which distributes the wind-generated electric power to its North Dakota customers. Karen Bleier/AFP via Getty Images hide caption
A windfarm near Velva, N.D. The 213-foot wind towers are owned by Global Renewable Energy Partners and Acciona Energia and purchased by Xcel Energy, which distributes the wind-generated electric power to its North Dakota customers.
Editor's note: Facebook's parent company, Meta, pays NPR to license NPR content.

Wind Energy’s Key Environmental Advantage? Low Emissions
By Kate Yandell
Posted on June 24, 2024
Q: Are wind farms harmful to the environment?
A: Like all energy sources, wind farms have some negative environmental impacts. But getting energy from wind farms results in dramatically lower greenhouse gas emissions than getting it from fossil fuels.
FULL QUESTION
I received this post on Facebook today. I know it contains a lot of claims [challenging the eco-friendliness of wind turbines] and I don’t know where to start in terms of validating them (for myself). Can you help out?
FULL ANSWER
A genre of lengthy posts on social media claims to poke holes in measures designed to reduce greenhouse gas emissions and mitigate climate change. The posts throw out numerous statistics without citations, making it difficult to quickly sort fact from fiction.
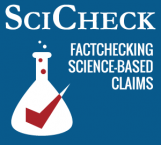
A reader recently contacted us asking for help evaluating one such post , which cast doubt on the eco-friendliness of wind energy. The post has been circulating on Facebook since March 2021, racking up more than 135,000 shares. Other versions of the post have also been spreading on social media.
Some statistics and statements in the post are arguably accurate, while others are misleading or flat-out wrong. But perhaps more important is what the post left out. It referenced the petroleum products used while building, operating, maintaining and decommissioning wind turbines without ever stating that over its entire life cycle, a wind turbine produces among the lowest greenhouse gas emissions of the main electricity sources in the U.S.
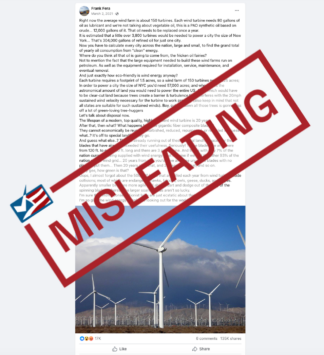
The post also misleadingly said that the land for wind farms “would have to be clear-cut land” and referenced the need to cut down “all those trees.” But in the U.S., wind farms are largely located on land that is already not forested, and this is likely to remain the case as wind energy expands.
The post discussed the landfill space needed for decommissioned wind turbine blades without providing the context that, even as wind power increases, they will make up a small percentage of all waste, and without mentioning efforts to recycle them. And it referenced bird deaths from wind farms without putting them in the context of far greater threats to birds, such as collisions with glass buildings and vehicles.
We consulted experts on wind energy to get to the bottom of the claims made in the post, and to provide context on the challenges associated with this source of renewable energy.
Wind Turbines Generate Low Emissions Over Their Lifetimes
The major advantage of wind turbines is that, taking into account their entire lifespan, they generate very low emissions compared with fossil fuels.
Researchers often calculate greenhouse gas emissions associated with methods of electricity generation using a technique called life cycle assessment . In the case of wind energy, this includes emissions generated in the course of “extraction and processing of materials, fabrication of components, transportation, installation, operations and maintenance … decommissioning, and disposal or recycling,” Aubryn Cooperman , an engineering analyst at the National Renewable Energy Laboratory, told us via email.
“Each of these processes may have emissions associated with the equipment used, such as trucks, cranes, ships, etc.,” she said. “Petroleum-based fuels are used for most types of transportation and portable equipment, and the related emissions are accounted for in the wind energy lifecycle assessment.”
In the case of fossil fuels, a life cycle assessment of greenhouse gas emissions also includes emissions caused by burning the fuel, such as coal or natural gas.
A report from NREL reviewed studies of life cycle greenhouse gas emissions for various sources of electricity. The median published estimates of emissions associated with wind were more than 37 times lower than those from natural gas and 77 times lower than those from coal.
Over their lifetimes, wind farms also are associated with very little generation of air pollutants such as particulate matter and ground-level ozone.
Despite this, the Facebook post misleadingly implied that because petroleum products can be used in various ways in building, operating, maintaining and removing wind turbines, this means that they do not qualify as clean energy.
First, the post presented various statistics on the lubricant oil in wind turbines, used in varying amounts depending on the turbine. To be clear, this oil is used to lubricate the moving parts in wind turbines and is distinct from fuels such as gasoline that are combusted and produce greenhouse gas emissions.
“Now you have to calculate every city across the nation, large and small, to find the grand total of yearly oil consumption from ‘clean’ energy,” the post said. “Where do you think all that oil is going to come from, the fricken oil fairies?”
The post went on to describe the use of petroleum products by “the large equipment needed to build these wind farms,” as well as service, maintain and remove them.
As we’ve said, emissions from petroleum product-powered equipment associated with wind farms are included in life cycle emissions calculations, and these indicate that wind farms are far cleaner sources of energy than fossil fuels.
“It’s also worth noting that as the transportation sector decarbonizes, that will reduce emissions in the wind energy lifecycle as well,” Cooperman said.
As for the lubricant oil used in wind turbines, this oil does need to be changed from time to time, which can be challenging given the locations and height of the turbines. However, it does not necessarily need to be changed annually, contrary to what the post said.
“Oil change interval on wind turbines has been extended over the years and nowadays, it is typical to do every 5 or 7 years,” Shawn Sheng , a senior research engineer at NREL, told us in an email. “Some research is being done by extending this even further to ten years or longer, such as fill-for-life (~20 years) oil.”
Emissions associated with changing this oil are included in life cycle emissions calculations, Cooperman said.
Many machines require lubricant oil, and the wind industry makes up only a fraction of the market for these products. Furthermore, lubricants only make up 1% of petroleum products produced in U.S. refineries.
Post Distorts Wind Farm Land Use Impacts
Next, the Facebook post listed various statistics about wind farm land use, mixing somewhat plausible figures with estimates based on bad math and misleading statements about impacts on forests.
“And just exactly how eco-friendly is wind energy anyway?” the post said. “Each turbine requires a footprint of 1.5 acres, so a wind farm of 150 turbines needs 225 acres…” This estimate is roughly plausible if considering the direct footprint of a relatively new, average-sized land-based wind turbine, but it doesn’t acknowledge the wide variation in turbine size.
Grace Wu , who studies land use and climate change mitigation at UC Santa Barbara, told us via email that the science and engineering community would not calculate the space requirements of a wind farm per turbine, but rather per unit of energy the wind farm can produce.
“The larger the wind turbine the more spacing it required, so to generalize across wind turbines itself (treating the unit as the wind turbine) is inaccurate,” she said. Wind turbines can have one megawatt of capacity to over 10 megawatts of capacity, Wu explained. Offshore wind turbines can be greater than 20 megawatts.
A May 2024 U.S. Department of Agriculture report on utility-scale solar and wind installations in rural areas said that the typical direct footprint of a wind farm is approximately 0.74 acres per megawatt of capacity. The direct footprint of a wind farm includes “the relatively small area on which service roads, turbine pads, and other infrastructure are constructed,” the report said.
Wind turbine capacity has been increasing. The average land-based wind turbine installed in 2022 can generate 3.2 megawatts of electricity, according to a report from the U.S. Department of Energy. Based on this, the average land-based turbine installed in 2022 would have a direct footprint of more than two acres.
Wind farms also have an indirect footprint, the USDA report explained , since wind turbines need sufficient spacing between them to best take advantage of wind flow. However, the land between wind turbines often can still be used for other purposes — typically for growing crops or as rangeland.
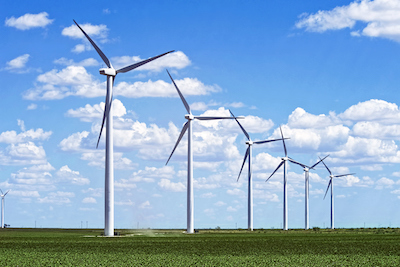
The post’s math and logic went more seriously awry as it discussed the broader impacts of wind farm land use. “In order to power a city the size of NYC you’d need 57,000 acres; and who knows the astronomical amount of land you would need to power the entire US,” the post said.
Here, the post did its own internal math incorrectly. The post previously had claimed it would take 3,800 turbines to power a city the size of New York, and 3,800 multiplied by the post’s estimated footprint of 1.5 acres per turbine is 5,700 acres, not 57,000 acres.
A spokesperson from the U.S. Energy Information Administration told us via email that it “would be impossible” to accurately calculate the number of turbines needed to power a city without knowing various other factors, including the height of the turbines, wind speed and generation capacity.
The USDA report found that in 2020, there were 88,000 acres in rural areas of the U.S. in the direct footprint of wind farms. As of 2023, 10% of U.S. electricity came from wind farms, according to the EIA. For context, the U.S. has 897 million acres of farmland.
The post’s suggestion that the entire U.S. would be solely powered by wind farms is unrealistic. According to EIA projections for 2050, even in a high-uptake scenario a minority of electricity in the U.S. would be generated by wind, with solar power showing a greater increase. “There is no scenario we have modeled in which there would be only one source of electricity generation in the United States,” the EIA spokesperson told us.
The post also did not put the land use requirements of wind farms in the context of land use requirements of other methods of generating electricity. A 2022 study published in PLOS One indicated that, when looking just at the direct footprint, natural gas and coal use more land per unit of energy generated than wind.
However, “the answer differs significantly depending on whether or not one considers spacing between turbines,” Wu said. Wind is more space-intensive than most other energy sources when taking into account the full area of wind farms.
The Facebook post misleadingly said that the land used by wind farms would all need to be clear cut. “Boy, cutting down all those trees is gonna piss off a lot of green-loving tree-huggers,” the post said.
Building wind farms does sometimes require trees to be cut down. But Wu explained that in the U.S., wind farms are largely located on existing agricultural land in the Midwest . “There are of course *some* cases of wind farms in forest land, but the vast majority of wind capacity has and will be sited in the ‘wind belt’ (midwest),” she said.
According to the USDA report , between 2012 and 2020 just around 3% of newly built wind farms were located on forest land.
Wind Turbine Blades Represent Small Proportion of All Waste
The Facebook post also painted a misleading picture of what happens to wind turbine blades at the end of their life.
“They cannot economically be reused, refurbished, reduced, repurposed, or recycled so guess what..? It’s off to special landfills they go,” the post said. “And guess what else..? They’re already running out of these special landfill spaces for the blades that have already exceeded their usefulness.”
Claire Barlow , a sustainability and materials engineer at the University of Cambridge, explained to us in an email that there are real challenges associated with the end of life of wind turbine blades. She said that the “normal lifetime” of a wind turbine is 20 to 25 years, and that the blades currently being decommissioned generally come from a time when wind energy was “starting to take off in a big way” — particularly in Europe and China. This means that “the number of end-of-life blades coming through will increase hugely in the next decade, and steadily after that.”
Putting them in landfills is the cheapest way to dispose of wind turbine blades, Barlow said. It is least expensive to simply bury the blades near the wind farms they came from, if there is space. “‘Special’ landfills yes, but not in the sense of meaning dangerous or difficult,” she said.
A Wind Energy End-of-Service Guide from the DOE explained that as of 2018, wind turbine blades sent to landfills represented 0.017% of “combined municipal solid waste and construction and demolition waste” in the U.S. The report said that by 2050 wind turbine blade waste would represent less than 0.15% of total waste, in a calculation using 2018 total waste levels.
Barlow went on to explain that in more densely populated areas, such as Northern Europe, there is less space to dispose of wind turbines via landfills and it is even banned in some areas. This means there’s an incentive to find alternate options.
“There is a great deal of work going on in finding economically (and environmentally) attractive solutions for end-of-life blade material, some of which are already commercially successful enterprises, and others that are busy scaling up from prototypes to full-scale,” she said.
The majority of wind turbine parts are readily recyclable, according to the DOE guide. “Blades are difficult to recycle because they are made from mixed materials which can’t easily be separated,” Barlow said.
The most basic recycling method, she said, “involves cutting up blades into small pieces (a few cm, or smaller for some applications) and using this material as filler in material used for road or playground surfaces, where it contributes useful strength.” There are companies using this approach, although “this recycled material isn’t high-value,” she said.
The DOE guide said that this and other recycling approaches are “increasingly being used in the United States,” although the majority of blades are still sent to landfills and the number of blades that are recycled “is difficult to determine.”
People are currently attempting to develop better processes for separating “potentially valuable fibres” out of the blades so they can be reused, Barlow said. There are challenges, but “real significant advances are being made,” she said.
The blades also “can safely be disposed of in modern well-controlled” waste-to-energy power plants, she said, although these are more common in Northern Europe than the U.S. There is also at least one operation in the U.S., she said, and others outside the country that are using wind turbine blades as fuel during the cement-making process .
Wind Turbine Impacts on Birds Need Context
Finally, the Facebook post brought up bird deaths caused by wind turbines. This, again, is a real problem, but the claims in the post are missing context, including that there are far greater causes of bird deaths.
“Oops, I almost forgot about the 500,000 birds that are killed each year from wind turbine blade collisions; most of which are endangered hawks, falcons, owls, geese, ducks, and eagles,” the post said. “Apparently smaller birds are more agile and able to dart and dodge out of the way of the spinning blades, whereas the larger soaring birds aren’t so lucky. I’m sure the wildlife conservationist folks are just ecstatic about that. I’m so glad the wind energy people are looking out for the world.”
Estimates vary for the total bird deaths from wind turbines. A 2020 report based on the American Wind Wildlife Information Center database, which compiles data from multiple studies, concluded that the median estimate for mortality was 1.3 bird deaths per megawatt of wind capacity per year.
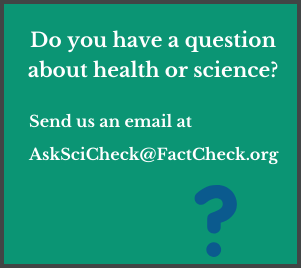
Given that the total wind power capacity of the U.S. is more than 150,000 megawatts, this would represent around 200,000 bird deaths per year. Other work, done using data from 2012 , estimated up to more than 500,000 bird deaths per year — and bird deaths would be expected to have risen as significantly more wind turbines have been built.
The Facebook post does not put wind turbine-related deaths in the context of other threats to birds. According to median estimates compiled in 2017 by the U.S. Fish and Wildlife Service, bird deaths from wind turbine collisions are dwarfed by bird deaths from collisions with building glass, estimated to kill nearly 600 million birds annually, collisions with vehicles, estimated to kill more than 200 million birds a year, and encounters with cats, estimated to kill 2.4 billion birds a year.
The post’s claim that most bird deaths are among large birds is incorrect. According to the AWWIC report , median estimates were for 1.3 small bird deaths per megawatt of wind capacity per year, compared with 0.24 deaths for large birds and 0.06 deaths for raptors.
However, it is true that researchers have particular concerns about raptor deaths from wind turbine collisions , due to their relatively small populations and reproductive life histories. Raptor deaths from wind turbines may have an outsized impact on their population size.
A final piece of context: Birds face major threats to their diversity and abundance from climate change. By providing an alternative form of energy with reduced greenhouse gas emissions, wind turbines may help mitigate climate change-related threats to birds.
Editor’s note: SciCheck’s articles providing accurate health information and correcting health misinformation are made possible by a grant from the Robert Wood Johnson Foundation. The foundation has no control over FactCheck.org’s editorial decisions, and the views expressed in our articles do not necessarily reflect the views of the foundation.
Frank Fera. “ Right now the average wind farm is about 150 turbines. Each wind turbine needs 80 gallons of oil as lubricant and we’re not talking about vegetable oil, this is a PAO synthetic oil based on crude… 12,000 gallons of it. … ” Facebook. 2 Mar 2021.
Carol Bebb McLaren. “ This was shared to me by a friend in the USA. It was written by a Man named Frank Fera. … ” Facebook. 4 Jun 2024.
Love Europe, hate the EU. “ Right now the average wind farm is about 150 turbines. Each wind turbine needs 80 gallons of oil as lubricant and we’re not talking about vegetable oil, this is a PAO synthetic oil based on crude… 12,000 gallons of it. … ” Facebook. 6 Jun 2024.
UK Keep Britain United. “ Right now the average wind farm is about 150 turbines. Each wind turbine needs 80 gallons of oil as lubricant and we’re not talking about vegetable oil, this is a PAO synthetic oil based on crude… 12,000 gallons of it. … ” Facebook. 6 Jun 2024.
National Renewable Energy Laboratory. “ Life Cycle Greenhouse Gas Emissions from Electricity Generation: Update .” Life Cycle Greenhouse Gas Emissions from Electricity Generation: Update 1. Sep 2021.
“ What is U.S. electricity generation by energy source? ” FAQ on Energy Information Agency website. Updated 29 Feb 2024.
Maguire, Karen et al. “ Utility-Scale Solar and Wind Development in Rural Areas: Land Cover Change (2009–20) .” USDA Economic Research Report Number 330. May 2024.
“ Wind Energy End-of-Service Guide .” WindExchange. Accessed 24 Jun 2024.
“ Threats to Birds .” U.S. Fish and Wildlife Service website. Accessed 24 Jun 2024.
“ Life Cycle Assessment Harmonization .” NREL website. Accessed 24 Jun 2024.
Cooperman, Aubryn. Emails to FactCheck.org. 12 and 18 Jun 2024.
“ How Wind Can Help Us Breathe Easier .” Wind Energy Technologies Office, Energy.gov. 24 Aug 2024.
“ Going Green – Wind Turbines & Lubricant Testing .” Savant Labs website. Accessed 24 Jun 2024.
Hennigan, Gary. “ Maintenance and Lubrication of Wind Turbines .” altenergymag.com. 7 Jul 2016.
Sheng, Shawn. Email to FactCheck.org. 12 Jun 2024.
“ Shell named #1 global lubricants supplier for the 17th year in a row .” Shell website. Accessed 24 Jun 2024.
“ Oil and petroleum products explained .” Energy Information Agency website. Updated 20 Jun 2024.
Wu, Grace. Email to FacCheck.org. 12 Jun 2024.
Wiser, Ryan et al. “ Land-Based Wind Market Report: 2023 Edition .” U.S. Department of Energy. 2023.
Energy Information Agency spokesperson. Email to FactCheck.org. 16 Jun 2024.
DeCarolis, Joseph and LaRose, Angelina. “ Annual Energy Outlook 2023 .” 16 Mar 2023.
“ Clearing the air: Wind farms more land efficient than previously thought .” McGill University press release. 17 Apr 2024.
Lovering, Jessica et al. “ Land-Use Intensity of Electricity Production and Tomorrow’s Energy Landscape .” PLOS One. 6 Jul 2022.
Rahman, Grace. “ Does Scotland Have an ‘Order’ to Chop down 17 Million Trees? ” Full Fact. 26 Aug 2022.
“ U.S. Wind Turbine Database .” USGS website. May 2024.
“ Power of Place – National Technical Briefing .” The Nature Conservancy. 2023.
Barlow, Claire. Email to FactCheck.org. 13 Jun 2024.
Holger, Dieter and Petroni, Gilulia. “ Cement Makers Burn Turbine Blades as Wind Power Faces Recycling Headache .” Wall Street Journal PRO. 26 Apr 2022.
“ Summary of Bird Fatality Monitoring Data Contained in AWWIC (2nd Edition) .” American Wind Wildlife Institute (AWWI). 2020.
“ U.S. Installed and Potential Wind Power Capacity and Generation .” WINDExchange. Accessed 24 Jun 2024.
Smallwood, K. Shawn. “ Comparing bird and bat fatality-rate estimates among North American wind-energy projects .” Wildlife Society Bulletin. 26 Mar 2013.
Schipani, Vanessa. “ Trump’s Hot Air on Wind Energy .” FactCheck.org. 2 Jun 2016.
Allison, Taber D. and Butryn, Ryan. “ 2nd Edition: Summary of Bird Fatality Monitoring Data Contained in AWWIC .” American Wind Wildlife Institute (AWWI). 24 Nov 2020.
Diffendorfer, Jay E. et al. “ Demographic and Potential Biological Removal Models Identify Raptor Species Sensitive to Current and Future Wind Energy .” Ecosphere. 16 Jun 2021.
“ Survival by Degrees: 389 Bird Species on the Brink .” Audubon. Accessed 24 Jun 2024.
Bateman, Brooke L. et al. “ North American Birds Require Mitigation and Adaptation to Reduce Vulnerability to Climate Change .” Conservation Science and Practice. 13 Jul 2020.
Chen, Luoye and Khanna, Madhu. “ Heterogeneous and long-term effects of a changing climate on bird biodiversity .” Global Environmental Change Advances. 26 Apr 2024.
Thank you for visiting nature.com. You are using a browser version with limited support for CSS. To obtain the best experience, we recommend you use a more up to date browser (or turn off compatibility mode in Internet Explorer). In the meantime, to ensure continued support, we are displaying the site without styles and JavaScript.
- View all journals
- Explore content
- About the journal
- Publish with us
- Sign up for alerts
- Review Article
- Published: 20 October 2020
Climate change impacts on wind power generation
- Sara C. Pryor ORCID: orcid.org/0000-0003-4847-3440 1 ,
- Rebecca J. Barthelmie ORCID: orcid.org/0000-0003-0403-6046 2 ,
- Melissa S. Bukovsky ORCID: orcid.org/0000-0001-6415-965X 3 ,
- L. Ruby Leung ORCID: orcid.org/0000-0002-3221-9467 4 &
- Koichi Sakaguchi ORCID: orcid.org/0000-0001-9672-6364 4
Nature Reviews Earth & Environment volume 1 , pages 627–643 ( 2020 ) Cite this article
4947 Accesses
129 Citations
75 Altmetric
Metrics details
- Atmospheric dynamics
- Climate-change impacts
- Climate-change mitigation
- Wind energy
Wind energy is a virtually carbon-free and pollution-free electricity source, with global wind resources greatly exceeding electricity demand. Accordingly, the installed capacity of wind turbines grew at an annualized rate of >20% from 2000 to 2019 and is projected to increase by a further 50% by the end of 2023. In this Review, we describe the factors that dictate the wind resource magnitude and variability and illustrate the tools and techniques that are being used to make projections of wind resources and wind turbine operating conditions. Natural variability due to the action of internal climate modes appears to dominate over global-warming-induced non-stationarity over most areas with large wind energy installations or potential. However, there is evidence for increased wind energy resources by the end of the current century in northern Europe and the US Southern Great Plains. New technology trends are changing the sensitivity of wind energy to global climate non-stationarity and, thus, present new challenges and opportunities for innovative research. The evolution of climate modelling to increasingly address mesoscale processes is providing improved projections of both wind resources and wind turbine operating conditions, and will contribute to continued reductions in the levelized cost of energy from wind power generation.
This is a preview of subscription content, access via your institution
Access options
Access Nature and 54 other Nature Portfolio journals
Get Nature+, our best-value online-access subscription
24,99 € / 30 days
cancel any time
Subscribe to this journal
Receive 12 digital issues and online access to articles
111,21 € per year
only 9,27 € per issue
Buy this article
- Purchase on SpringerLink
- Instant access to full article PDF
Prices may be subject to local taxes which are calculated during checkout
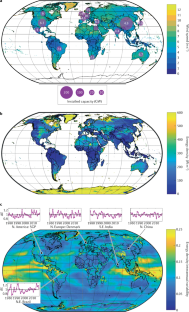
Similar content being viewed by others
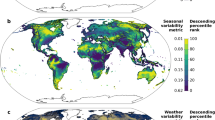
Identification of reliable locations for wind power generation through a global analysis of wind droughts
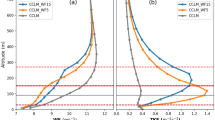
Larger wind turbines as a solution to reduce environmental impacts
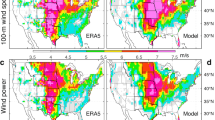
Skillful seasonal prediction of wind energy resources in the contiguous United States
Wiser, R. et al. in IPCC Special Report on Renewable Energy Sources and Climate Change Mitigation (eds Edenhofer, O. et al.) 535 (Cambridge Univ. Press, 2012). A comprehensive analysis of the technical potential wind resource, the role of wind energy in climate change mitigation and wind energy technologies .
Eurek, K. et al. An improved global wind resource estimate for integrated assessment models. Energy Econ. 64 , 552–567 (2017).
Google Scholar
Marvel, K., Kravitz, B. & Caldeira, K. Geophysical limits to global wind power. Nat. Clim. Change 3 , 118–121 (2013).
Possner, A. & Caldeira, K. Geophysical potential for wind energy over the open oceans. Proc. Natl Acad. Sci. USA 114 , 11338–11343 (2017).
Jung, C., Schindler, D. & Laible, J. National and global wind resource assessment under six wind turbine installation scenarios. Energy Convers. Manag. 156 , 403–415 (2018).
International Energy Agency. Global energy & CO 2 status (IEA, 2019).
International Energy Agency. Key world energy statistics 2019 (IEA, 2019).
Global Wind Energy Council. Global wind report 2018 (GWEC, 2019).
Veers, P. et al. Grand challenges in the science of wind energy. Science 366 , eaau2027 (2019). Emphasizes important trends within the wind energy industry and highlights resulting key research avenues .
Wiser, R. et al. Expert elicitation survey on future wind energy costs. Nat. Energy 1 , 16135 (2016).
Global Wind Energy Council. Global wind report: annual market update 2017 (GWEC, 2018).
Smoucha, E. A., Fitzpatrick, K., Buckingham, S. & Knox, O. G. Life cycle analysis of the embodied carbon emissions from 14 wind turbines with rated powers between 50KW and 3.4Mw. J. Fundam. Renew. Energy Appl. 6 , 1000211 (2016).
Barthelmie, R. J. & Pryor, S. C. Potential contribution of wind energy to climate change mitigation. Nat. Clim. Change 4 , 684–688 (2014). Quantifies the potential role of wind energy to climate change mitigation .
Wiser, R. et al. Long-term implications of sustained wind power growth in the United States: Potential benefits and secondary impacts. Appl. Energy 179 , 146–158 (2016).
Global Wind Energy Council. Global wind energy outlook 2016 (GWEC, 2016).
Intergovernmental Panel on Climate Change. Climate Change 2013. The physical science basis (Cambridge Univ. Press, 2013).
Pryor, S. C. & Barthelmie, R. J. Climate change impacts on wind energy: A review. Renew. Sustain. Energy Rev. 14 , 430–437 (2010). A comprehensive review on the topic of climate change impacts on the wind energy industry .
Pryor, S. C. & Barthelmie, R. J. Assessing the vulnerability of wind energy to climate change and extreme events. Clim. Change 121 , 79–91 (2013). Addresses climate change impacts on the conditions in which WTs operate.
Manwell, J. F., McGowan, J. G. & Rogers, A. L. Wind Energy Explained: Theory, Design and Application (Wiley, 2010).
Stull, R. B. An Introduction to Boundary Layer Meteorology (Springer, 2012).
Irwin, J. S. A theoretical variation of the wind profile power-law exponent as a function of surface roughness and stability. Atmos. Environ. 13 , 191–194 (1979).
Barthelmie, R. J., Shepherd, T. J., Aird, J. A. & Pryor, S. C. Power and wind shear implications of large wind turbine scenarios in the US Central Plains. Energies 13 , 4269 (2020).
Gryning, S.-E., Batchvarova, E., Brümmer, B., Jørgensen, H. & Larsen, S. On the extension of the wind profile over homogeneous terrain beyond the surface boundary layer. Bound. Layer Meteorol. 124 , 251–268 (2007).
Nunalee, C. G. & Basu, S. Mesoscale modeling of coastal low-level jets: implications for offshore wind resource estimation. Wind Energy 17 , 1199–1216 (2014).
Pryor, S. C., Shepherd, T. J. & Barthelmie, R. J. Interannual variability of wind climates and wind turbine annual energy production. Wind Energy Sci. 3 , 651–665 (2018).
Pryor, S. C., Nielsen, M., Barthelmie, R. J. & Mann, J. Can satellite sampling of offshore wind speeds realistically represent wind speed distributions? Part II: Quantifying uncertainties associated with sampling strategy and distribution fitting methods. J. Appl. Meteorol. 43 , 739–750 (2004).
Mann, J., Kristensen, L. & Jensen, N. O. in Bridge Aerodynamics (eds Larsen, A. & Esdahl, S.) 49–56 (Balkema, 1998).
Ramon, J., Lledó, L., Torralba, V., Soret, A. & Doblas-Reyes, F. J. What global reanalysis best represents near-surface winds? Q. J. R. Meteorol. Soc. 145 , 3236–3251 (2019).
Olauson, J. ERA5: The new champion of wind power modelling? Renew. Energy 126 , 322–331 (2018).
Garcia-Heller, V., Espinasa, R. & Paredes, S. Forecast study of the supply curve of solar and wind technologies in Argentina, Brazil, Chile and Mexico. Renew. Energy 93 , 168–179 (2016).
Bianchi, E., Solarte, A. & Guozden, T. Spatiotemporal variability of the wind power resource in Argentina and Uruguay. Wind Energy 22 , 1086–1100 (2019).
Pryor, S. C., Conrick, R., Miller, C., Tytell, J. & Barthelmie, R. J. Intense and extreme wind speeds observed by anemometer and seismic networks: An eastern US case study. J. Appl. Meteorol. Climatol. 53 , 2417–2429 (2014).
Pryor, S. C., Barthelmie, R. J. & Schoof, J. T. Inter-annual variability of wind indices across Europe. Wind Energy 9 , 27–38 (2006).
Grams, C. M., Beerli, R., Pfenninger, S., Staffell, I. & Wernli, H. Balancing Europe’s wind-power output through spatial deployment informed by weather regimes. Nat. Clim. Change 7 , 557–562 (2017).
Reichenberg, L., Wojciechowski, A., Hedenus, F. & Johnsson, F. Geographic aggregation of wind power-an optimization methodology for avoiding low outputs. Wind Energy 20 , 19–32 (2017).
International Electrotechnical Commission. IEC 61400-1:2019. Wind energy generation systems – Part 1: design requirements (IEC, 2019).
International Electrotechnical Commission. IEC 61400-3-1:2019. Wind energy generation systems. Part 3-1: design requirements for fixed offshore wind turbines (IEC, 2019).
Johansson, V. et al. Value of wind power–implications from specific power. Energy 126 , 352–360 (2017).
Jackson, K., Van Dam, C. & Yen-Nakafuji, D. Wind turbine generator trends for site-specific tailoring. Wind Energy 8 , 443–455 (2005).
Hansen, M. O. L. Aerodynamics of Wind Turbines (Routledge, 2015).
Knutson, T. et al. Tropical cyclones and climate change assessment: Part I: Detection and attribution. Bull. Am. Meteorol. Soc. 100 , 1987–2007 (2019).
Romero, R. & Emanuel, K. Climate change and hurricane-like extratropical cyclones: projections for North Atlantic polar lows and medicanes based on CMIP5 models. J. Clim. 30 , 279–299 (2017).
Davis, N., Hahmann, A. N., Clausen, N. E. & Zagar, M. Forecast of icing events at a wind farm in Sweden. J. Appl. Meteorol. Climatol. 53 , 262–281 (2014).
Sabatier, J., Lanusse, P., Feytout, B. & Gracia, S. in Informatics in Control, Automation and Robotics Vol. 495 (eds Gusikhin, O. & Madani, K.) 641–663 (Springer, 2020).
Letson, F. W., Barthelmie, R. J. & Pryor, S. C. RADAR-derived precipitation climatology for wind turbine blade leading edge erosion. Wind Energy Sci. 5 , 331–347 (2020).
Herring, R., Dyer, K., Martin, F. & Ward, C. The increasing importance of leading edge erosion and a review of existing protection solutions. Renew. Sustain. Energy Rev. 115 , 109382 (2019).
Letson, F., Shepherd, T. J., Barthelmie, R. J. & Pryor, S. C. WRF modelling of deep convection and hail for wind power applications. J. App. Meteorol. Climatol . https://doi.org/10.1175/JAMC-D-20-0033.1 (2020).
Feingold, G., Koren, I., Wang, H., Xue, H. & Brewer, W. A. Precipitation-generated oscillations in open cellular cloud fields. Nature 466 , 849–852 (2010).
Pleskachevsky, A. L., Lehner, S. & Rosenthal, W. Storm observations by remote sensing and influences of gustiness on ocean waves and on generation of rogue waves. Ocean Dyn. 62 , 1335–1351 (2012).
Larsén, X. G., Vincent, C. & Larsen, S. Spectral structure of mesoscale winds over the water. Q. J. R. Meteorol. Soc. 139 , 685–700 (2013).
Passon, P. & Branner, K. Condensation of long-term wave climates for the fatigue design of hydrodynamically sensitive offshore wind turbine support structures. Ships Offshore Struct. 11 , 142–166 (2016).
Young, I., Vinoth, J., Zieger, S. & Babanin, A. V. Investigation of trends in extreme value wave height and wind speed. J. Geophys. Res. Oceans 117 , C00J06 (2012).
Sun, C. & Jahangiri, V. Fatigue damage mitigation of offshore wind turbines under real wind and wave conditions. Eng. Struct. 178 , 472–483 (2019).
Trenberth, K. E., Dai, A., Rasmussen, R. M. & Parsons, D. B. The changing character of precipitation. Bull. Am. Meteorol. Soc. 84 , 1205–1217 (2003).
Ma, J., Xie, S.-P. & Kosaka, Y. Mechanisms for tropical tropospheric circulation change in response to global warming. J. Clim. 25 , 2979–2994 (2012).
Shaw, T. et al. Storm track processes and the opposing influences of climate change. Nat. Geosci. 9 , 656–664 (2016).
Bengtsson, L., Hodges, K. & Roeckner, E. Storm tracks and climate change. J. Clim. 19 , 3518–3543 (2006).
Catto, J. L. et al. The future of midlatitude cyclones. Curr. Clim. Change Rep. 5 , 407–420 (2019).
O’Gorman, P. A. Understanding the varied response of the extratropical storm tracks to climate change. Proc. Natl Acad. Sci. USA 107 , 19176–19180 (2011).
McCabe, G., Clark, M. & Serreze, M. Trends in Northern Hemisphere surface cyclone frequency and intensity. J. Clim. 14 , 2763–2768 (2001).
Pryor, S. C. & Hahmann, A. N. in Oxford Research Encyclopedias: Climate Science (ed. von Storch, H.) (Oxford Univ. Press, 2019).
Jung, C., Taubert, D. & Schindler, D. The temporal variability of global wind energy–Long-term trends and inter-annual variability. Energy Convers. Manag. 188 , 462–472 (2019).
Bett, P. E., Thornton, H. E. & Clark, R. T. Using the Twentieth Century Reanalysis to assess climate variability for the European wind industry. Theor. Appl. Climatol. 127 , 61–80 (2017).
Moemken, J., Reyers, M., Buldmann, B. & Pinto, J. G. Decadal predictability of regional scale wind speed and wind energy potentials over Central Europe. Tellus A 68 , 29199 (2016).
Higuchi, K., Huang, J. & Shabbar, A. A wavelet characterization of the North Atlantic oscillation variation and its relationship to the North Atlantic sea surface temperature. Int. J. Climatol. 19 , 1119–1129 (1999).
Schwing, F. B., Jiang, J. & Mendelssohn, R. Coherency of multi-scale abrupt changes between the NAO, NPI, and PDO. Geophys. Res. Lett. 30 , 1406 (2003).
Schoof, J. T. & Pryor, S. C. Assessing the fidelity of AOGCM-simulated relationships between large-scale modes of climate variability and wind speeds. J. Geophys. Res. 119 , 9719–9734 (2014).
Sandeep, S., Ajayamohan, R., Boos, W. R., Sabin, T. & Praveen, V. Decline and poleward shift in Indian summer monsoon synoptic activity in a warming climate. Proc. Natl Acad. Sci. USA 115 , 2681–2686 (2018).
Vautard, R., Cattiaux, J., Yiou, P., Thepaut, J. N. & Ciais, P. Northern hemisphere atmospheric stilling partly attributed to an increase in surface roughness. Nat. Geosci. 3 , 756–761 (2010).
Pryor, S. C. & Ledolter, J. Addendum to “Wind speed trends over the contiguous United States”. J. Geophys. Res. Atmos. 115 , D10103 (2010).
Pryor, S. C. et al. Wind speed trends over the contiguous United States. J. Geophys. Res. Atmos. 114 , D14105 (2009).
Wu, J., Zha, J. L., Zhao, D. M. & Yang, Q. D. Changes in terrestrial near-surface wind speed and their possible causes: an overview. Clim. Dyn. 51 , 2039–2078 (2018).
Chen, L., Pryor, S. C., Wang, H. & Zhang, R. Distribution and variation of the surface sensible heat flux over the central and eastern Tibetan Plateau: comparison of station observations and multireanalysis products. J. Geophys. Res. Atmos. 124 , 6191–6206 (2019).
Zeng, Z. et al. A reversal in global terrestrial stilling and its implications for wind energy production. Nat. Clim. Change 9 , 979–985 (2019). Reports evidence for the presence of low-frequency variability in near-surface wind speeds .
Poan, E., Gachon, P., Laprise, R., Aider, R. & Dueymes, G. Investigating added value of regional climate modeling in North American winter storm track simulations. Clim. Dyn. 50 , 1799–1818 (2018).
Trzeciak, T. M., Knippertz, P., Pirret, J. S. & Williams, K. D. Can we trust climate models to realistically represent severe European windstorms? Clim. Dyn. 46 , 3431–3451 (2016).
Hodges, K. I., Lee, R. W. & Bengtsson, L. A comparison of extratropical cyclones in recent reanalyses ERA-Interim, NASA MERRA, NCEP CFSR, and JRA-25. J. Clim. 24 , 4888–4906 (2011).
Pryor, S. C., Schoof, J. T. & Barthelmie, R. J. Winds of change?: Projections of near-surface winds under climate change scenarios. Geophys. Res. Lett. 33 , L11702 (2006).
Pryor, S. C. & Barthelmie, R. J. in Climate Change in the Midwest: Impacts, Risks, Vulnerability, and Adaptation Ch. 16 (ed. Pryor, S.C.) 213–229 (Indiana Univ. Press, 2013).
Pryor, S. C. & Barthelmie, R. J. Assessing climate change impacts on the near-term stability of the wind energy resource over the USA. Proc. Natl Acad. Sci. USA 108 , 8167–8171 (2011).
Winterfeldt, J. & Weisse, R. Assessment of value added for surface marine wind speed obtained from two regional climate models. Mon. Weather Rev. 137 , 2955–2965 (2009).
Pryor, S. C., Barthelmie, R. J. & Schoof, J. T. Past and future wind climates over the contiguous USA based on the North American Regional Climate Change Assessment Program model suite. J. Geophys. Res. 117 , D19119 (2012).
Larsen, X. G., Mann, J., Berg, J., Gottel, H. & Jacob, D. Wind climate from the regional climate model REMO. Wind Energy 13 , 279–296 (2010).
Pryor, S. C., Nikulin, G. & Jones, C. Influence of spatial resolution on regional climate model derived wind climates. J. Geophys. Res. Atmos. 117 , D03117 (2012).
Kusiak, A. Share data on wind energy: giving researchers access to information on turbine performance would allow wind farms to be optimized through data mining. Nature 529 , 19–22 (2016).
Holt, E. & Wang, J. Trends in wind speed at wind turbine height of 80 m over the contiguous United States using the North American Regional Reanalysis (NARR). J. Appl. Meteorol. Climatol. 51 , 2188–2202 (2012).
McVicar, T. R. et al. Wind speed climatology and trends for Australia, 1975–2006: Capturing the stilling phenomenon and comparison with near-surface reanalysis output. Geophys. Res. Lett. 35 , L20403 (2008).
Chen, L., Li, D. & Pryor, S. C. Wind speed trends over China: quantifying the magnitude and assessing causality. Int. J. Climatol. 33 , 2579–2590 (2013).
Hueging, H., Haas, R., Born, K., Jacob, D. & Pinto, J. G. Regional changes in wind energy potential over Europe using regional climate model ensemble projections. J. Appl. Meteorol. Climatol. 52 , 903–917 (2013).
Pryor, S. C. et al. Analyses of possible changes in intense and extreme wind speeds over northern Europe under climate change scenarios. Clim. Dyn. 38 , 189–208 (2012).
Reyers, M., Moemken, J. & Pinto, J. G. Future changes of wind energy potentials over Europe in a large CMIP5 multi-model ensemble. Int. J. Climatol. 36 , 783–796 (2016).
Hdidouan, D. & Staffell, I. The impact of climate change on the levelised cost of wind energy. Renew. Energy 101 , 575–592 (2017).
Tobin, I. et al. Assessing climate change impacts on European wind energy from ENSEMBLES high-resolution climate projections. Clim. Change 128 , 99–112 (2015). Includes one of the most comprehensive RCM ensembles and a focus on medium-term projections of wind resources .
Pryor, S. C., Shepherd, T. J., Bukovsky, M. & Barthelmie, R. J. Assessing the stability of wind resource and operating conditions. J. Phys. Conf. Ser. 1452 , 012084 (2020).
Greene, J. S., Chatelain, M., Morrissey, M. & Stadler, S. Projected future wind speed and wind power density trends over the Western US High Plains. Atmos. Clim. Sci. 2 , 32–40 (2012).
Ruffato-Ferreira, V. et al. A foundation for the strategic long-term planning of the renewable energy sector in Brazil: hydroelectricity and wind energy in the face of climate change scenarios. Renew. Sustain. Energy Rev. 72 , 1124–1137 (2017).
de Jong, P. et al. Estimating the impact of climate change on wind and solar energy in Brazil using a South American regional climate model. Renew. Energy 141 , 390–401 (2019).
Kulkarni, S. & Huang, H. P. Changes in surface wind speed over North America from CMIP5 model projections and implications for wind energy. Adv. Meteorol. 2014 , 292768 (2014).
Wiser, R. H. & Bolinger, M. Benchmarking anticipated wind project lifetimes: results from a survey of U.S. Wind industry professionals. Electricity Markets & Policy https://emp.lbl.gov/publications/benchmarking-anticipated-wind-project (2019).
Pryor, S. C. & Barthelmie, R. J. Hybrid downscaling of wind climates over the eastern USA. Environ. Res. Lett. 9 , 024013 (2014).
Pryor, S. C. & Schoof, J. T. Importance of the SRES in projections of climate change impacts on near-surface wind regimes. Meteorol. Z. 19 , 267–274 (2010).
Carvalho, D., Rocha, A., Gomez-Gesteira, M. & Santos, C. S. Potential impacts of climate change on European wind energy resource under the CMIP5 future climate projections. Renew. Energy 101 , 29–40 (2017).
Sakaguchi, K. et al. Exploring a multiresolution approach using AMIP simulations. J. Clim. 28 , 5549–5574 (2015).
Giorgetta, M. A. et al. Climate and carbon cycle changes from 1850 to 2100 in MPI-ESM simulations for the Coupled Model Intercomparison Project phase 5. J. Adv. Model. Earth Syst. 5 , 572–597 (2013).
Johnson, D. L. & Erhardt, R. J. Projected impacts of climate change on wind energy density in the United States. Renew. Energy 85 , 66–73 (2016).
Lindvall, J., Svensson, G. & Caballero, R. The impact of changes in parameterizations of surface drag and vertical diffusion on the large-scale circulation in the Community Atmosphere Model (CAM5). Clim. Dyn. 48 , 3741–3758 (2017).
Larsén, X. G., Ott, S., Badger, J., Hahmann, A. N. & Mann, J. Recipes for correcting the impact of effective mesoscale resolution on the estimation of extreme winds. J. Appl. Meteorol. Climatol. 51 , 521–533 (2012).
Lombardo, F. T. Improved extreme wind speed estimation for wind engineering applications. J. Wind Eng. Ind. Aerodyn. 104–106 , 278–284 (2012).
Cook, N. J. Confidence limits for extreme wind speeds in mixed climates. J. Wind Eng. Ind. Aerodyn. 92 , 41–51 (2004).
Weisse, R. & von Storch, H. in Marine Climate and Climate Change: Storms, Wind Waves and Storm Surges Ch. 5 165–203 (Springer, 2010).
Lombardo, F. T. & Ayyub, B. Approach to estimating near-surface extreme wind speeds with climate change considerations. J. Risk Uncertain. Eng. Syst. A 3 , A4017001 (2017).
Kumar, D., Mishra, V. & Ganguly, A. R. Evaluating wind extremes in CMIP5 climate models. Clim. Dyn. 45 , 441–453 (2015).
Born, K., Ludwig, P. & Pinto, J. G. Wind gust estimation for Mid-European winter storms: towards a probabilistic view. Tellus A 64 , 17471 (2012).
Cheng, C. S., Lopes, E., Fu, C. & Huang, Z. Possible impacts of climate change on wind gusts under downscaled future climate conditions: updated for Canada. J. Clim. 27 , 1255–1270 (2014).
Prein, A. F. et al. A review on regional convection-permitting climate modeling: Demonstrations, prospects, and challenges. Rev. Geophys. 53 , 323–361 (2015). Emphasizes key trends towards higher-fidelity, high-resolution simulations .
Orwig, K. D. & Schroeder, J. L. Near-surface wind characteristics of extreme thunderstorm outflows. J. Wind Eng. Ind. Aerodyn. 95 , 565–584 (2007).
Hoogewind, K. A., Baldwin, M. E. & Trapp, R. J. The impact of climate change on hazardous convective weather in the United States: insight from high-resolution dynamical downscaling. J. Clim. 30 , 10081–10100 (2017).
Di, Y., Lu, J., Xu, X., Feng, T. & Li, L. A response characteristics study of widespread power grid icing to El Nino. Math. Probl. Eng. 2019 , 6589410 (2019).
Yu, Y., Ren, Z., Gao, F. & Meng, X. Changes in surface icing duration over north china during 1961–2015. Atmos. Sci. Lett. 19 , e827 (2018).
Clausen, N. E. et al. in Impacts of Climate Change on Renewable Energy Sources (ed Fenger, J.) 105–128 (Nordic Council of Ministers, 2007).
Zhang, Y., Chen, W. & Riseborough, D. W. Transient projections of permafrost distribution in Canada during the 21st century under scenarios of climate change. Glob. Planet. Change 60 , 443–456 (2008).
Zheng, M., Yang, Z. J., Yang, S. & Still, B. Modeling and mitigation of excessive dynamic responses of wind turbines founded in warm permafrost. Eng. Struct. 148 , 36–46 (2017).
Cheng, G. Permafrost studies in the Qinghai–Tibet Plateau for road construction. J. Cold Reg. Eng. 19 , 19–29 (2005).
Vanem, E. Non-stationary extreme value models to account for trends and shifts in the extreme wave climate due to climate change. Appl. Ocean Res. 52 , 201–211 (2015).
Shope, J. B., Storlazzi, C. D., Erikson, L. H. & Hegermiller, C. A. Changes to extreme wave climates of islands within the Western Tropical Pacific throughout the 21st century under RCP 4.5 and RCP 8.5, with implications for island vulnerability and sustainability. Glob. Planet. Change 141 , 25–38 (2016).
Dvorak, P. A look at repowering older wind farms to 2020. Windpower Engineering & Development https://www.windpowerengineering.com/look-repowering-older-wind-farms-2020/ (2018).
International Energy Agency. Wind technology collaboration programme: annual report 2017 (IEA, 2018).
Pryor, S. C., Barthelme, R. J. & Shepherd, T. 20% of US electricity from wind will have limited impacts on system efficiency and regional climate. Sci. Rep. 10 , 541 (2020).
American Wind Energy Association. Wind industry annual market report, year ending 2017. AWEA https://www.awea.org/resources/publications-and-reports/market-reports/2017-u-s-wind-industry-market-reports (2018).
Dalla Riva, A. et al. IEA wind TCP Task 26 – Wind technology, cost, and performance trends in Denmark, Germany, Ireland, Norway, Sweden, the European Union, and the United States: 2008–2016 (National Renewable Energy Laboratory, 2019).
Wind Europe. Wind energy in Europe in 2018 (Wind Europe, 2019).
National Renewable Energy Laboratory. Annual technology baseline: electricity. NREL https://www.nrel.gov/analysis/data-tech-baseline.html (2019).
Musial, W., Beiter, J., Spitsen, P., Nunemaker, J. & Gevorgian, V. 2018 offshore wind technologies market report (Department of Energy, 2019).
Horn, J. T., Krokstad, J. R. & Leira, B. J. Impact of model uncertainties on the fatigue reliability of offshore wind turbines. Mar. Struct. 64 , 174–185 (2019).
Luengo, J., Negro, V., García-Barba, J., López-Gutiérrez, J.-S. & Esteban, M. D. New detected uncertainties in the design of foundations for offshore wind turbines. Renew. Energy 131 , 667–677 (2019).
Igwemezie, V., Mehmanparast, A. & Kolios, A. Current trend in offshore wind energy sector and material requirements for fatigue resistance improvement in large wind turbine support structures – A review. Renew. Sustain. Energy Rev. 101 , 181–196 (2019).
Dai, K. S. et al. Nonlinear response history analysis and collapse mode study of a wind turbine tower subjected to tropical cyclonic winds. Wind Struct. 25 , 79–100 (2017).
Larsen, X. G. et al. Estimation of offshore extreme wind from wind-wave coupled modeling. Wind Energy 22 , 1043–1057 (2019).
Du, J. T., Bolanos, R. & Larsen, X. G. The use of a wave boundary layer model in SWAN. J. Geophys. Res. Oceans 122 , 42–62 (2017).
Pryor, S. C., Shepherd, T., Volker, P., Hahmann, A. & Barthelmie, R. J. “Wind theft” from onshore wind turbine arrays: Sensitivity to wind farm parameterization and resolution. J. Appl. Meteorol. Climatol. 59 , 153–174 (2020).
Jiménez, P. A., Navarro, J., Palomares, A. M. & Dudhia, J. Mesoscale modeling of offshore wind turbine wakes at the wind farm resolving scale: a composite-based analysis with the Weather Research and Forecasting model over Horns Rev. Wind Energy 18 , 559–566 (2015).
Wilczak, J. et al. The Wind Forecast Improvement Project (WFIP): A public–private partnership addressing wind energy forecast needs. Bull. Am. Meteorol. Soc. 96 , 1699–1718 (2015).
Gutowski, W. J. Jr. et al. The ongoing need for high-resolution regional climate models: Process understanding and stakeholder information. Bull. Am. Meteorol. Soc. 101 , E664–E683 (2020).
Kendon, E. J. et al. Do convection-permitting regional climate models improve projections of future precipitation change? Bull. Am. Meteorol. Soc. 98 , 79–93 (2017).
Bukovsky, M. S., Gochis, D. J. & Mearns, L. O. Towards assessing NARCCAP regional climate model credibility for the North American monsoon: Current climate simulations. J. Clim. 26 , 8802–8826 (2013).
Haarsma, R. J. et al. High resolution model intercomparison project (HighResMIP v1. 0) for CMIP6. Geosci. Model Dev. 9 , 4185–4208 (2016).
Stevens, B. et al. DYAMOND: The DYnamics of the Atmospheric general circulation Modeled On Non-hydrostatic Domains. Prog. Earth Planet. Sci. 6 , 61 (2019).
Hagos, S., Leung, L. R., Zhao, C., Feng, Z. & Sakaguchi, K. How do microphysical processes influence large-scale precipitation variability and extremes? Geophys. Res. Lett. 45 , 1661–1667 (2018).
Yang, Z. et al. Modeling analysis of the swell and wind-sea climate in the Salish Sea. Estuarine Coast. Shelf Sci. 224 , 289–300 (2019).
Coppola, E. et al. A first-of-its-kind multi-model convection permitting ensemble for investigating convective phenomena over Europe and the Mediterranean. Clim. Dyn. 55 , 3–34 (2020).
Roberts, M. et al. The benefits of global high resolution for climate simulation: process understanding and the enabling of stakeholder decisions at the regional scale. Bull. Am. Meteorol. Soc. 99 , 2341–2359 (2018).
von Trentini, F., Leduc, M. & Ludwig, R. Assessing natural variability in RCM signals: comparison of a multi model EURO-CORDEX ensemble with a 50-member single model large ensemble. Clim. Dyn. 53 , 1963–1979 (2019).
Marbaix, P., Gallée, H., Brasseur, O. & van Ypersele, J.-P. Lateral boundary conditions in regional climate models: a detailed study of the relaxation procedure. Mon. Weather Rev. 131 , 461–479 (2003).
Giorgi, F. Thirty years of regional climate modeling: Where are we and where are we going next? J. Geophys. Res. Atmos. 124 , 5696–5723 (2019). Summarizes the history of, challenges to and new prospects for regional climate modelling .
Gao, J. & O’Neill, B. C. Data-driven spatial modeling of global long-term urban land development: The SELECT model. Environ. Model. Softw. 119 , 458–471 (2019).
Hamlington, B. D., Hamlington, P. E., Collins, S. G., Alexander, S. R. & Kim, K. Y. Effects of climate oscillations on wind resource variability in the United States. Geophys. Res. Lett. 42 , 145–152 (2015).
Lledo, L., Bellprat, O., Doblas-Reyes, F. J. & Soret, A. Investigating the effects of Pacific sea surface temperatures on the wind drought of 2015 over the United States. J. Geophys. Res. Atmos. 123 , 4837–4849 (2018).
Greene, J. S., Chatelain, M., Morrissey, M. & Stadler, S. Estimated changes in wind speed and wind power density over the western High Plains, 1971–2000. Theor. Appl. Climatol. 109 , 507–518 (2012).
Klink, K. Atmospheric circulation effects on wind speed variability at turbine height. J. Appl. Meteorol. Climatol. 46 , 445–456 (2007).
Clifton, A. & Lundquist, J. K. Data clustering reveals climate impacts on local wind phenomena. J. Appl. Meteorol. Climatol. 51 , 1547–1557 (2012).
George, S. S. & Wolfe, S. A. El Niño stills winter winds across the southern Canadian Prairies. Geophys. Res. Lett. 36 , L23806 (2009).
Torres Silva dos Santos, A., E Silva, S. & Moisés, C. Seasonality, interannual variability, and linear tendency of wind speeds in the Northeast Brazil from 1986 to 2011. Sci. World J. 2013 , 490857 (2013).
Bianchi, E., Solarte, A. & Guozden, T. M. Large scale climate drivers for wind resource in Southern South America. Renew. Energy 114 , 708–715 (2017).
Watts, D., Duran, P. & Flores, Y. How does El Nino Southern Oscillation impact the wind resource in Chile? A techno-economical assessment of the influence of El Nino and La Nina on the wind power. Renew. Energy 103 , 128–142 (2017).
Ravestein, P., van der Schrier, G., Haarsma, R., Scheele, R. & van den Broek, M. Vulnerability of European intermittent renewable energy supply to climate change and climate variability. Renew. Sustain. Energy Rev. 97 , 497–508 (2018).
Francois, B. Influence of winter North-Atlantic oscillation on climate-related-energy penetration in Europe. Renew. Energy 99 , 602–613 (2016).
Kriesche, P. & Schlosser, C. A. The association of the North Atlantic and the Arctic Oscillation on wind energy resource over Europe and its intermittency. Energy Procedia 52 , 270–277 (2014).
Jerez, S. et al. The impact of the North Atlantic Oscillation on renewable energy resources in southwestern Europe. J. Appl. Meteorol. Climatol. 52 , 2204–2225 (2013).
Brayshaw, D. J., Troccoli, A., Fordham, R. & Methven, J. The impact of large scale atmospheric circulation patterns on wind power generation and its potential predictability: A case study over the UK. Renew. Energy 36 , 2087–2096 (2011).
Earl, N., Dorling, S., Hewston, R. & von Glasow, R. 1980–2010 variability in UK surface wind climate. J. Clim. 26 , 1172–1191 (2013).
Iversen, E. C. & Burningham, H. Relationship between NAO and wind climate over Norway. Clim. Res. 63 , 115–134 (2015).
Commin, A. N. et al. The influence of the North Atlantic Oscillation on diverse renewable generation in Scotland. Appl. Energy 205 , 855–867 (2017).
Albani, A., Ibrahim, M. Z. & Yong, K. H. Influence of the ENSO and monsoonal season on long-term wind energy potential in Malaysia. Energies 11 , 2965 (2018).
Dunning, C., Turner, A. & Brayshaw, D. The impact of monsoon intraseasonal variability on renewable power generation in India. Environ. Res. Lett. 10 , 064002 (2015).
Haupt, S. E. et al. A method to assess the wind and solar resource and to quantify interannual variability over the United States under current and projected future climate. J. Appl. Meteorol. Climatol. 55 , 345–363 (2016).
Rasmussen, D., Holloway, T. & Nemet, G. Opportunities and challenges in assessing climate change impacts on wind energy — a critical comparison of wind speed projections in California. Environ. Res. Lett. 6 , 024008 (2011).
Karnauskas, K. B., Lundquist, J. K. & Zhang, L. Southward shift of the global wind energy resource under high carbon dioxide emissions. Nat. Geosci. 11 , 38–43 (2018).
Daines, J. T., Monahan, A. H. & Curry, C. L. Model-based projections and uncertainties of near-surface wind climate in western Canada. J. Appl. Meteorol. Climatol. 55 , 2229–2245 (2016).
Gross, M. & Magar, V. Offshore wind energy climate projection using UPSCALE climate data under the RCP8.5 emission scenario. PLoS ONE 11 , e0165423 (2016).
Devis, A., Van Lipzig, N. P. M. & Demuzere, M. Should future wind speed changes be taken into account in wind farm development? Environ. Res. Lett. 13 , 064012 (2018).
Reyers, M., Pinto, J. G. & Moemken, J. Statistical-dynamical downscaling for wind energy potentials: evaluation and applications to decadal hindcasts and climate change projections. Int. J. Climatol. 35 , 229–244 (2015).
Davy, R., Gnatiuk, N., Pettersson, L. & Bobylev, L. Climate change impacts on wind energy potential in the European domain with a focus on the Black Sea. Renew. Sustain. Energy Rev. 81 , 1652–1659 (2018).
Chen, L., Pryor, S. C. & Li, D. Assessing the performance of Intergovernmental Panel on Climate Change AR5 climate models in simulating and projecting wind speeds over China. J. Geophys. Res. Atmos. 117 , D24102 (2012).
Xiong, Y. J., Xin, X. G. & Kou, X. X. Simulation and projection of near-surface wind speeds in China by BCC-CSM models. J. Meteorol. Res. 33 , 149–158 (2019).
Evans, J. P., Kay, M., Prasad, A. & Pitman, A. The resilience of Australian wind energy to climate change. Environ. Res. Lett. 13 , 024014 (2018).
Ohba, M. The impact of global warming on wind energy resources and ramp events in Japan. Atmosphere 10 , 265 (2019).
Kamranzad, B. & Mori, N. Future wind and wave climate projections in the Indian Ocean based on a super-high-resolution MRI-AGCM3.2S model projection. Clim. Dyn. 53 , 2391–2410 (2019).
Lawrence, D. M. et al. Parameterization improvements and functional and structural advances in version 4 of the Community Land Model. J. Adv. Model. Earth Syst. 3 , M03001 (2011).
Bogenschutz, P. A. et al. The path to CAM6: coupled simulations with CAM5.4 and CAM5.5. Geosci. Model. Dev. 11 , 235–255 (2018).
Zhao, C. et al. Exploring the impacts of physics and resolution on aqua-planet simulations from a nonhydrostatic global variable-resolution modeling framework. J. Adv. Model. Earth Syst. 8 , 1751–1768 (2016).
Wilks, D. S. Statistical Methods in the Atmospheric Sciences Vol. 100 (Academic, 2011).
Download references
Acknowledgements
This work was supported by the U.S. Department of Energy (DoE) (DE-SC0016438 and DE-SC0016605). The research used computing resources from the NCAR-CISL programme (UCOR0020) and the National Science Foundation’s Extreme Science and Engineering Discovery Environment (XSEDE) (allocation award to S.C.P. is TG-ATM170024). The authors express their appreciation to N. Davis of DTU Wind Energy for providing access to a digital form of the Global Wind Atlas mean wind speeds shown in Fig. 1a.
Author information
Authors and affiliations.
Department of Earth and Atmospheric Sciences, Cornell University, Ithaca, NY, USA
Sara C. Pryor
Sibley School of Mechanical and Aerospace Engineering, Cornell University, Ithaca, NY, USA
Rebecca J. Barthelmie
National Center for Atmospheric Research, Boulder, CO, USA
Melissa S. Bukovsky
Earth Systems Analysis & Modeling, Pacific Northwest National Laboratory, Richland, WA, USA
L. Ruby Leung & Koichi Sakaguchi
You can also search for this author in PubMed Google Scholar
Contributions
S.C.P. conducted the majority of the analyses presented herein, made Figs 1,3 and Tables 1,2, undertook the literature review and wrote the initial draft of the manuscript. R.J.B. conducted the analyses presented in Fig. 2 and wrote related text and wrote the initial draft of the wind energy industry trends. M.S.B. performed the WRF simulations that are analysed and presented herein and wrote part of the section on reconciling climate and wind energy science. L.R.L. helped perform the simulations presented in Fig. 4 and contributed related text. K.S. performed the MPAS simulations that are analysed and presented herein and wrote parts of the section on reconciling climate and wind energy science. All co-authors contributed to editing the draft manuscript and read and approved the revised manuscript.
Corresponding author
Correspondence to Sara C. Pryor .
Ethics declarations
Competing interests.
The authors declare no competing interests.
Additional information
Peer review information.
Nature Reviews Earth & Environment thanks Christopher Jung and the other, anonymous, reviewer(s) for their contribution to the peer review of this work.

Publisher’s note
Springer Nature remains neutral with regard to jurisdictional claims in published maps and institutional affiliations.
Rights and permissions
Reprints and permissions
About this article
Cite this article.
Pryor, S.C., Barthelmie, R.J., Bukovsky, M.S. et al. Climate change impacts on wind power generation. Nat Rev Earth Environ 1 , 627–643 (2020). https://doi.org/10.1038/s43017-020-0101-7
Download citation
Accepted : 27 August 2020
Published : 20 October 2020
Issue Date : December 2020
DOI : https://doi.org/10.1038/s43017-020-0101-7
Share this article
Anyone you share the following link with will be able to read this content:
Sorry, a shareable link is not currently available for this article.
Provided by the Springer Nature SharedIt content-sharing initiative
Quick links
- Explore articles by subject
- Guide to authors
- Editorial policies
Sign up for the Nature Briefing newsletter — what matters in science, free to your inbox daily.

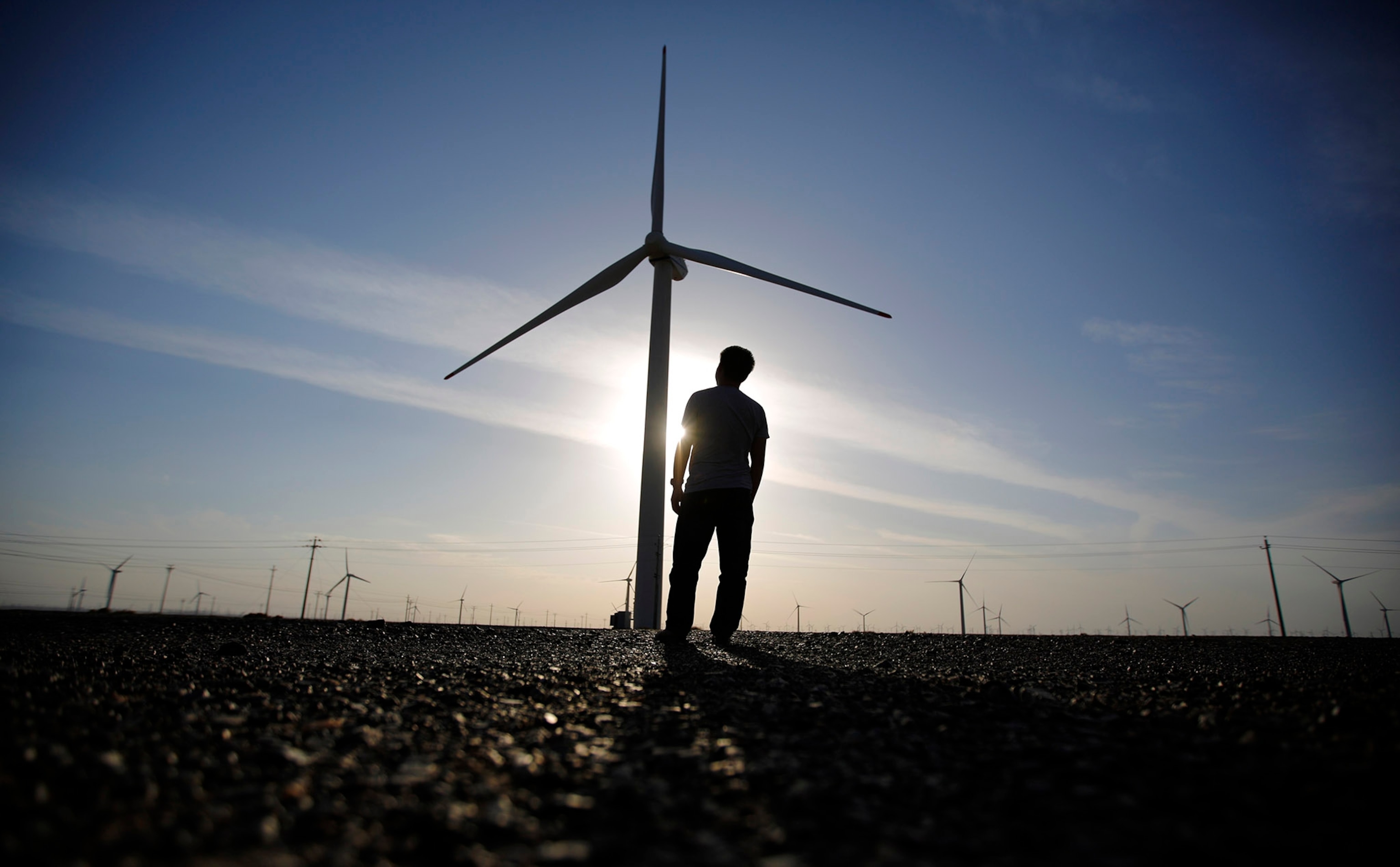
A worker looks at a wind turbine used to generate electricity, at a wind farm in Guazhou, China. China is the world's biggest producer of CO2 emissions, but is also the world's leading generator of renewable electricity.
Wind power offers a sustainable option in the pursuit of renewable energy.
Wind is the movement of air from an area of high pressure to an area of low pressure. In fact, wind exists because the sun unevenly heats the surface of the Earth. As hot air rises, cooler air moves in to fill the void. As long as the sun shines, the wind will blow. And wind has long served as a power source to humans.
Ancient mariners used sails to capture the wind. Farmers once used windmills to grind their grains and pump water. Today, more and more wind turbines wring electricity from the breeze. Over the past decade, wind turbine use has increased more than 25 percent per year. Still, it only provides a small fraction of the world's energy.
How it Works
Most wind energy comes from turbines that can be as tall as a 20-story building and have three 200-foot (60-meter)-long blades. The wind spins the blades, which turn a shaft connected to a generator that produces electricity.
The biggest wind turbines generate enough electricity in a year (about 12 megawatt-hours) to supply about 600 U.S. homes. Wind farms have tens and sometimes hundreds of these turbines lined up together in particularly windy spots. Smaller turbines erected in a backyard can produce enough electricity for a single home or small business.
The Booming Wind Energy Industry
Wind is a clean source of renewable energy that produces no air or water pollution. And since the wind is free, operational costs are nearly zero once a turbine is erected. Mass production and technology advances are making turbines cheaper, and many governments offer tax incentives to spur wind-energy development.
Drawbacks include complaints from locals that wind turbines are ugly and noisy. The slowly rotating blades can also kill birds and bats, but not nearly as many as cars, power lines, and high-rise buildings do. The wind is also variable: If it's not blowing, there's no electricity generated.
Nevertheless, the wind energy industry is booming. Thanks to global efforts to combat climate change, such as the Paris Agreement, renewable energy is seeing a boom in growth, with wind energy leading the way. From 2000 to 2015 , cumulative wind capacity around the world increased from 17,000 megawatts to more than 430,000 megawatts. In 2015, China also surpassed the EU in the number of installed wind turbines and continues to lead installation efforts.
Industry experts predict that if this pace of growth continues, by 2050 one third of the world's electricity needs will be fulfilled by wind power.
Related Topics
- ENVIRONMENT AND CONSERVATION
You May Also Like
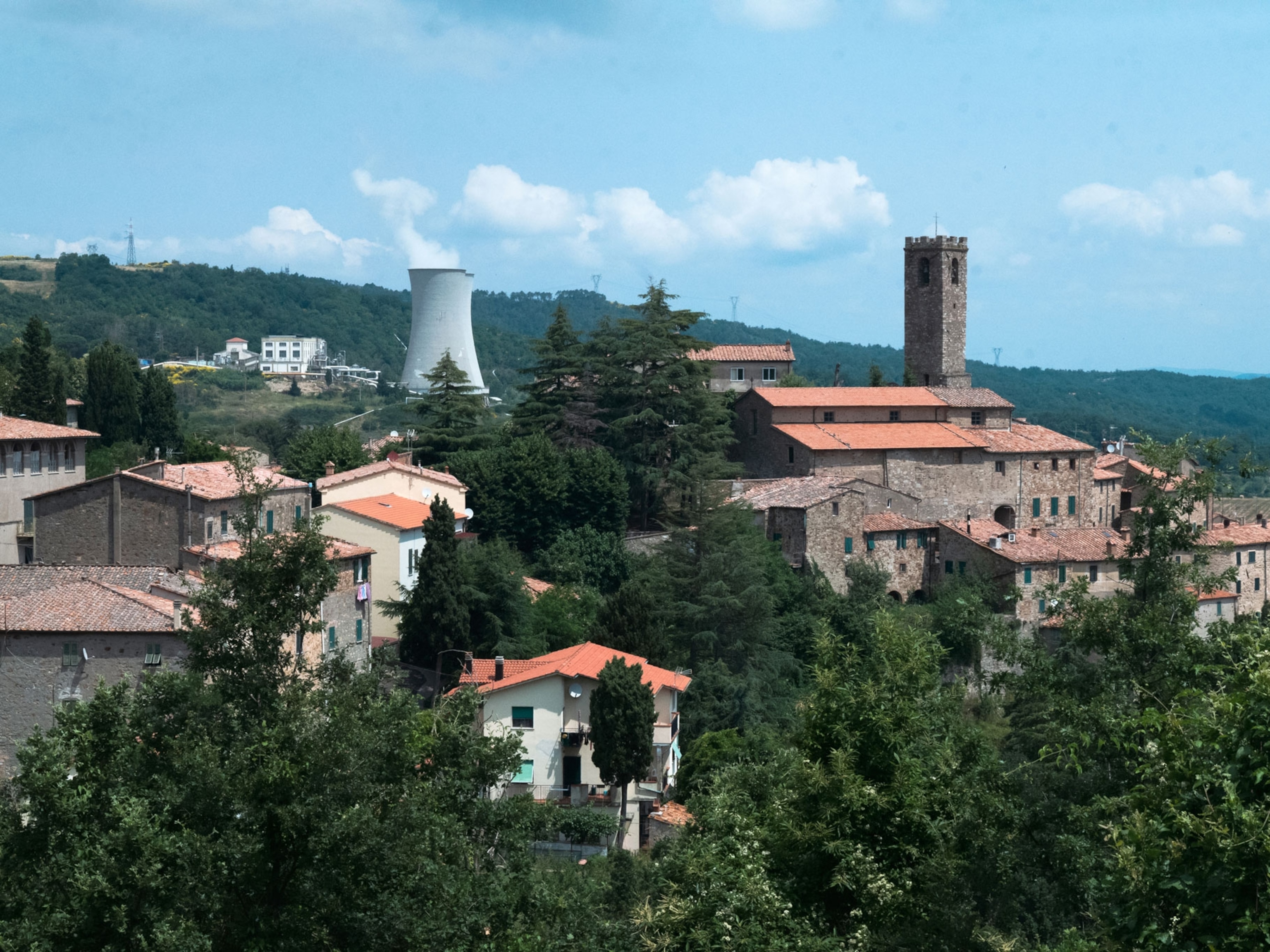
Can energy harnessed from Earth’s interior help power the world?
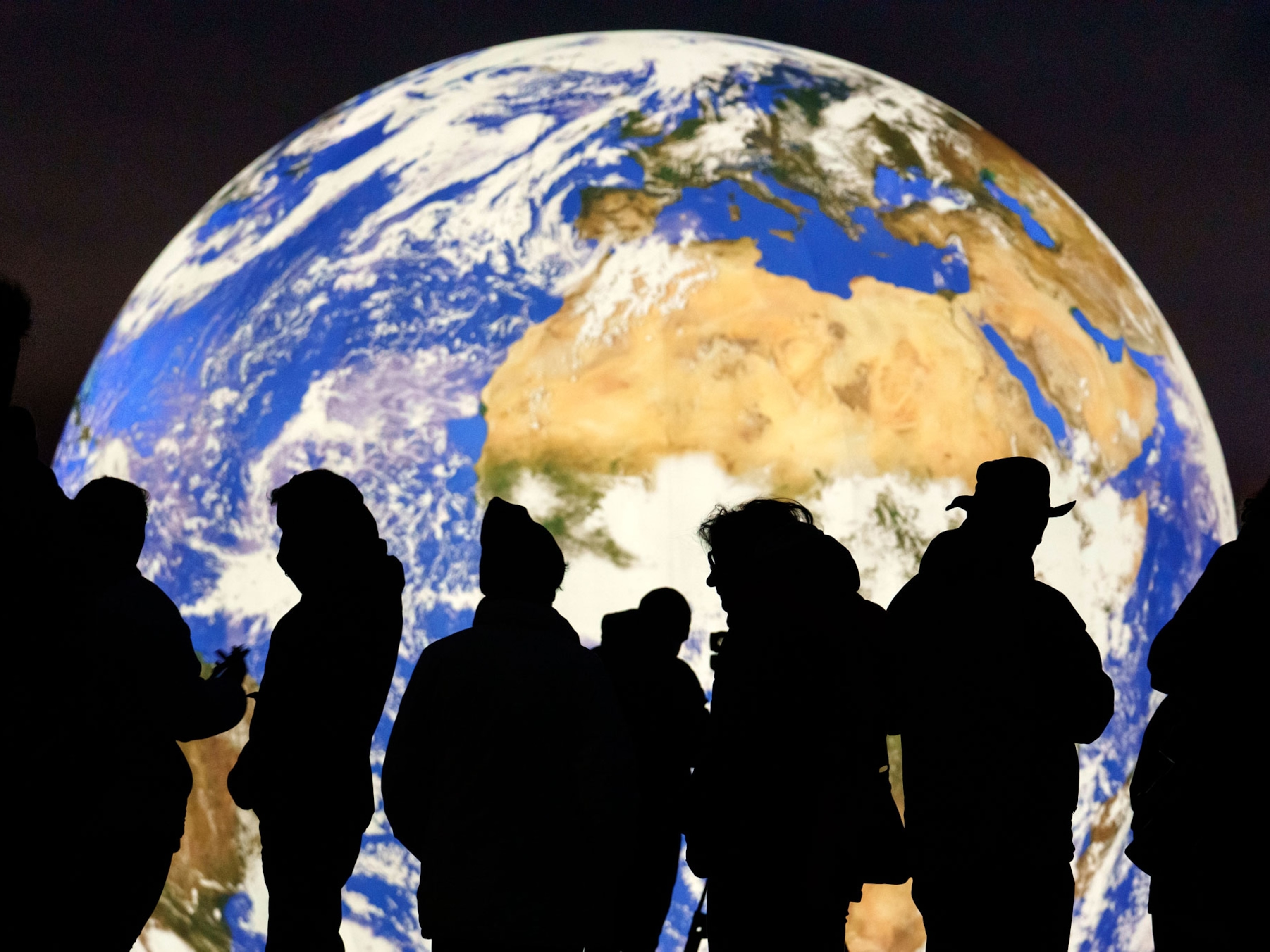
How global warming is disrupting life on Earth
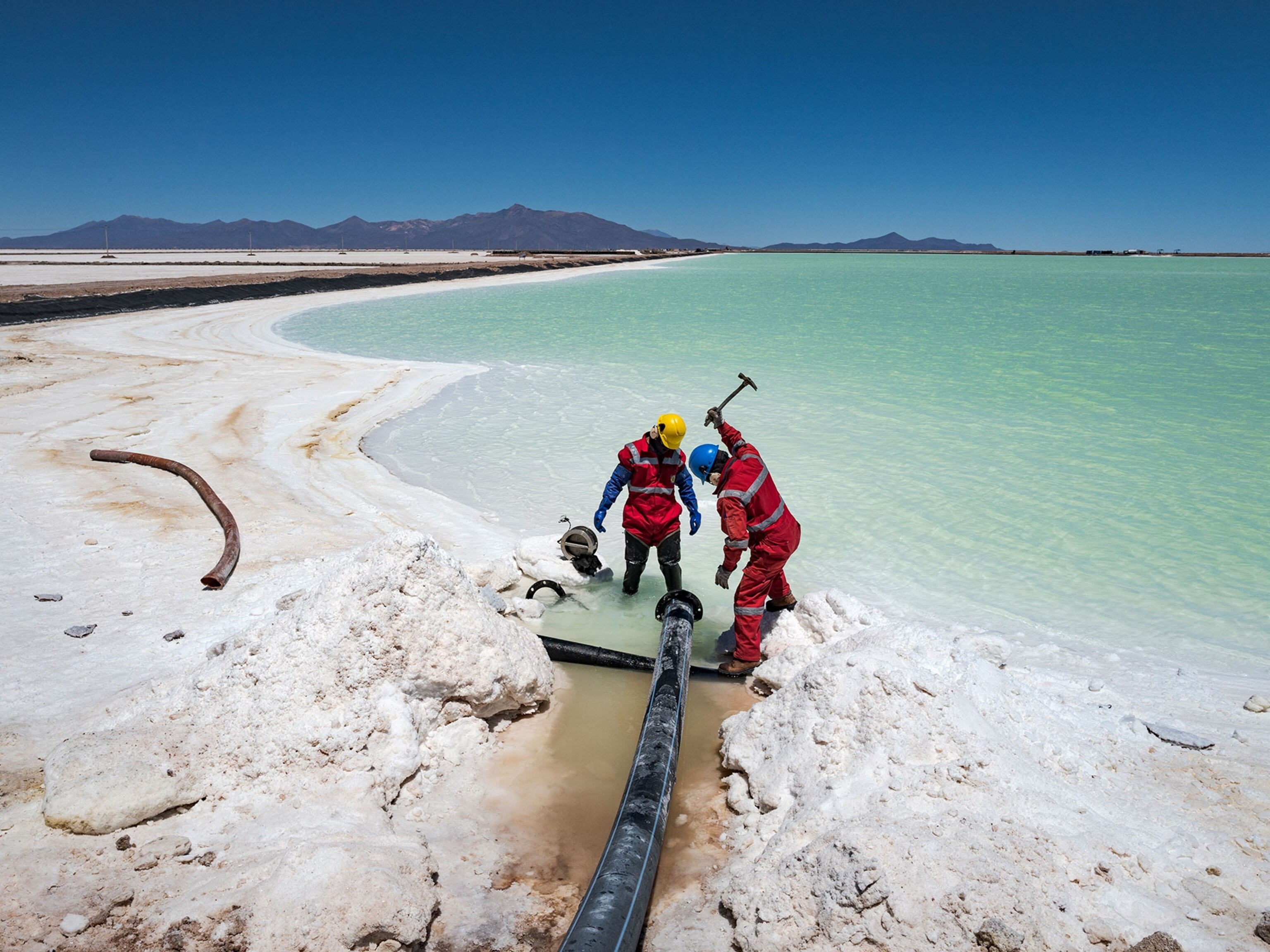
Lithium batteries power your world. How much do you really know about them?
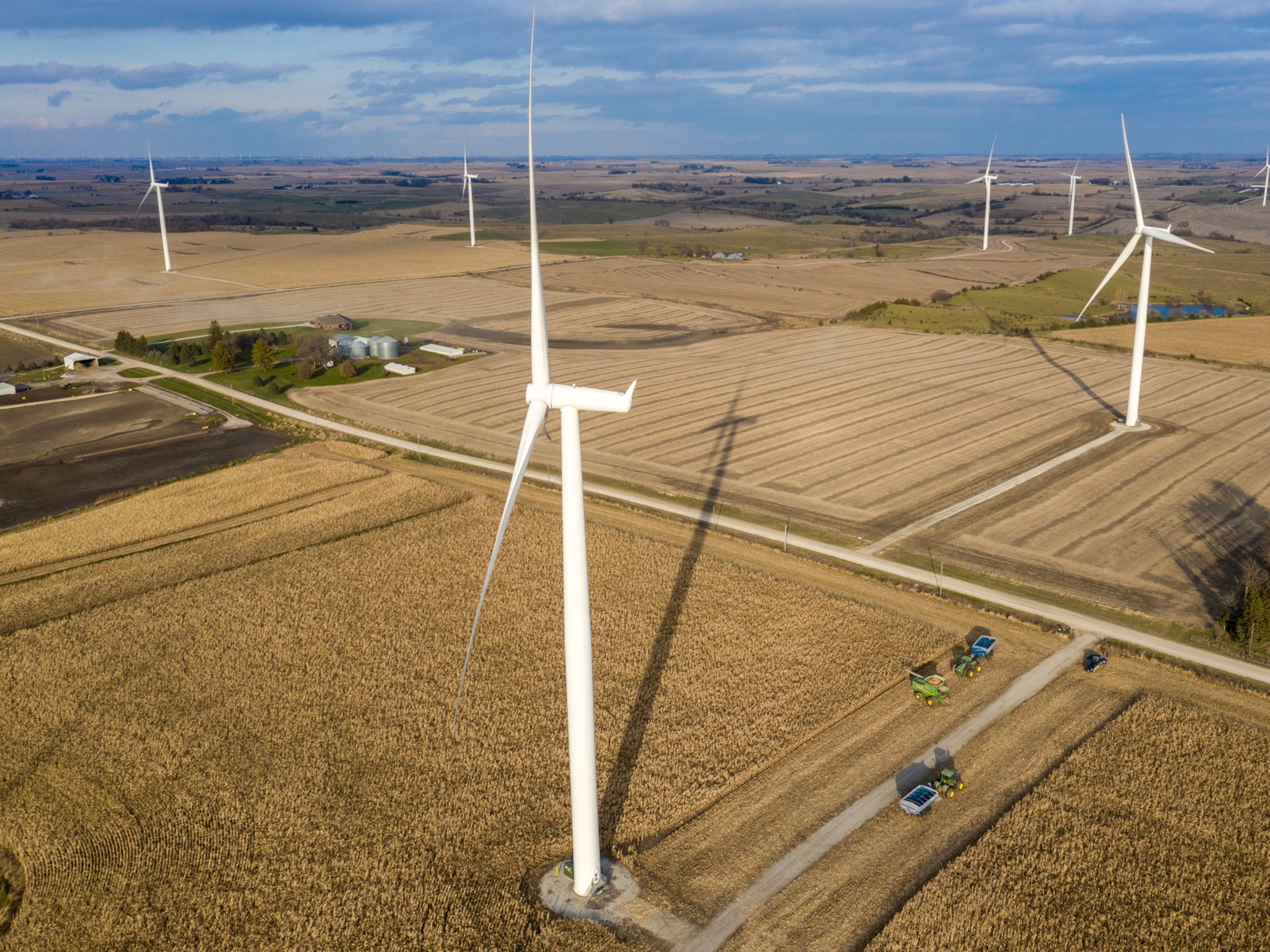
How the historic climate bill will dramatically reduce U.S. emissions
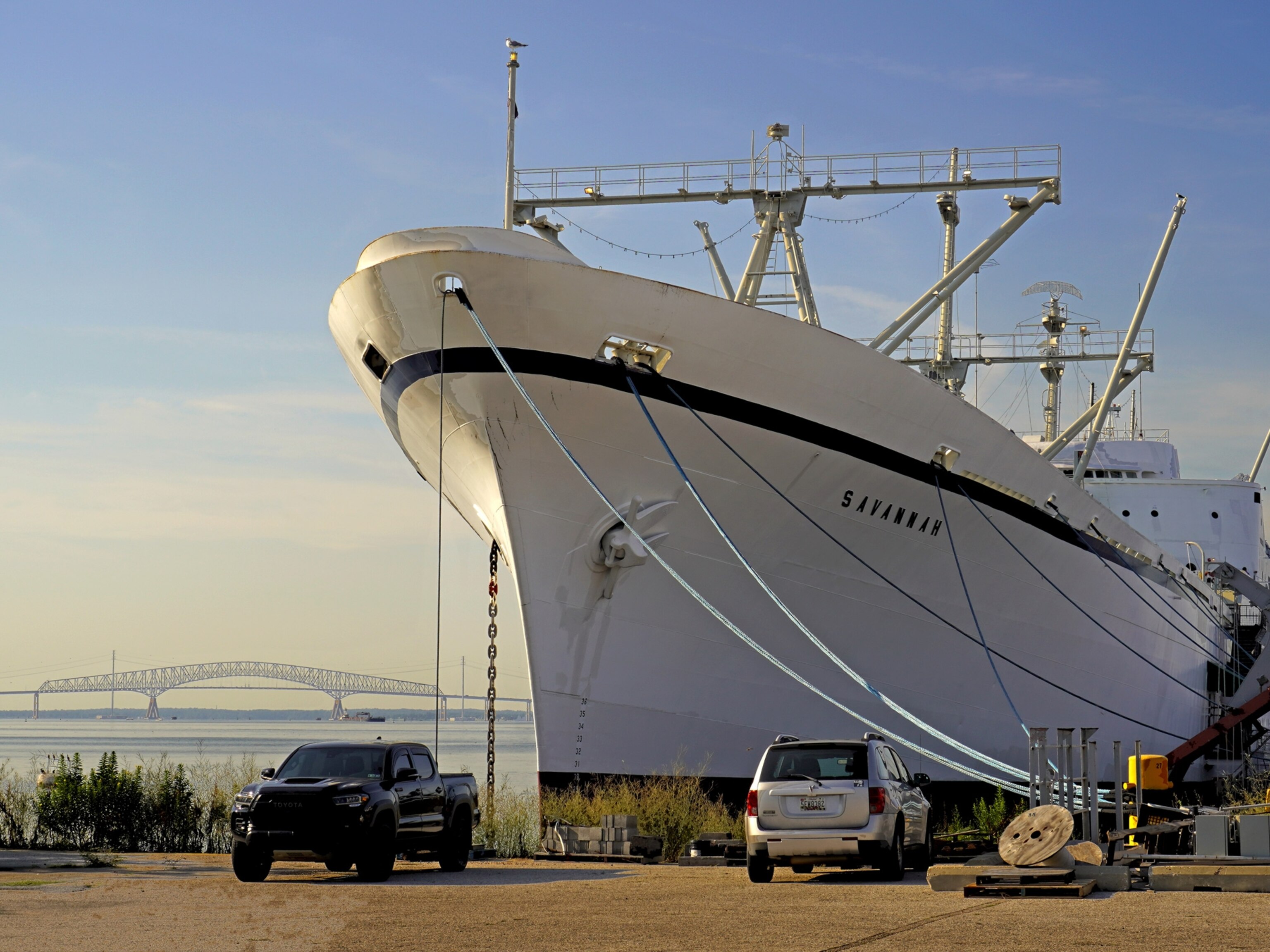
This ship was supposed to usher in an age of nuclear-powered travel
- Environment
- Paid Content
History & Culture
- History & Culture
- Terms of Use
- Privacy Policy
- Your US State Privacy Rights
- Children's Online Privacy Policy
- Interest-Based Ads
- About Nielsen Measurement
- Do Not Sell or Share My Personal Information
- Nat Geo Home
- Attend a Live Event
- Book a Trip
- Inspire Your Kids
- Shop Nat Geo
- Visit the D.C. Museum
- Learn About Our Impact
- Support Our Mission
- Advertise With Us
- Customer Service
- Renew Subscription
- Manage Your Subscription
- Work at Nat Geo
- Sign Up for Our Newsletters
- Contribute to Protect the Planet
Copyright © 1996-2015 National Geographic Society Copyright © 2015-2024 National Geographic Partners, LLC. All rights reserved
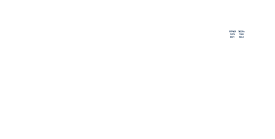
The world’s energy problem
The world faces two energy problems: most of our energy still produces greenhouse gas emissions, and hundreds of millions lack access to energy..
The world lacks safe, low-carbon, and cheap large-scale energy alternatives to fossil fuels. Until we scale up those alternatives the world will continue to face the two energy problems of today. The energy problem that receives most attention is the link between energy access and greenhouse gas emissions. But the world has another global energy problem that is just as big: hundreds of millions of people lack access to sufficient energy entirely, with terrible consequences to themselves and the environment.
The problem that dominates the public discussion on energy is climate change. A climate crisis endangers the natural environment around us, our wellbeing today and the wellbeing of those who come after us.
It is the production of energy that is responsible for 87% of global greenhouse gas emissions and as the chart below shows, people in the richest countries have the very highest emissions.
This chart here will guide us through the discussion of the world's energy problem. It shows the per capita CO2 emissions on the vertical axis against the average income in that country on the horizontal axis.
In countries where people have an average income between $15,000 and $20,000, per capita CO 2 emissions are close to the global average ( 4.8 tonnes CO 2 per year). In every country where people's average income is above $25,000 the average emissions per capita are higher than the global average.
The world’s CO 2 emissions have been rising quickly and reached 36.6 billion tonnes in 2018 . As long as we are emitting greenhouse gases their concentration in the atmosphere increases . To bring climate change to an end the concentration of greenhouse gases in the atmosphere needs to stabilize and to achieve this the world’s greenhouse gas emissions have to decline towards net-zero.
To bring emissions down towards net-zero will be one of the world’s biggest challenges in the years ahead. But the world’s energy problem is actually even larger than that, because the world has not one, but two energy problems.
The twin problems of global energy
The first energy problem: those that have low carbon emissions lack access to energy.
The first global energy problem relates to the left-hand side of the scatter-plot above.
People in very poor countries have very low emissions. On average, people in the US emit more carbon dioxide in 4 days than people in poor countries – such as Ethiopia, Uganda, or Malawi – emit in an entire year. 1
The reason that the emissions of the poor are low is that they lack access to modern energy and technology. The energy problem of the poorer half of the world is energy poverty . The two charts below show that large shares of people in countries with a GDP per capita of less than $25,000 do not have access to electricity and clean cooking fuels. 2
The lack of access to these technologies causes some of the worst global problems of our time.
When people lack access to modern energy sources for cooking and heating, they rely on solid fuel sources – mostly firewood, but also dung and crop waste. This comes at a massive cost to the health of people in energy poverty: indoor air pollution , which the WHO calls "the world's largest single environmental health risk." 3 For the poorest people in the world it is the largest risk factor for early death and global health research suggests that indoor air pollution is responsible for 1.6 million deaths each year, twice the death count of poor sanitation. 4
The use of wood as a source of energy also has a negative impact on the environment around us. The reliance on fuelwood is the reason why poverty is linked to deforestation. The FAO reports that on the African continent the reliance on wood as fuel is the single most important driver of forest degradation. 5 Across East, Central, and West Africa fuelwood provides more than half of the total energy. 6
Lastly, the lack of access to energy subjects people to a life in poverty. No electricity means no refrigeration of food; no washing machine or dishwasher; and no light at night. You might have seen the photos of children sitting under a street lamp at night to do their homework. 7
The first energy problem of the world is the problem of energy poverty – those that do not have sufficient access to modern energy sources suffer poor living conditions as a result.
The second energy problem: those that have access to energy produce greenhouse gas emissions that are too high
The second energy problem is the one that is more well known, and relates to the right hand-side of the scatterplot above: greenhouse gas emissions are too high.
Those that need to reduce emissions the most are the extremely rich. Diana Ivanova and Richard Wood (2020) have just shown that the richest 1% in the EU emit on average 43 tonnes of CO 2 annually – 9-times as much as the global average of 4.8 tonnes. 8
The focus on the rich, however, can give the impression that it is only the emissions of the extremely rich that are the problem. What isn’t made clear enough in the public debate is that for the world's energy supply to be sustainable the greenhouse gas emissions of the majority of the world population are currently too high. The problem is larger for the extremely rich, but it isn’t limited to them.
The Paris Agreement's goal is to keep the increase of the global average temperature to well below 2°C above pre-industrial levels and “to pursue efforts to limit the temperature increase to 1.5°C”. 9
To achieve this goal emissions have to decline to net-zero within the coming decades.
Within richer countries, where few are suffering from energy poverty, even the emissions of the very poorest people are far higher. The paper by Ivanova and Wood shows that in countries like Germany, Ireland, and Greece more than 99% of households have per capita emissions of more than 2.4 tonnes per year.
The only countries that have emissions that are close to zero are those where the majority suffers from energy poverty. 10 The countries that are closest are the very poorest countries in Africa : Malawi, Burundi, and the Democratic Republic of Congo.
But this comes at a large cost to themselves as this chart shows. In no poor country do people have living standards that are comparable to those of people in richer countries.
And since living conditions are better where GDP per capita is higher, it is also the case that CO 2 emissions are higher where living conditions are better. Emissions are high where child mortality is the lowest , where children have good access to education, and where few of them suffer from hunger .
The reason for this is that as soon as people get access to energy from fossil fuels their emissions are too high to be sustainable over the long run (see here ).
People need access to energy for a good life. But in a world where fossil fuels are the dominant source of energy, access to modern energy means that carbon emissions are too high.
The more accurate description of the second global energy problem is therefore: the majority of the world population – all those who are not very poor – have greenhouse gas emissions that are far too high to be sustainable over the long run.
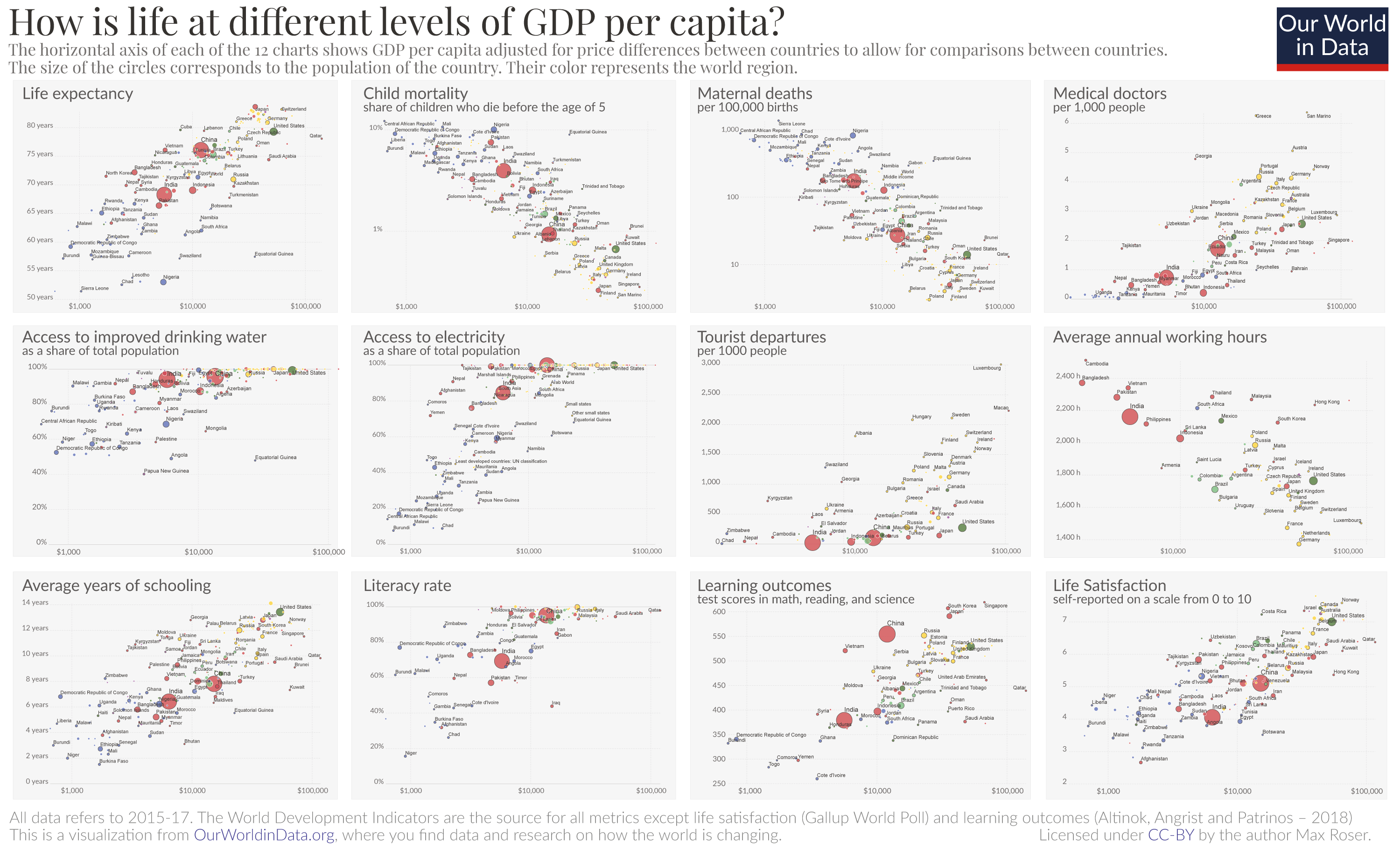
The current alternatives are energy poverty or fossil-fuels and greenhouse gases
The chart here is a version of the scatter plot above and summarizes the two global energy problems: In purple are those that live in energy poverty, in blue those whose greenhouse gas emissions are too high if we want to avoid severe climate change.
So far I have looked at the global energy problem in a static way, but the world is changing of course.
For millennia all of our ancestors lived in the pink bubble: the reliance on wood meant they suffered from indoor air pollution; the necessity of acquiring fuelwood and agricultural land meant deforestation; and minimal technology meant that our ancestors lived in conditions of extreme poverty.
In the last two centuries more and more people have moved from the purple to the blue area in the chart. In many ways this is a very positive development. Economic growth and increased access to modern energy improved people's living conditions. In rich countries almost no one dies from indoor air pollution and living conditions are much better in many ways as we've seen above. It also meant that we made progress against the ecological downside of energy poverty: The link between poverty and the reliance on fuelwood is one of the key reasons why deforestation declines with economic growth. 11 And progress in that direction has been fast: on any average day in the last decade 315,000 people in the world got access to electricity for the first time in their life.
But while living conditions improved, greenhouse gas emissions increased.
The chart shows what this meant for greenhouse gas emissions over the last generation. The chart is a version of the scatter plot above, but it shows the change over time – from 1990 to the latest available data.
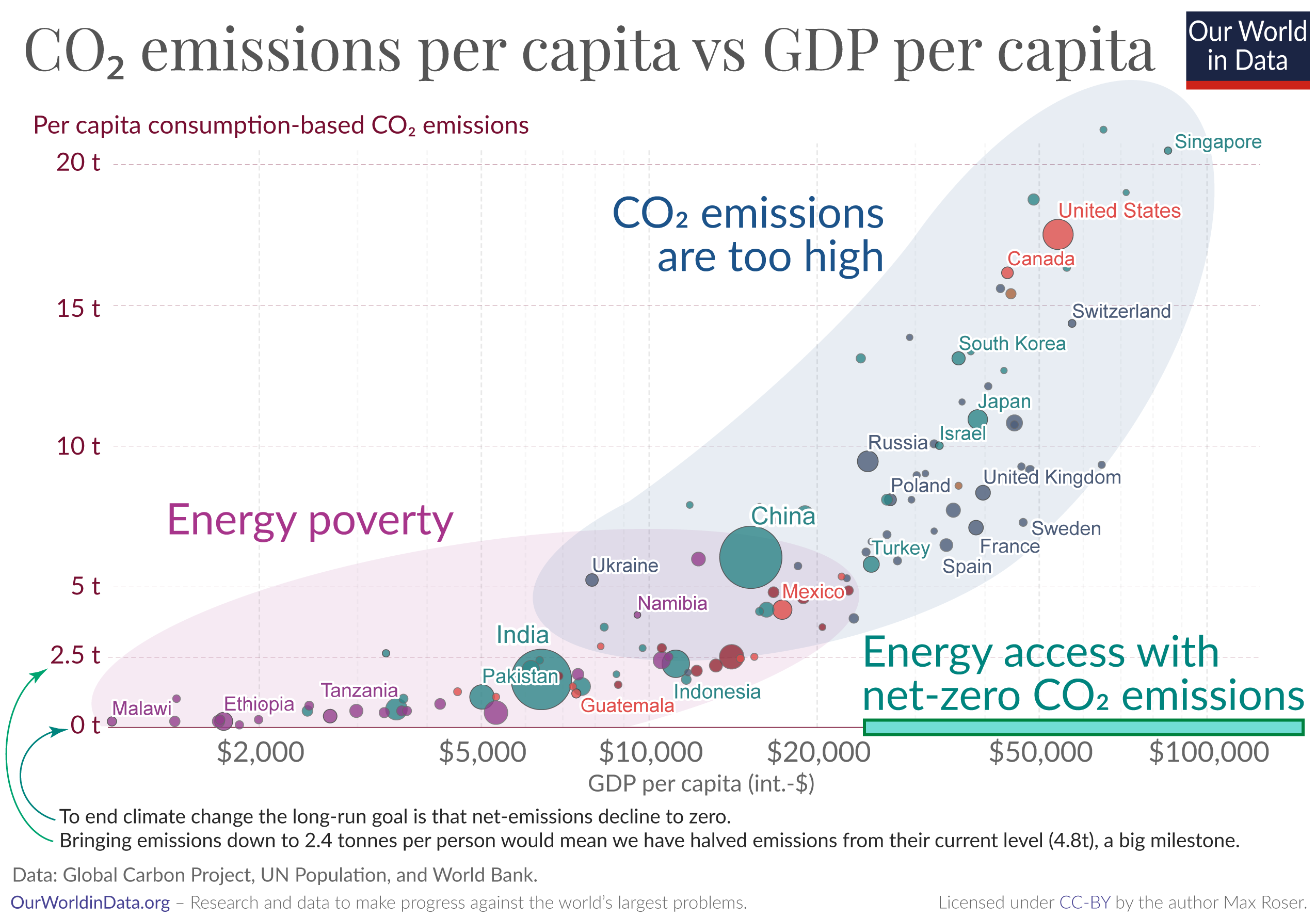
The data is now also plotted on log-log scales which has the advantage that you can see the rates of change easily. On a logarithmic axis the steepness of the line corresponds to the rate of change. What the chart shows is that low- and middle-income countries increased their emissions at very similar rates.
By default the chart shows the change of income and emission for the 14 countries that are home to more than 100 million people, but you can add other countries to the chart.
What has been true in the past two decades will be true in the future. For the poorer three-quarters of the world income growth means catching up with the good living conditions of the richer world, but unless there are cheap alternatives to fossil fuels it also means catching up with the high emissions of the richer world.
Our challenge: find large-scale energy alternatives to fossil fuels that are affordable, safe and sustainable
The task for our generation is therefore twofold: since the majority of the world still lives in poor conditions, we have to continue to make progress in our fight against energy poverty. But success in this fight will only translate into good living conditions for today’s young generation when we can reduce greenhouse gas emissions at the same time.
Key to making progress on both of these fronts is the source of energy and its price . Those living in energy poverty cannot afford sufficient energy and those that left the worst poverty behind rely on fossil fuels to meet their energy needs.
Once we look at it this way it becomes clear that the twin energy problems are really the two sides of one big problem. We lack large-scale energy alternatives to fossil fuels that are cheap, safe, and sustainable.
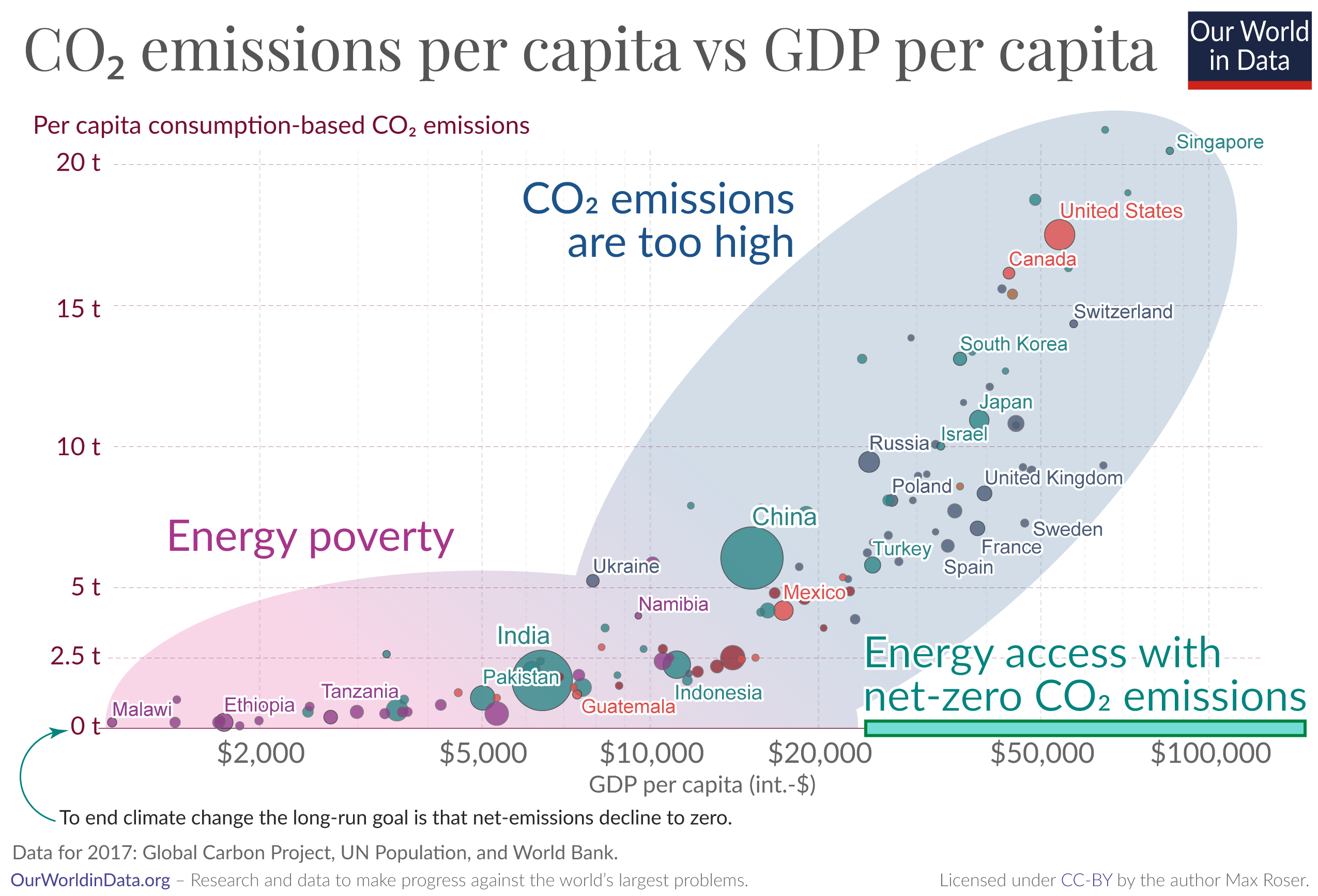
This last version of the scatter plot shows what it would mean to have such energy sources at scale. It would allow the world to leave the unsustainable current alternatives behind and make the transition to the bottom right corner of the chart: the area marked with the green rectangle where emissions are net-zero and everyone has left energy poverty behind.
Without these technologies we are trapped in a world where we have only bad alternatives: Low-income countries that fail to meet the needs of the current generation; high-income countries that compromise the ability of future generations to meet their needs; and middle-income countries that fail on both counts.
Since we have not developed all the technologies that are required to make this transition possible large scale innovation is required for the world to make this transition. This is the case for most sectors that cause carbon emissions , in particular in the transport (shipping, aviation, road transport) and heating sectors, but also cement production and agriculture.
One sector where we have developed several alternatives to fossil fuels is electricity. Nuclear power and renewables emit far less carbon (and are much safer) than fossil fuels. Still, as the last chart shows, their share in global electricity production hasn't changed much: only increasing from 36% to 38% in the last three decades.
But it is possible to do better. Some countries have scaled up nuclear power and renewables and are doing much better than the global average. You can see this if you change the chart to show the data for France and Sweden – in France 92% of electricity comes from low carbon sources, in Sweden it is 99%. The consequence of countries doing better in this respect should be that they are closer to the sustainable energy world of the future. The scatter plot above shows that this is the case.
But for the global energy supply – especially outside the electricity sector – the world is still far away from a solution to the world's energy problem.
Every country is still very far away from providing clean, safe, and affordable energy at a massive scale and unless we make rapid progress in developing these technologies we will remain stuck in the two unsustainable alternatives of today: energy poverty or greenhouse gas emissions.
As can be seen from the chart, the ratio of emissions is 17.49t / 0.2t = 87.45. And 365 days/87.45=4.17 days
It is worth looking into the cutoffs for what it means – according to these international statistics – to have access to energy. The cutoffs are low.
See Raising Global Energy Ambitions: The 1,000 kWh Modern Energy Minimum and IEA (2020) – Defining energy access: 2020 methodology, IEA, Paris.
WHO (2014) – Frequently Asked Questions – Ambient and Household Air Pollution and Health . Update 2014
While it is certain that the death toll of indoor air pollution is high, there are widely differing estimates. At the higher end of the spectrum, the WHO estimates a death count of more than twice that. We discuss it in our entry on indoor air pollution .
The 2018 estimate for premature deaths due to poor sanitation is from the same analysis, the Global Burden of Disease study. See here .
FAO and UNEP. 2020. The State of the World’s Forests 2020. Forests, biodiversity and people. Rome. https://doi.org/10.4060/ca8642en
The same report also reports that an estimated 880 million people worldwide are collecting fuelwood or producing charcoal with it.
This is according to the IEA's World Energy Balances 2020. Here is a visualization of the data.
The second largest energy source across the three regions is oil and the third is gas.
The photo shows students study under the streetlights at Conakry airport in Guinea. It was taken by Rebecca Blackwell for the Associated Press.
It was published by the New York Times here .
The global average is 4.8 tonnes per capita . The richest 1% of individuals in the EU emit 43 tonnes per capita – according to Ivanova D, Wood R (2020). The unequal distribution of household carbon footprints in Europe and its link to sustainability. Global Sustainability 3, e18, 1–12. https://doi.org/10.1017/sus.2020.12
On Our World in Data my colleague Hannah Ritchie has looked into a related question and also found that the highest emissions are concentrated among a relatively small share of the global population: High-income countries are home to only 16% of the world population, yet they are responsible for almost half (46%) of the world’s emissions.
Article 2 of the Paris Agreement states the goal in section 1a: “Holding the increase in the global average temperature to well below 2 °C above pre-industrial levels and to pursue efforts to limit the temperature increase to 1.5 °C above pre-industrial levels, recognizing that this would significantly reduce the risks and impacts of climate change.”
It is an interesting question whether there are some subnational regions in richer countries where a larger group of people has extremely low emissions; it might possibly be the case in regions that rely on nuclear energy or renewables (likely hydro power) or where aforestation is happening rapidly.
Crespo Cuaresma, J., Danylo, O., Fritz, S. et al. Economic Development and Forest Cover: Evidence from Satellite Data. Sci Rep 7, 40678 (2017). https://doi.org/10.1038/srep40678
Bruce N, Rehfuess E, Mehta S, et al. Indoor Air Pollution. In: Jamison DT, Breman JG, Measham AR, et al., editors. Disease Control Priorities in Developing Countries. 2nd edition. Washington (DC): The International Bank for Reconstruction and Development / The World Bank; 2006. Chapter 42. Available from: https://www.ncbi.nlm.nih.gov/books/NBK11760/ Co-published by Oxford University Press, New York.
Cite this work
Our articles and data visualizations rely on work from many different people and organizations. When citing this article, please also cite the underlying data sources. This article can be cited as:
BibTeX citation
Reuse this work freely
All visualizations, data, and code produced by Our World in Data are completely open access under the Creative Commons BY license . You have the permission to use, distribute, and reproduce these in any medium, provided the source and authors are credited.
The data produced by third parties and made available by Our World in Data is subject to the license terms from the original third-party authors. We will always indicate the original source of the data in our documentation, so you should always check the license of any such third-party data before use and redistribution.
All of our charts can be embedded in any site.
Our World in Data is free and accessible for everyone.
Help us do this work by making a donation.

An official website of the United States government
The .gov means it’s official. Federal government websites often end in .gov or .mil. Before sharing sensitive information, make sure you’re on a federal government site.
The site is secure. The https:// ensures that you are connecting to the official website and that any information you provide is encrypted and transmitted securely.
- Publications
- Account settings
Preview improvements coming to the PMC website in October 2024. Learn More or Try it out now .
- Advanced Search
- Journal List
- Springer Nature - PMC COVID-19 Collection

Wind energy and sustainable electricity generation: evidence from Germany
Sakiru adebola solarin.
1 School of Economics, University of Nottingham Malaysia Jalan Broga, 43500 Semenyih, Malaysia
Mufutau Opeyemi Bello
2 Faculty of Social Sciences, Department of Economics, University of Ilorin, PMB 1515, Ilorin, Nigeria
Wind energy is one of the renewable energy sources that has been touted to address the challenges of energy security and environmental degradation. This is only attainable if countries with substantial wind energy potential use it in significant proportion to satisfy their energy needs. One promising sector where wind energy can be employed to actualize this potential is the electricity sector. However, the current reality is that fossil fuels still dominate the energy profiles of most economies of the world, including the advanced economies, with wind renewable energy source accounting for a very small proportion of the energy mix. Germany is one of the few countries that offers promising opportunities in deploying wind energy to its full potentials. This study therefore explores the feasibility of substituting wind energy for nuclear energy and other fossil fuels using Germany as a country of focus. We use the ridge regression procedure to analyse yearly time series data for the German power sector that spans the period 1986 to 2018. With respect to output elasticities of the energy inputs, the results reveal that wind and natural gas have positive output elasticity estimates while the estimates for nuclear and coal are negative. We also found that all the inputs pairs have positive substitution elasticity estimates between them. With respect to wind energy, the highest substitutability estimate occurred with nuclear power which is followed by natural gas and then coal. The study recommended that policies such as granting of tax credit for wind energy technology, reduction in property taxes for wind power facilities, and allocation of fund for research and development (R&D) in wind energy technology are recommended to promote the use of wind energy in the economy.
Introduction
The growing importance of renewable energy, especially among the developed countries, is expected to lead to reduction in fossil fuels in the energy mix which will eventually lead to decrease in greenhouse gas emissions. This is because fossil fuels usage generates emissions, while renewable energy sources are widely regarded as carbon neutral. One form of renewable energy that can be used to generate electricity is wind energy. Wind is a widely available source of energy. Wind energy is a green source of power because wind turbine does not directly generate emissions, thereby assisting nations to achieve their emission reduction goals and tackling climate change. It has been shown that wind energy consumption leads to a decrease in CO2 emissions (Kuskaya and Bilgili, 2020 ). The usage of wind energy reduces the need for water consumption in the process of electricity generation. Relative to nuclear power, wind energy is a less expensive source of energy.
Unlike nuclear energy, the usage of wind energy is not associated with major disasters. Wind power has lower maintenance and operational costs compared to nuclear power. Kyshtym disaster of 1957, Chernobyl disaster of 1986, Tokaimura nuclear accident of 1999 and Fukushima Daiichi nuclear disaster of 2011 are some of the major nuclear disasters that have been experienced over the years. These disasters have led to several deaths as well as clean-up costs running into several millions of dollars. Exposure to nuclear materials such as uranium can generate health risks. Wind energy consumption can promote economic growth as the promotion of renewable energy use can attract energy-related investment from both local and foreign investors. For instance, global offshore wind investment in the globe increased quadrupled in the first 6 months of 2020 (which amounts to $35bn) despite being a coronavirus pandemic ravaged period (The Guardian, 2020 ).
As wind energy account for a small portion of the global electricity mix, the possibility of deriving all the benefits associated with wind energy is reliant on the degree at which it is feasible to change from other sources of energy (especially nuclear energy and fossil fuels) to wind energy in the electricity sector. For instance, in 2019, about 16.9 petawatt-hours or 63% of the aggregate electric power was produced from sources of fossil fuels, while 2.8 petawatt-hours or 10% of the aggregate electric power was produced from nuclear energy in the globe. Only 1.4 petawatt-hours or 5% of the aggregate electric power was produced from wind energy (British Petroleum, 2020 ).
However, it is difficult to find papers that have investigated the feasibility of replacing wind energy with fossil fuels or nuclear energy within the electricity sector. Many papers have concentrated on the inter-fuel substitution possibility between specific fossil fuels with specific renewable energy sources. Solarin and Bello ( 2019 ) have shown that it is possible to substitute coal, gas and oil with biomass in Brazil. Hossain and Serletis ( 2020 ) provided evidence for the feasibility of replacing biofuel for natural gas and biofuel for oil in the transportation industry of the USA. Tan and Lin ( 2020 ) provided evidence for substitution between coal and electricity, between electricity and oil, and between electricity and gas in China’s energy intensive industries. There are even studies that have concentrated on the feasibility of substituting electricity generation from one source of nonrenewable energy with another source of nonrenewable energy. For instance, Mugabe et al. ( 2020 ) illustrated that it is possible to substitute coal with natural gas in the USA.
The empirical findings generated from these studies might not necessarily be applicable for electricity generation. Moreover, policy options for primary energy consumption or generation might be different to those available for electricity generation. Hence, few studies have also concentrated on inter-fuel substitution for electricity generation. Lin and Ankrah ( 2019a , b ) illustrated that it is possible to shift from nonrenewable power generation system to renewable power generation system in Ghana and Nigeria, respectively. Kim ( 2019 ) disclosed that it is not possible to substitute nuclear power with renewable energy sources in Korea. Bello et al. ( 2020 ) suggested that it is possible to substitute coal and gas with hydroelectricity in the course of generating electric power in a south-east Asian country, Malaysia.
We aim to contribute to the existing body of work by investigating the feasibility of replacing the fossil fuels—natural gas and coal, as well as nuclear energy for wind power in the process of producing electric power in Germany. We have chosen Germany because of the following reasons. Firstly, Germany is not only among the biggest economies in the world, but also has the largest economy in Europe (World Bank, 2020 ). Secondly, being responsible for 16% of the aggregate energy consumption in 2019, the country has the biggest energy sector in the continent (British Petroleum, 2020 ). Thirdly, by producing carbon dioxide of 0.7 billion tonnes in 2019, the nation has the largest carbon dioxide in Europe and accounts for 17% of the aggregate carbon dioxide produced in the continent (British Petroleum, 2020). Fourthly, similar to the scenario in numerous economies, fossil fuels account for the bulk of electric power in Germany. About 0.3 petawatt-hours or 44% of the aggregate electric power was produced from fossil fuels sources. The total electricity generated through the renewable energy sources was 244 terawatt-hours, which is under 40% of the total. Only 0.1 petawatt-hours or 21% of the aggregate electricity was produced from wind energy (British Petroleum, 2020 ). Hence, this has made the power sector to be a key source of the country’s emissions. Production of heat and electricity led to CO2 emissions of 303 million tonnes or 42% of the aggregate fuel combustion induced CO2 emissions in Germany in 2017 (International Energy Association, 2020 ).
Fifthly, the government in Germany has introduced several policies and initiatives aimed at improving the popularity of wind energy. Some of these initiatives and policies include Offshore Grid Development Plan of 2005, Electricity Grid Development Plan of 2005, Energy Act passed in 2011 and Renewable Energy Act of 2017. Sixthly, the use of nuclear power to generate electricity has decreased substantially over the years. For instance, nuclear energy accounted for 133 terawatt-hours of electricity generated or 28% of the total electricity generated in 2010. However, by 2015, nuclear energy accounted for 86.8 terawatt-hours of electricity generated or 16% of the total electricity generated (which still made Germany, the seventh-biggest generator of nuclear energy in the globe). The German government approved the amendment of the Nuclear Energy Act of 2002, designed at decreasing reliance on nuclear energy in the country (World Wind Energy Association, 2018 ). Aftermath of the Fukushima disaster in Japan in March 2011, the authorities in Germany choose to shut down eight reactors that were in existent before 1980 due to public complaints and to shut down the remaining nine nuclear reactors in Germany before 2022 (Energy Information Administration, 2016 ).
The remaining parts of the paper are arranged in the following form. Section 2 describes the methodology, as well as the datasets that have been employed in this study. Section 3 presents the empirical findings and discussion resulting from the findings, while Sect. 4 contains the conclusion and policy implications of the paper.
Methodology
We begin by specifying the following production function:
where output ( Y ) depends on Energy ( E ) , Labour ( L ) and physical capital stock ( K ) . Energy (E) is supposed to be homothetic and weakly separable in its various components. For Germany, the main energy types that we have considered in this research are Coal ( C ), Gas ( G ), Wind ( W ) and Nuclear energy ( N ). Thus, segregating energy into its sub-components, Eq. ( 1 ) is re-written as:
We then transform Eq. ( 2 ) into a double differentiable transcendental logarithmic or trans-log production specification as follows:
Equation ( 1 ) is the general form of a second-order Taylor Series representation where Y is the output and the X s is the various units of input combinations (i.e. K, L, C, G, W, and N ) with subscripts i and j representing such combinations. Subscript t denotes period, the α s is the parameter estimates while ln shows that the variables are in their natural logarithm forms.
To avoid overparameterization, we have reduced the number of estimable parameters by including just the trans-log components of the energy elements as these are the focus of this study. Thus, the specific trans-log production function is stated as follows:
The parameter estimates of specification in ( 4 ) are used to derive the estimates of the output of elasticities of each of the energy sources. The output elasticity estimates are subsequently employed to produce the estimates of substitution coefficients between the energy inputs.
The output elasticity of an input i is computed as:
Thus, for the respective energy inputs ( C ) , ( G ) , ( W ) and ( N ) , the output elasticity is obtained as:
where η Ct , η Gt , η Wt , and η Nt specify elasticities of Coal, Gas, Wind, and Nuclear energy outputs, respectively. Given the estimates of the output elasticities of the energy inputs, the estimates of the substitution elasticities between the energy inputs are given as follows: σ ij = 1 + 2 α ij - α ii ( η j / η i ) - α jj ( η i / η j ) . η i + η j - 1 - 1 ( i ≠ j ; = c , g , w , n ) 10
The elasticity estimates are symmetry, i.e. ( σ ij = σ ji ) . The substitution elasticity between the respective energy pairs is therefore calculated as:
In Eqs. ( 11 – 16 ), positive estimate values imply that the energy pairs are substitutes while negative values connote complementary relationships between the energy pairs.
The dataset entails annual time series data on production, stock of physical capital, labour, coal, gas, wind energy and nuclear energy for Germany spanning the period 1986 to 2018. We have not included oil in the analysis as the country generates a negligible amount of electricity from it. Real GDP is used as proxy for output, while data for physical capital stock are represented by the Gross Fixed Capital Formation (GFCF). To address inflationary trend, data on both the real GDP and the GFCF have been obtained at constant 2010US$ from the world development indicators of the World Bank ( 2020 ). Labour, obtained from the data and analysis section of the Conference Board ( 2020 ), is computed as persons employed (in thousands per persons). Data on the energy series, in million tonnes of oil equivalent (MTOE), were sourced from the Statistical Review of World Energy, which is being printed by British Petroleum (BP) ( 2020 ).
Ridge regression procedure
Extreme multicollinearity can cause serious problem in model estimation. This is especially the case when the model includes squared exponential explanatory terms like the trans-log model in Eq. ( 4 ). A severe case of multicollinearity usually exaggerates the standard errors of the parameter estimates and reduces the t-statistics. This does not only lead to insignificant probability values and grossly inaccurate parameter estimates but also ultimately results into loss of overall projecting ability of the model. In such case, the use of the conventional ordinary least square procedure becomes not only inconsistent but also misleading. To ascertain the propriety or otherwise of the OLS procedure, we commence the estimation procedure by first conducting a test for multicollinearity through the examination of the variance inflation factors of the regressors and the condition number of the Eigenvalues of correlation of the variables. The outcomes of the multicollinearity test, displayed in Table Table1, 1 , shows that not only is the variance inflation factors for each of the regressors significantly exceed 10 but also the condition number of the Eigenvalues of correlation of some series exceeds 100 thereby establishing the existence of an extreme multicollinearity problem and rendering the application of the OLS technique unsuitable in this circumstance.
Least squares multicollinearity test result
Independent variable | Variance inflation factors | Eigenvalues | Condition number |
---|---|---|---|
InK | 25.0023 | 10.7306 | 1.00000 |
InL | 28.1082 | 3.460019 | 3.10000 |
InC | 203,922.7 | 1.088957 | 9.85000 |
InG | 729,133.1 | 0.468429 | 22.9100 |
InW | 1,014,980 | 0.11589 | 92.5900 |
InN | 114,518.5 | 0.085683 | 125.240 |
InC*InG | 591,200.9 | 0.049124 | 218.440 |
InC*InW | 1,052,053 | 0.000667 | 16,082.73 |
InC*InN | 203,274.8 | 0.000493 | 21,766.77 |
InG*InW | 44,959.23 | 0.000064 | 168,348.6 |
InG*InN | 13,216.56 | 0.000036 | 302,184.1 |
InW*InN | 22,378.5 | 0.000023 | 461,031.1 |
InC*InC | 251,670.7 | 0.000010 | 1,103,073 |
InG*InG | 41,096.09 | 0.000002 | 4,538,996 |
InW*InW | 82.5726 | 0.000001 | 7,274,368 |
InN*InN | 26,460.14 | 0.000000 | 32,351,833 |
Multicollinearity is severe as the variance inflation factors exceeds 10 and some condition figures of the Eigenvalues of correlations of some series exceed 100
To circumvent these challenges, Hoerl ( 1962 ) developed a unique regression procedure called the ridge regression procedure. The ridge regression approach involves the modification of the OLS parameter estimate by introducing a penalty parameter knows as a biasing constant ( c ). Therefore, the original matrix for the OLS coefficient estimate α ols = ( x ′ x ) - 1 x ′ y is modified into a ridge expression as α ridge = ( x ′ x + c I ) - 1 x ′ y where c is the penalty term whose values ranges from 0 to 1 and I is an identity matrix. The penalty parameter that is equal to zero corresponds to the OLS estimates.
Hoerl and Kennard ( 1970 ) suggested the usage of the ridge trace as a methodical means of finding the optimum value of c . The ridge trace plots the ridge regression coefficients as a function of c, and the value of c, for which the regression coefficients stabilise, is selected as the optimum. A ridge parameter of 0.184 has been selected as the optimum value of c based on the ridge trace plot shown in Fig. 1 as the parameter estimates seem to stabilise around this value.

Ridge trace plot
Furthermore, Table Table2 2 is also used to show the effect of the ridge regression method on the variance inflation factors. As can be seen, changing the penalty parameters decreases the variance inflation factors. The zero value of the penalty parameter corresponds to the variance inflation factors for the OLS estimates which are very large, but steady increase in the penalty parameter continues to decrease the variance inflation factors until the value of 0.184 where the variance inflation factors for all variables have come under 10 and multicollinearity successfully addressed.
Effect of penalty parameter ( c ) on the variance inflation factor
InK | InL | InC | InG | InW | InN | InC*InG | InC*InW | InC*InN | InG*InW | |
---|---|---|---|---|---|---|---|---|---|---|
0.000 | 25.0023 | 28.1082 | 203,922.678 | 729,133.1409 | 1,014,980.07 | 114,518.46 | 591,200.88 | 1,052,053.02 | 203,274.78 | 44,959.23 |
0.001 | 15.7012 | 8.0505 | 10.4708 | 35.0329 | 22.0789 | 27.0848 | 41.8638 | 24.4309 | 19.3971 | 83.3271 |
0.002 | 15.0375 | 7.8605 | 3.6344 | 12.7224 | 8.44 | 8.868 | 15.7853 | 8.8509 | 6.4734 | 32.0868 |
0.003 | 14.4556 | 7.6978 | 2.195 | 6.8102 | 4.8636 | 4.8134 | 8.595 | 5.0181 | 3.5104 | 17.2519 |
0.004 | 13.916 | 7.5448 | 1.6579 | 4.3957 | 3.3932 | 3.2592 | 5.6091 | 3.4791 | 2.3565 | 10.9505 |
0.080 | 2.4662 | 2.5732 | 0.6382 | 0.284 | 0.3845 | 0.3839 | 0.3881 | 0.3994 | 0.2356 | 0.3367 |
0.090 | 2.14 | 2.3205 | 0.6132 | 0.2585 | 0.3474 | 0.3499 | 0.3538 | 0.3612 | 0.2136 | 0.3012 |
0.100 | 1.8767 | 2.1043 | 0.59 | 0.2371 | 0.3161 | 0.3212 | 0.3249 | 0.329 | 0.1952 | 0.272 |
:: | :: | :: | :: | :: | :: | :: | :: | :: | :: | :: |
:: | :: | :: | :: | :: | :: | :: | :: | :: | :: | :: |
InG*InN | InW*InN | InC*InC | InG*InG | InW*InW | InN*InN | |
---|---|---|---|---|---|---|
0.000 | 13,216.56 | 22,378.5 | 251,670.7 | 41,096.09 | 82.5726 | 26,460.14 |
0.001 | 103.5917 | 54.819 | 7.3 | 109.4797 | 5.6909 | 61.1214 |
0.002 | 37.3583 | 17.7174 | 2.6427 | 42.8218 | 5.4807 | 21.693 |
0.003 | 19.2867 | 9.2529 | 1.7088 | 23.0087 | 5.3322 | 11.5219 |
0.004 | 11.849 | 5.9881 | 1.3669 | 14.5278 | 5.2008 | 7.4141 |
0.080 | 0.2899 | 0.455 | 0.6312 | 0.3774 | 1.7139 | 0.4527 |
0.090 | 0.2716 | 0.4101 | 0.6066 | 0.3389 | 1.5616 | 0.4098 |
0.100 | 0.2566 | 0.3724 | 0.5839 | 0.3074 | 1.4326 | 0.374 |
:: | :: | :: | :: | :: | :: | :: |
:: | :: | :: | :: | :: | :: | :: |
The first row with a c value of 0 corresponds to the VIFs of the OLS procedure. The last row with a c value of 0.426 is the VIF of the ridge regression procedure which neutralised the severe multicollinearity problem. Source: Authors’ computations
Results and discussion
We begin the discussion of the results by presenting the results of the parameter estimates of the ridge regression procedure in Table Table3. 3 . The table shows the figures of the variance inflation factor for each of the parameter, and as can be observed, these figures are less than 10, in so doing, supporting the fact that the issue of multicollinearity has been successfully resolved. In addition to this, the f -ratio is 1% significant with a 97.1% R -square signifying a robust goodness of fit and explanatory power of the parameters in the regression.
Ridge regression parameter estimates
Source: Authors’ computations
Independent variable | Parameter estimates | -stat | Variance inflation factor |
---|---|---|---|
Constant | 18.604 | ||
InK | 0.193 | 4.479 | 0.801 |
InL | 0.290 | 1.858 | 1.062 |
InC | − 0.008 | − 0.130 | 0.439 |
InG | 0.020 | 2.957 | 0.137 |
InW | 0.006 | 7.373 | 0.170 |
InN | − 0.036 | − 3.181 | 0.186 |
InC*InG | 0.005 | 2.880 | 0.189 |
InC*InW | 0.001 | 7.283 | 0.178 |
InC*InN | − 0.005 | − 3.493 | 0.111 |
InG*InW | 0.002 | 7.796 | 0.141 |
InG*InN | 0.002 | 0.844 | 0.186 |
InW*InN | 0.001 | 6.868 | 0.197 |
InC*InC | − 0.001 | -0.161 | 0.435 |
InG*InG | 0.002 | 2.436 | 0.168 |
InW*InW | − 0.003 | − 0.464 | 0.819 |
InN*InN | − 0.004 | − 2.883 | 0.209 |
R Squared: 0.971 | |||
F-ratio: 33.0621 (0.000) |
A implies 1% level of significance, C implies 10% level of significance, Figures in parenthesis are probability values.
From the parameter estimates of the ridge regression depicted in Table Table3, 3 , the output elasticities of each of the energy inputs are obtained using Eqs. ( 6 ), ( 7 ), ( 8 ) and ( 9 ), respectively, for coal, gas, wind, and nuclear energy and the empirical findings are depicted in Table Table4. 4 . The result shows that the average output elasticities for both gas and wind energy are positive, while the corresponding figures for both coal and nuclear are negative. This outcome is not totally surprising as wind energy is considered a cleaner energy with an increasing share in the energy profile of Germany over the recent years. Similarly, gas is considered less harmful compared to other fossil fuel like coal which is perhaps most environmentally detrimental to the environment after oil, while nuclear, on the other hand, has been experiencing a downward trend in the energy profile of Germany. The estimates of the output elasticities are employed to compute the elasticities of substitution estimates between the energy pairs. These are given in Table Table5 5 with positive estimate values between all energy inputs averaging unity. This implies that all energy pairs considered in this study for Germany are substitutes. With specific reference to wind, the highest substitutability estimate is nuclear energy, followed by gas and then coal.
Output elasticity estimates
Year | ||||
---|---|---|---|---|
1986 | − 0.031 | 0.058 | 0.027 | − 0.100 |
1987 | − 0.031 | 0.060 | 0.027 | − 0.100 |
1988 | − 0.029 | 0.062 | 0.026 | − 0.099 |
1989 | − 0.028 | 0.065 | 0.026 | − 0.097 |
1990 | − 0.027 | 0.066 | 0.025 | − 0.096 |
1991 | − 0.026 | 0.066 | 0.025 | − 0.095 |
1992 | − 0.026 | 0.067 | 0.025 | − 0.094 |
1993 | − 0.025 | 0.068 | 0.024 | − 0.093 |
1994 | − 0.024 | 0.069 | 0.024 | − 0.092 |
1995 | − 0.023 | 0.071 | 0.024 | − 0.091 |
1996 | − 0.022 | 0.072 | 0.024 | − 0.091 |
1997 | − 0.022 | 0.073 | 0.024 | − 0.091 |
1998 | − 0.021 | 0.073 | 0.024 | − 0.090 |
1999 | − 0.021 | 0.074 | 0.024 | − 0.090 |
2000 | − 0.020 | 0.075 | 0.023 | − 0.089 |
2001 | − 0.020 | 0.075 | 0.024 | − 0.089 |
2002 | − 0.019 | 0.076 | 0.023 | − 0.088 |
2003 | − 0.018 | 0.077 | 0.023 | − 0.088 |
2004 | − 0.018 | 0.077 | 0.023 | − 0.088 |
2005 | − 0.017 | 0.078 | 0.023 | − 0.087 |
2006 | − 0.017 | 0.078 | 0.023 | − 0.087 |
2007 | − 0.016 | 0.079 | 0.023 | − 0.086 |
2008 | − 0.015 | 0.079 | 0.023 | − 0.086 |
2009 | − 0.015 | 0.078 | 0.023 | − 0.085 |
2010 | − 0.015 | 0.079 | 0.023 | − 0.085 |
2011 | − 0.014 | 0.078 | 0.023 | − 0.083 |
2012 | − 0.014 | 0.078 | 0.023 | − 0.083 |
2013 | − 0.014 | 0.078 | 0.022 | − 0.083 |
2014 | − 0.015 | 0.077 | 0.022 | − 0.083 |
2015 | − 0.014 | 0.078 | 0.022 | − 0.082 |
2016 | − 0.012 | 0.078 | 0.022 | − 0.081 |
2017 | − 0.011 | 0.079 | 0.022 | − 0.079 |
2018 | − 0.011 | 0.078 | 0.022 | − 0.079 |
Average | − 0.020 | 0.073 | 0.024 | − 0.089 |
Substitution elasticity estimates
Year | ||||||
---|---|---|---|---|---|---|
1986 | 0.7623 | 0.9548 | 0.8101 | 0.9773 | 1.1906 | 0.9822 |
1987 | 0.7836 | 0.9554 | 0.8093 | 0.9766 | 1.1890 | 0.9821 |
1988 | 0.8095 | 0.9559 | 0.8080 | 0.9750 | 1.1874 | 0.9820 |
1989 | 0.8393 | 0.9566 | 0.8056 | 0.9739 | 1.1867 | 0.9817 |
1990 | 0.8526 | 0.9565 | 0.8032 | 0.9726 | 1.1885 | 0.9815 |
1991 | 0.8592 | 0.9565 | 0.8020 | 0.9721 | 1.1894 | 0.9814 |
1992 | 0.8667 | 0.9564 | 0.8009 | 0.9710 | 1.1902 | 0.9813 |
1993 | 0.8780 | 0.9564 | 0.7989 | 0.9701 | 1.1922 | 0.9811 |
1994 | 0.8886 | 0.9566 | 0.7974 | 0.9698 | 1.1937 | 0.9809 |
1995 | 0.9007 | 0.9570 | 0.7960 | 0.9696 | 1.1954 | 0.9807 |
1996 | 0.9080 | 0.9574 | 0.7958 | 0.9696 | 1.1958 | 0.9807 |
1997 | 0.9136 | 0.9576 | 0.7951 | 0.9693 | 1.1972 | 0.9806 |
1998 | 0.9224 | 0.9577 | 0.7935 | 0.9690 | 1.2006 | 0.9804 |
1999 | 0.9244 | 0.9577 | 0.7933 | 0.9689 | 1.2013 | 0.9804 |
2000 | 0.9286 | 0.9576 | 0.7927 | 0.9683 | 1.2039 | 0.9803 |
2001 | 0.9349 | 0.9580 | 0.7921 | 0.9685 | 1.2060 | 0.9802 |
2002 | 0.9414 | 0.9579 | 0.7906 | 0.9681 | 1.2113 | 0.9801 |
2003 | 0.9483 | 0.9583 | 0.7901 | 0.9682 | 1.2153 | 0.9800 |
2004 | 0.9518 | 0.9582 | 0.7894 | 0.9679 | 1.2194 | 0.9799 |
2005 | 0.9602 | 0.9585 | 0.7880 | 0.9680 | 1.2270 | 0.9797 |
2006 | 0.9624 | 0.9586 | 0.7880 | 0.9681 | 1.2294 | 0.9797 |
2007 | 0.9736 | 0.9585 | 0.7849 | 0.9676 | 1.2501 | 0.9793 |
2008 | 0.9790 | 0.9587 | 0.7844 | 0.9679 | 1.2574 | 0.9791 |
2009 | 0.9777 | 0.9582 | 0.7828 | 0.9674 | 1.2576 | 0.9790 |
2010 | 0.9810 | 0.9586 | 0.7832 | 0.9677 | 1.2618 | 0.9790 |
2011 | 0.9947 | 0.9580 | 0.7787 | 0.9669 | 1.3143 | 0.9784 |
2012 | 0.9915 | 0.9577 | 0.7785 | 0.9666 | 1.3094 | 0.9784 |
2013 | 0.9851 | 0.9575 | 0.7791 | 0.9663 | 1.2924 | 0.9786 |
2014 | 0.9814 | 0.9571 | 0.7788 | 0.9659 | 1.2858 | 0.9786 |
2015 | 0.9897 | 0.9570 | 0.7770 | 0.9655 | 1.3276 | 0.9784 |
2016 | 1.0120 | 0.9575 | 0.7739 | 0.9659 | 1.5351 | 0.9778 |
2017 | 1.0307 | 0.9573 | 0.7703 | 0.9655 | − 1.7761 | 0.9773 |
2018 | 1.0294 | 0.9570 | 0.7698 | 0.9652 | − 4.9650 | 0.9773 |
Average | 0.9260 | 0.9574 | 0.7910 | 0.9692 | 1.1414 | 0.9800 |
The foregoing results suggest that wind energy has positive impact on economic growth, as well as being able to substitute nuclear energy, coal and natural gas in Germany. The results can be attributed to several reasons. First, wind power is cost-effective in many regions. Wind deployment is associated with integrated costs including balancing costs (arising from uncertainty that is connected to the adoption of wind power); grid costs (which are associated with the adaptation of the grid to wind energy generation), and profile costs (which are associated with the need for backup capacity, particularly during peak-load periods). The integrated costs are collectively less than the costs that would have been incurred if most of the alternative sources of electricity were being utilised. Its appeal has risen over the years because of its substantial cost reduction and its non-susceptibility to oil price volatility (Ortega-Izquierdo & del Río, 2020 ).
Another rationale for the above the results is the smaller space required by wind technology and the flexibility in its use. Wind energy stations usually require less space than conventional power stations. Therefore, the freed space and land resulting from the shift to wind energy can be employed for other productive projects. Wind turbines can be mounted in locations that are remote in nature. Besides, wind farms capacity is changeable in line with the energy and electricity needs. It is possible to build wind turbines on existing ranches or farms. This significantly aids the productive activities in rural areas. It is possible for ranchers and farmers to continue to work on their land because the wind turbines occupy only a portion of the land. For their use of the leased land, the owners of wind power plant regularly pay rents to the ranchers or farmers, offering landowners with extra income.
The foregoing results can also be justified on the basis that increase in investment has accompanied the deployment of wind energy in Germany. The deployment of wind energy technologies requires both public and private investments. In Germany, public investment on wind energy technologies has increased over the years. For instance, the government support for technology increased nine-fold from 740 million euro in 2009 to 6520 million euro in 2016 (Ortega-Izquierdo & del Río, 2020 ). Investment in new technology increases productivity and the productive capacity of the economy, which assists to shift the long-run aggregate supply to the right. An increase in long-run aggregate supply is essential for long-term economic growth. Investment leads to a substantial rise in productivity, as well as an increase in the productive capacity of the country.
Another justification for the foregoing empirical findings is that wind is a domestic source of energy. Hence, substituting fossil fuels with wind energy is likely to reduce the dependence of the country on imported fuels, which grain the resources. The saved resources, as a result of the deployment of wind energy, can be used to fund other productive activities such as research and development. Germany is regarded as the biggest importer of natural gas in the globe. The largest gas imports come from the Netherlands, Norway and Russia via the Nord Stream. Imports account for about 90% of total natural gas supply (Energy Information Administration, 2016 ).
In this paper, we have used the trans-log production approach to investigate the inter-fuel substitution elasticity between wind energy, nuclear energy and fossil fuels for the period 1986–2018 in the electricity sector of Germany. Due to the existence of multicollinearity among the regressors, the ridge regression approach has been used in the estimation process. The results reveal that wind and natural gas have positive output elasticity estimates, while the estimates for nuclear and coal are negative. We also found that all the inputs pairs have positive substitution elasticity estimates between them. With respect to wind energy, the highest substitutability estimate occurred with nuclear power which is followed by natural gas and then coal.
One of the policy implications of wind energy having positive output elasticity is that attempts of the authorities to increase wind energy will positively contribute to the economy through expansion in the country’s gross domestic product. This also implies that negative shocks to wind energy consumption will negatively affect economic growth. Although natural gas also has a positive impact on economic growth, expansion of natural gas will lead to more emission in the economy due to its nature as a carbon emitting fossil fuel.
The implication of the results that provides evidence for substitutability among the inputs is that it is possible to substitute coal, natural gas and nuclear energy for wind energy in the electricity sector. On the one hand, wind energy can be used to substitute coal and natural gas, which cause emission. One the other hand, as the government in Germany continues to wind down the use of nuclear reactors, nuclear energy can be replaced with renewable energy sources, especially wind energy as against using substituting nuclear energy with fossil fuels. This possibility is underscored by the fact that only about 24% of the 60,822 megawatts of the installed wind turbine capacity was utilised in 2019 (British Petroleum, 2020 ). Therefore, the country has the capacity to increase wind energy for electricity generation.
Hence, it is recommended that several policies should be introduced to encourage the usage of wind energy in the economy. One of such policies is the introduction of tax credit for wind energy technology. Such initiative is likely to decrease net project costs to consumers, encourage the adoption of wind energy technologies and boost market acceptance of clean energy projects. Another policy option that will yield the same result is the reduction in property taxes for wind power facilities. The effort to enhance grid connection guidelines, an effective financial system, the commitment on the part of the government to allocate fund for research and development (R&D), in addition to synergy between the wind industry, science sector and the state are other initiatives necessary for the success of the wind industry. There is also a need for a mix of public policies that lead to an enabling atmosphere for the success of the wind industry.
The government in Germany has been initiating policies in this direction. For instance, the authorities in Germany have introduced remuneration scheme and the Feed-In-Tariff (FiT), which has led to greater longevity, transparency, and certainty for investors in the wind energy market. Besides, there has been an increase in the financing and deployment of wind energy technologies and erecting of wind turbines across the country (World Wind Energy Association, 2018 ). Therefore, the government should continue on the path of introducing policies aimed at encouraging wind energy development in the country.
Publisher's Note
Springer Nature remains neutral with regard to jurisdictional claims in published maps and institutional affiliations.
Contributor Information
Sakiru Adebola Solarin, Email: moc.liamg@niralosurikas .
Mufutau Opeyemi Bello, Email: moc.oohay@4002_ytthpum .
- Bello MO, Solarin SA, Yen YY. Interfuel substitution, hydroelectricity consumption and CO2 emissions mitigation in Malaysia: Evidence from a transcendental logarithm (trans-log) cost function framework. Environmental Science and Pollution Research. 2020; 27 :17162–17174. doi: 10.1007/s11356-020-08251-z. [ PubMed ] [ CrossRef ] [ Google Scholar ]
- British Petroleum (BP) (2020) Statistical Review of World Energy. Available at https://www.bp.com/en/global/corporate/energy-economics/statistical-review-of-world-energy.html [25 July, 2020]
- Energy Information Administration (2016). Country Brief, Germany. Available at https://www.eia.gov/international/analysis/world [25 July, 2020]
- Hoerl AE. Applications of ridge analysis to regression problems. Chemical Engineering Progress. 1962; 58 (3):54–59. [ Google Scholar ]
- Hoerl AE, Kennard RW. Ridge regression: Biased estimation for nonorthogonal problems. Technometrics. 1970; 12 (1):55–67. doi: 10.1080/00401706.1970.10488634. [ CrossRef ] [ Google Scholar ]
- Hossain AN, Serletis A. Biofuel substitution in the US transportation sector. The Journal of Economic Asymmetries. 2020; 22 :e00161. doi: 10.1016/j.jeca.2020.e00161. [ CrossRef ] [ Google Scholar ]
- International Energy Association (2020). IEA World Energy Balances 2020. Available at https://www.iea.org/reports/world-energy-balances-overview [25 July, 2020]
- Kim K. Elasticity of substitution of renewable energy for nuclear power: Evidence from the Korean electricity industry. Nuclear Engineering and Technology. 2019; 51 (6):1689–1695. doi: 10.1016/j.net.2019.04.005. [ CrossRef ] [ Google Scholar ]
- Kuşkaya S, Bilgili F. The wind energy-greenhouse gas nexus: The wavelet-partial wavelet coherence model approach. Journal of Cleaner Production. 2020; 245 :118872. doi: 10.1016/j.jclepro.2019.118872. [ CrossRef ] [ Google Scholar ]
- Lin B, Ankrah I. Renewable energy (electricity) development in Ghana: Observations, concerns, substitution possibilities, and implications for the economy. Journal of Cleaner Production. 2019; 233 :1396–1409. doi: 10.1016/j.jclepro.2019.06.163. [ CrossRef ] [ Google Scholar ]
- Lin B, Ankrah I. On Nigeria’s renewable energy program: Examining the effectiveness, substitution potential, and the impact on national output. Energy. 2019; 167 :1181–1193. doi: 10.1016/j.energy.2018.11.031. [ CrossRef ] [ Google Scholar ]
- Mugabe D, Elbakidze L, Zaynutdinova G. Elasticity of substitution and technical efficiency: Evidence from the US electricity generation. Applied Economics. 2020; 52 (16):1789–1805. doi: 10.1080/00036846.2019.1678733. [ CrossRef ] [ Google Scholar ]
- Ortega-Izquierdo M, del Río P. An analysis of the socioeconomic and environmental benefits of wind energy deployment in Europe. Renewable Energy. 2020; 160 :1067–1080. doi: 10.1016/j.renene.2020.06.133. [ CrossRef ] [ Google Scholar ]
- Solarin SA, Bello MO. Interfuel substitution, biomass consumption, economic growth, and sustainable development: Evidence from Brazil. Journal of Cleaner Production. 2019; 211 :1357–1366. doi: 10.1016/j.jclepro.2018.11.268. [ CrossRef ] [ Google Scholar ]
- Tan R, Lin B. The influence of carbon tax on the ecological efficiency of China’s energy intensive industries—A inter-fuel and inter-factor substitution perspective. Journal of Environmental Management. 2020; 261 :110252. doi: 10.1016/j.jenvman.2020.110252. [ PubMed ] [ CrossRef ] [ Google Scholar ]
- The Conference Board. (2020). Economic data and analysis. Available at: https://www.conference-board.org/data/ [25 July, 2020]
- The Guardian (2020). Offshore wind energy investment quadruples despite Covid-19 slump. Available at https://www.theguardian.com/environment/2020/jul/13/offshore-wind-energy-investment-quadruples-despite-covid-19-slump [26 June, 2020]
- World Bank Group (2020). World Development Indicators 2020. World Bank Publications.
- World Wind Energy Association (2018). World wind energy association policy paper series. Available at: https://wwindea.org/wp-content/uploads/2018/06/Germany_Full.pdf [25 July 2020]
ENCYCLOPEDIC ENTRY
Wind energy.
Scientists and engineers are using energy from the wind to generate electricity. Wind energy, or wind power, is created using a wind turbine.
Earth Science, Climatology
As renewable energy technology continues to advance and grow in popularity, wind farms like this one have become an increasingly common sight along hills, fields, or even offshore in the ocean.
Photograph by inga spence / Alamy Stock Photo
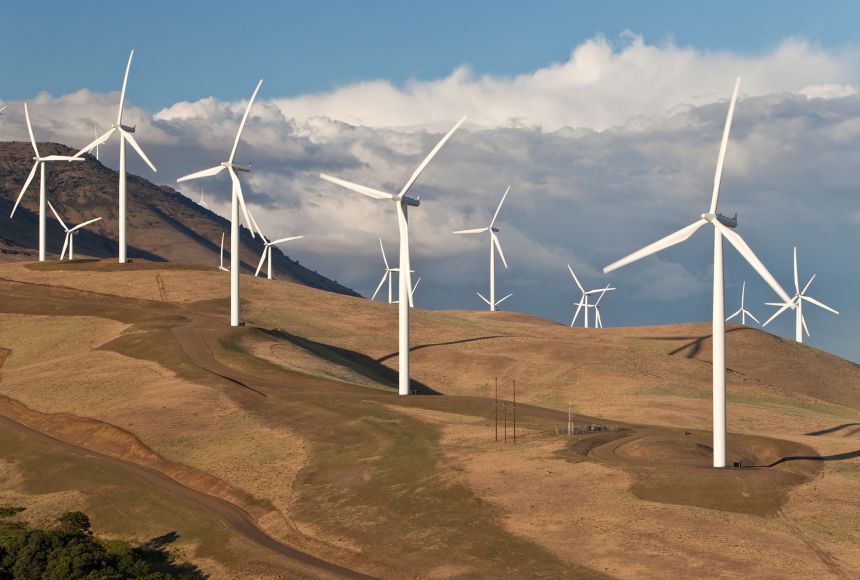
Anything that moves has kinetic energy , and scientists and engineers are using the wind’s kinetic energy to generate electricity. Wind energy , or wind power , is created using a wind turbine , a device that channels the power of the wind to generate electricity.
The wind blows the blades of the turbine , which are attached to a rotor. The rotor then spins a generator to create electricity. There are two types of wind turbines : the horizontal - axis wind turbines (HAWTs) and vertical - axis wind turbines (VAWTs). HAWTs are the most common type of wind turbine . They usually have two or three long, thin blades that look like an airplane propeller. The blades are positioned so that they face directly into the wind. VAWTs have shorter, wider curved blades that resemble the beaters used in an electric mixer.
Small, individual wind turbines can produce 100 kilowatts of power, enough to power a home. Small wind turbines are also used for places like water pumping stations. Slightly larger wind turbines sit on towers that are as tall as 80 meters (260 feet) and have rotor blades that extend approximately 40 meters (130 feet) long. These turbines can generate 1.8 megawatts of power. Even larger wind turbines can be found perched on towers that stand 240 meters (787 feet) tall have rotor blades more than 162 meters (531 feet) long. These large turbines can generate anywhere from 4.8 to 9.5 megawatts of power.
Once the electricity is generated, it can be used, connected to the electrical grid, or stored for future use. The United States Department of Energy is working with the National Laboratories to develop and improve technologies, such as batteries and pumped-storage hydropower so that they can be used to store excess wind energy. Companies like General Electric install batteries along with their wind turbines so that as the electricity is generated from wind energy, it can be stored right away.
According to the U.S. Geological Survey, there are 57,000 wind turbines in the United States, both on land and offshore. Wind turbines can be standalone structures, or they can be clustered together in what is known as a wind farm . While one turbine can generate enough electricity to support the energy needs of a single home, a wind farm can generate far more electricity, enough to power thousands of homes. Wind farms are usually located on top of a mountain or in an otherwise windy place in order to take advantage of natural winds.
The largest offshore wind farm in the world is called the Walney Extension. This wind farm is located in the Irish Sea approximately 19 kilometers (11 miles) west of the northwest coast of England. The Walney Extension covers a massive area of 149 square kilometers (56 square miles), which makes the wind farm bigger than the city of San Francisco, California, or the island of Manhattan in New York. The grid of 87 wind turbines stands 195 meters (640 feet) tall, making these offshore wind turbines some of the largest wind turbines in the world. The Walney Extension has the potential to generate 659 megawatts of power, which is enough to supply 600,000 homes in the United Kingdom with electricity.
Media Credits
The audio, illustrations, photos, and videos are credited beneath the media asset, except for promotional images, which generally link to another page that contains the media credit. The Rights Holder for media is the person or group credited.
Production Managers
Program specialists, last updated.
October 19, 2023
User Permissions
For information on user permissions, please read our Terms of Service. If you have questions about how to cite anything on our website in your project or classroom presentation, please contact your teacher. They will best know the preferred format. When you reach out to them, you will need the page title, URL, and the date you accessed the resource.
If a media asset is downloadable, a download button appears in the corner of the media viewer. If no button appears, you cannot download or save the media.
Text on this page is printable and can be used according to our Terms of Service .
Interactives
Any interactives on this page can only be played while you are visiting our website. You cannot download interactives.
Related Resources
Cornell Chronicle
- Architecture & Design
- Arts & Humanities
- Business, Economics & Entrepreneurship
- Computing & Information Sciences
- Energy, Environment & Sustainability
- Food & Agriculture
- Global Reach
- Health, Nutrition & Medicine
- Law, Government & Public Policy
- Life Sciences & Veterinary Medicine
- Physical Sciences & Engineering
- Social & Behavioral Sciences
- Coronavirus
- News & Events
- Public Engagement
- New York City
- Photos of the Week
- Big Red Sports
- Freedom of Expression
- Student Life
- University Statements
- Around Cornell
- All Stories
- In the News
- Expert Quotes
- Cornellians
Wind energy can help Earth blow back climate calamity
By blaine friedlander.
The answer to climate change – or at least part of it – is blowing in the wind, according to research published Aug. 28 in the journal Climate.
“Early action will reap dividends,” said Rebecca Barthelmie, professor in the Sibley School of Mechanical and Aerospace Engineering, in the College of Engineering. “In terms of averting the worst of climate change, our work confirms that accelerating wind-energy technology deployment is a logical and a cost-effective part of the required strategy. Waiting longer will mean more drastic action will be needed.”
Barthelmie and Sara C. Pryor, professor in the Department of Earth and Atmospheric Sciences, in the College of Agriculture and Life Sciences, calculated that implementing advance wind energy scenarios could achieve a reduction in global warming atmospheric average temperatures of 0.3 to 0.8 degrees Celsius by the end of the century.
To avert environmental disaster, other greenhouse gas reduction strategies will also need to be implemented, they said.
In early August, the Intergovernmental Panel on Climate Change (IPCC) Working Group I Sixth Assessment Report said that climate change is rapid and intensifying, and that Earth’s atmosphere could add 1.5 degrees C of average warming by 2040. To avoid further warming, the IPCC report said there must be transformational change.
“Our work shows that it is feasible for the United States to accelerate its deployment of wind energy,” Barthelmie said, “to substantially reduce carbon dioxide emissions and that will make a real difference to the kind of warming that the world endures.”
Global wind resources exceed current electricity demand, Pryor said, and the cost of energy from wind turbines has declined sharply. “It makes perfect sense to rapidly deploy wind energy as a key part of decarbonizing the electricity supply,” she said.
The global wind energy industry has been growing. Since 2005, the total installed capacity of global wind energy shows a 14% annualized growth rate for Asia, Europe and North America. Global wind energy electricity production expanded from 104 terawatt-hours (one trillion watts for one hour) in 2005 to 1,273 terawatt-hours in 2018, the paper said.
In 2019, wind energy generated approximately 6.5% of 26,600 terawatt-hours of global electricity demand. Six countries are generating more than 20% of their demand, while the United Kingdom, Germany and Spain are close to achieving 20% of electricity demand with wind energy. China has reported about 5% of its electricity supply from wind energy.
The United States generates 8.4% of its electricity from wind, as of 2020, with six states (Texas, Iowa, Oklahoma, California, Kansas and Illinois) containing more than half of wind energy capacity, according to the U.S. Energy Information Administration.
Wind turbines are now deployed in 90 countries, Barthelmie said, generating about 7% of global electricity, and the expansion of installed capacity of wind energy continues.
“While the scale of anthropogenic climate change is daunting, our research illustrates that wind energy can substantially reduce emissions of greenhouse gases at the national and global scale,” Barthelmie said, “and measurably reduce the amount of temperature increase.”
Sectors like solar and wind have become less expensive than fossil fuels. “So there really aren’t any arguments anymore for not making this kind of change,” Barthelmie said. “Both technically and economically, advanced deployment scenarios are feasible. It needs more political will.”
The research, “ Climate Change Mitigation Potential of Wind Energy ,” was funded by the U.S. Department of Energy. Barthelmie and Pryor are faculty fellows in the Cornell Atkinson Center for Sustainability .
Media Contact
Get Cornell news delivered right to your inbox.
You might also like
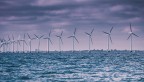
Gallery Heading

Environmental Impacts of Wind-Energy Projects (2007)
Chapter: 4 impacts of wind-energy development on humans, 4 impacts of wind-energy development on humans, introduction.
Although they have some unusual characteristics, such as visibility at a distance, wind-energy projects are not unique in their impacts on people. They share many characteristics with other projects—not only energy-production projects but also landfills, waste incinerators, etc.—that create both benefits and burdens. In considering how to undertake local interactions and how to temper negative socioeconomic impacts while enhancing benefits, much can be learned from past experiences with other potentially controversial issues.
One important lesson—and an important prelude to this chapter—is that concern about visual, auditory, and other impacts is a natural reaction, especially when the source of the impacts is or will be close to one’s home. The project’s potential for negative impacts as well as benefits, and the fact that different people have different values as well as different levels of sensitivity, are important aspects of impact assessment.
This chapter addresses some key potential human impacts, positive and negative, of wind-energy projects on people in surrounding areas. The impacts discussed here include aesthetic impacts; impacts on cultural resources such as historic and archeological sites and recreation sites; impacts on human health and well-being, specifically, from noise and from shadow flicker; economic and fiscal impacts; and the potential for electromagnetic interference with television and radio broadcasting, cellular phones, and radar.
The topics covered in this chapter do not represent an exhaustive list
of all possible human impacts from wind-energy projects. For example, we have not addressed potentially significant social impacts on community cohesion, sometimes exacerbated by differences in community make-up (e.g., differences in values and in amounts and sources of wealth between newcomers and long-time residents). Also not covered are psychological impacts—positive as well as negative—that can arise in confronting a controversial project (Gramling and Freudenburg 1992; NRC 2003). We have not focused on these matters because they can vary greatly from one local region or project site to another; and also as a function of population density and local and regional economic, social, and economic conditions; and in other ways. As a result, it is very difficult to generalize about them. In addition, not covered in this chapter but discussed elsewhere in this report (see especially Chapter 2 ) are diffuse health and economic effects of wind-energy projects. The topics covered in this chapter are, however, the chief local environmental impacts that have been recognized to date.
Thus far, there has been relatively little dispassionate analysis of the human impacts of wind-energy projects. Much that has been written has been from the vantage points of either proponents or opponents. There also are few data that have been systematically gathered on these impacts. In the absence of extensive data, this chapter is focused mainly on appropriate methods for analysis and assessment and on recommended practices in the face of uncertainty. Several of the methods discussed follow general principles and practice in socioeconomic impact assessments conducted as part of environmental impact statements; nevertheless, the chapter is tailored to the potential local human impacts of wind-energy projects and to their predominantly rural settings.
Wind-energy projects, like other potentially controversial developments, vary in their social context and thus in their social complexity. In this chapter, comments and methodological recommendations are directed toward relatively complex wind-energy facilities such as those being proposed for the Mid-Atlantic Highlands. While still applicable to smaller, less controversial installations, recommended methods should be simplified accordingly.
AESTHETIC IMPACTS
Aesthetics is often a primary reason for expressed concern about wind-energy projects ( Figure 4-1 ). Unfortunately, few regulatory review processes adequately address aesthetic issues, and far fewer address the unique aesthetic issues associated with wind-energy projects in a rational manner. This section begins by describing some of the aesthetic issues associated with wind-energy projects. It then discusses existing methods for identifying visual resources and evaluating visual impacts in general, and it

FIGURE 4-1 View of Mountaineer Project from .5 mile. The project includes a total of 44 wind turbines.
SOURCE: Photograph by Jean Vissering.
provides recommendations for adapting those methods to the assessment of visual impacts associated with wind-energy projects. Finally, the section briefly examines the potential for developing guidelines to protect scenic resources when planning for, siting, and evaluating prospective wind-energy projects.
Visual impacts are the focus of this discussion of aesthetic impacts, but noise is considered to the extent that it is related to the overall character of a particular landscape. Noise and shadow flicker are discussed further in this chapter, under the section addressing potential impacts on human health and well-being associated with wind-energy projects.
Aesthetic Issues
The essence of aesthetics is that humans experience their surroundings with multiple senses. We often have a strong attachment to place and an inherent tendency to protect our “nest.” Concern over changes in our personal landscapes is a universal phenomenon; it is not limited to the United States or to the present day. Public perceptions of wind-energy projects vary widely. To some, wind turbines appear visually pleasing, while others view them as intrusive industrial machines. Unlike some forms of development (e.g., cell towers), there are many people who find wind turbines to
be beautiful. Nevertheless, even beautiful objects may not be desirable in one’s current surroundings. Research has shown strong support for wind energy generally but substantially less support for projects close to one’s home (Thayer and Hansen 1989; Wolsink 1990; Gipe 2002).
There are a number of reasons why proposed wind-energy projects evoke strong emotional reactions. Modern wind turbines are relatively new to the United States. Some of the early projects were built in remote areas, but increasingly, they are being built in or proposed for areas that are close to residential and recreational uses, and often in areas never before considered for industrial land uses. They must be sited where wind resources, transmission lines, and access exist; in some cases, particularly in the eastern United States, these sites are relatively high in elevation (e.g., mountain ridgelines) and highly visible. Some projects extend over fairly extensive land areas, though only small portions of the area are occupied by the turbines themselves. The turbines 1 often are taller than any local zoning ordinance ever envisioned, and they are impossible to screen from view. The movement of the blades makes it more likely that they will draw attention (Thayer and Hanson 1988; Gipe 2002).
Federal Aviation Administration obstruction lighting (pulsing red or white lights at night) is another aesthetic issue, and one that may result in some of the greatest aesthetic concerns (Hecklau 2005). In addition, wind turbines may produce noise, and the movement of the blades can result in shadow flicker from certain vantage points. Both the noise and the shadow flicker can be aesthetically troubling for some people who live nearby. While less concern has been raised about other project infrastructure such as meteorological towers, roads, power lines, and substations along with their associated site clearing and regrading, these can also result in negative visual impacts. Finally, a lack of regulatory guidance and stakeholder participation can contribute to fears of cumulative impacts if numerous projects are within a single viewshed.
Based on the few studies that have been conducted, it appears that despite low public acceptance during the project-proposal phase, acceptance levels generally have increased following construction (Thayer and Hanson 1989; Wolsink 1990; Palmer 1997). It is possible to find communities that identify their local wind projects as tourist attractions. Part of the positive image many people hold is linked to wind energy’s “green image” and spe-
| Currently (late 2006), the most common commercial turbines being installed in the United States are 1.5 MW machines, usually 65-80 meters tall to the center of the rotor with rotor diameters of around 70 meters. The material in this chapter applies to turbines of this size. At several sites in the United States, 2.5 MW turbines are being used but are not yet in wide-spread use. |
cifically to its potential for replacing CO 2 -emitting electricity sources, with the hopeful prospect of reducing air pollution and global warming.
When evaluating the visual impacts of wind-energy projects, the essential question is not whether people will find them beautiful or not, but instead to what degree they may affect the important visual resources in the surrounding area. It is impossible to predict how any one individual will react to a wind-energy project. It is, however, possible to identify the visual character and scenic resources of a particular site and region. Evaluating the aesthetic impacts of wind-energy projects needs to focus on the relationship of the proposed project to the scenic landscape features of the site and its surrounding context. The factors that contribute to scenic quality can be identified and described with reasonable accuracy (Appleton 1975; Zube and Mills 1976; Litton 1979). This is especially true when viewing natural landscapes. Preferences are harder to predict for altered landscapes, although particular qualities of such landscapes have been identified in research of human preferences (Palmer 1983; Smardon et al. 1986). Nevertheless, we know enough to develop meaningful processes for reviewing aesthetic impacts. Despite the tremendous importance of a wind-energy project’s aesthetic impacts, especially on nearby residents, this issue is too often inadequately addressed.
Current Information
There is a growing body of information concerning the aesthetic impacts of wind-energy projects. The National Wind Coordinating Committee (NWCC) provides general outlines of aesthetic issues and some examples of local ordinances addressing wind-energy projects. The latter are very basic and do not address the broader issues of protecting particular landscape values. More comprehensive are the Proceedings of the NWCC Siting Technical Meeting (December 2005), which cover a range of relevant topics and provide a useful bibliography. The visual issues are addressed at length by Pasqualetti et al. (2002). While providing an excellent overview, that book predates the use of modern 1.5-3 MW turbines. And while it provides excellent guidance for mitigating impacts, it does not address siting or landscape characteristics. Research on public perceptions of specific wind-energy projects is fairly common in Europe (both pre- and post-construction studies), but there are fewer examples in the United States (Stanton 2005). Of those in the United States, most are focused on western landscapes (Thayer and Hansen 1989), while few are focused on eastern landscapes, including wooded ridgelines. While such studies are useful in understanding public reactions generally, visual impacts are largely site-specific (Pasqualetti 2005). Other available resources include legal and regulatory guidelines for review of wind-energy projects. New York’s State Environmental Quality Review Act (SEQRA) is one of the more explicit in the eastern United
States in terms of specifying what applicants need to submit and what will be considered (NYSDEC 2005; NYSERDA 2005a). Maine’s Department of Environmental Protection adopted similar language in its environmentalreview process (MEDEP 2003). In addition, there are several visual resource methods used for identifying scenic landscapes and for addressing visual impacts. Some important ones are discussed below.
Visual Assessment Methods
Two complementary approaches have been used to identify scenic resources and assess the impacts of proposed development projects. The first often is called a “professional approach” and relies on an individual or group with training in visual-resource and visual-impact assessment. These assessments rely on the research concerning human perceptions of landscapes (USFS 1979; Smardon et al. 1986) and on the adaptation of well-established methods for evaluating scenic landscape quality and for assessing visual impacts on particular landscapes. The second approach involves an assessment of public perceptions, attitudes, and values concerning a proposed project and its visual impacts on scenic resources. Landscapes are complex and imbued with cultural meaning that may not be understood by outside professionals. Techniques for assessing public perceptions, values, and attitudes include surveys, public meetings, interviews, and forums as well as examination of public documents identifying valued scenic resources (Smardon et al. 1986; Priestley 2006).
Among the best known and established methods for evaluating the scenic attributes of landscapes are the Visual Management System (USFS 1974) and the later Scenery Management System (USFS 1995) established by the U.S. Forest Service (USFS). Similarly, the U.S. Bureau of Land Management (BLM) uses a method called Visual Impact Assessment. The USFS and the BLM assessment methods have been used and adapted by numerous state and local agencies either for planning purposes (e.g., identifying scenic landscapes) or for assessing the impacts of proposed projects such as highways, ski areas, power plants, and forest harvesting (MADEM 1982; Smardon et al. 1986; RIDEM 1990).
While these methods are useful starting points, federal agencies such as the USFS usually go further in managing visual impacts on federal lands: they generally have plans in place that identify scenic values and set acceptable thresholds for alterations to the landscape. Even with detailed plans, these methods often fall short of providing meaningful guidance for evaluating the visual impacts of projects such as wind-energy facilities.
Most wind-energy projects are proposed on private land where there is far less guidance, especially with respect to evaluating aesthetic impacts. Many regulatory requirements adopted by states focus only on the tools for understanding the visibility of projects and fail to describe how visual im-
pacts should be evaluated. In other words, most processes are not very successful in addressing questions of what landscape or project characteristics would make a project aesthetically unacceptable or the impacts “undue.”
Below we outline a process for evaluating the conditions under which the aesthetic impacts of a proposed wind project might become unacceptable or “undue” in regulatory terms.
An Assessment Process for Evaluating the Visual Impacts of Wind-Energy Projects
The following steps summarize a process for moving from collecting measurable and observable information about visibility and landscape characteristics to analyzing the significance and importance of the visual resources involved and the effects of the proposed project on the landscape character and scenic resources of the surrounding area. Finally and most important, this process helps to inform the regulatory process about whether a proposed project is acceptable as designed, potentially acceptable with appropriate mitigation techniques, or unacceptable. The steps outlined below are described in greater detail in Appendix D .
Project Description
All site alterations that will have potential visual impacts must be identified by the developer in detail. These should include the turbine characteristics (height, rotor diameter, color, rated noise levels, proposed lighting) as well as the number of turbines and their locations; meteorological towers; roads; collector, distribution, and transmission lines; permanent and temporary storage “laydown” areas; substations; and any other structures associated with the project. In addition, all site clearings should be identified, including clearings for turbines, roads, power lines, substations, and laydown areas. All site regrading should be presented in sufficient detail to indicate the amount of cut and fill, locations, and clearing required. This information forms the basis for the visual assessment.
Project Visibility, Appearance, and Landscape Context
Viewshed mapping, photographic and virtual simulations, and field inventories of views are useful tools for determining with reasonable accuracy the visibility of the proposed project and for describing the characteristics of the views as well as identifying distinctive features within views (see Appendix D for more detail). Viewshed maps show areas of potential project visibility based on digital-elevation modeling. The modeling also can be used to determine the number of turbines that would be visible from a par-
ticular viewpoint. Actual visibility must be field-verified as trees, buildings, and other objects may restrict views. Field inventories also are necessary to document descriptive characteristics of the view. Inventories normally focus on areas of public use within a 10-mile radius of a project ( Box 4-1 ). These include public roads, recreation areas, trails, wilderness and natural areas, historic sites, village centers, and other important scenic or cultural features identified in planning documents or in public meetings.
Photomontages or simulations provide critical project information for analysis. They should most usefully illustrate visually sensitive viewpoints and a range of perspectives and distances. They should also illustrate “worst-case” conditions to the greatest extent possible (clear weather and leaf-off conditions). Excellent software is available for creating simulations, but the technical requirements for accuracy should be clearly understood and specified (see Appendix D ).
Identifying impacts from private residences can be more difficult without entering private property. Viewshed mapping can identify potential visibility. Geographic Information System (GIS) data generally provide additional information concerning existing vegetation and structures along with their primary use (residence, camp, or business). Providing regular notices to residents within a certain distance of the project can offer a means of learning more about visibility from private properties.
The size of the area for analysis may vary from location to location depending on the particular geography of the area and on the size of the project being proposed. Modern wind turbines of 1.5-3 MW can be seen in the landscape from 20 miles away or more (barring topographic or vegetative screening), but as one moves away from the project itself, the turbines appear smaller and smaller, and occupy an increasingly small part of the overall view. The most significant impacts are likely to occur within 3 miles of the project, with impacts possible from sensitive viewing areas up to 8 miles from the project. At 10 miles away the project is less likely to result in significant impacts unless it is located in or can be seen from a particularly sensitive site or the project is in an area that might be considered a regional focal point. Thus, a 10-mile radius provides a good basis for analysis including viewshed mapping and field assessment for current turbines. In some landscapes a 15-mile radius may be preferred if highly sensitive viewpoints occur at these distances, the overall scale of the project warrants a broader assessment, or if more than one project is proposed in an area. In the western United States, landscape scale and visibility may require a larger area of assessment. |
Scenic Resource Values and Sensitivity Levels
Some landscapes are more visually sensitive than others due to such factors as numbers of viewers, viewer expectations, and identified scenic values. Processes exist for determining the relative visual quality of landscapes, the features that contribute to visual quality, and the sensitivity levels of particular landscape features and their uses. These are outlined in Appendix D and also can be found in methods used by the USFS Visual Management System (USFS 1974) and its later Scenery Management System (USFS 1995). Scenic resources values can also be determined in public planning documents and through public meetings.
Assessment of Visual Impacts
Visual impacts vary considerably depending on the particular characteristics of the project and its landscape context. Visibility of a project is only one of many variables that should be examined. Significant visual impacts generally arise because of the combination of many factors such as proximity of views, sensitivity of views, duration of views, the presence of scenic resources of statewide or national significance, and the scale of the project in relation to its setting (see Appendix D ). Some examples of potentially significant impacts might include the following:
The project is located within a scenic context and is viewed in close proximity, for an extended duration (e.g., broad area or linear miles) from a highly sensitive use area, especially one for which the enjoyment of natural scenery is important, and that is an identified resource of statewide or national significance.
The project is located on a landform that is an important focal point that is highly visible throughout the region.
The project is of a scale that would dominate views throughout a region (or 10-mile assessment area) so that few other scenic natural views would be possible without including turbines.
Mitigation Techniques
A well-designed project will incorporate a number of techniques into the planning and design of the project to minimize visual impacts, including sensitive siting and ensuring that project infrastructure is well screened from view. Establishing “Best Practice” Guidelines can help ensure that minimum standards are met before project permit applications are submitted. Nevertheless, a thorough review by interested parties may result in further adjustments. If the visual impacts are deemed unacceptable, additional
mitigation techniques can be explored (see Appendix D ). In some cases, however, mitigation techniques may not solve inherent concerns, and the project may be found to have “undue aesthetic impacts.”
Determination of Unacceptable or Undue Aesthetic Impacts
Guidance on when projects may be found unacceptable tends to be lacking or inadequate in many review processes. The information gathered in the above process can inform this decision by providing a detailed understanding of the particular issues involved in the visual relationship between the project and its surrounding context. Appendix D provides questions that could help determine the degree of visual impact.
Among the factors to consider are:
Has the applicant provided sufficient information with which to make a decision? These would include detailed information about the visibility of the proposed project and simulations (photomontages) from sensitive viewing areas. New York’s SEQRA process offers an example of clearly identifying the information required and the mitigation measures that need to be considered.
Are scenic resources of local, statewide, or national significance located on or near the project site? Is the surrounding landscape unique in any way? What landscape characteristics are important to the experience and visual integrity of these scenic features?
Would these scenic resources be significantly degraded by the construction of the proposed project?
Would the scale of the project interfere with the general enjoyment of scenic landscape features throughout the region? Would the project appear as a dominant feature throughout the region or study area?
Has the applicant employed reasonable mitigation measures in the overall design and layout of the proposed project so that it fits reasonably well into the character of the area?
Would the project violate a clear, written community standard intended to protect the scenic or natural beauty of the area? Such standards can be developed at the community, county, region, or state level.
Guidelines for Protecting Scenic Resources
Planning and siting guidelines.
Siting guidelines that prospectively identify suitable and unsuitable locations for wind-energy projects have been considered in many regions. Problems with such guidelines arise, however. Each site is visually different,
local attitudes toward wind-energy development vary, and a wind developer must grapple with several non-aesthetic factors in locating a potentially developable site (e.g., willing property lessors, adequate wind resources, access to transmission lines, and a market for the electricity generated). Several combined approaches may be the most feasible. As discussed in more detail in Chapter 5 , they would include the following:
State and regional guidance providing criteria concerning site conditions that may be inherently suited or unsuited to wind development due to particular scenic values, and/or sensitivity levels that would raise concerns requiring additional detailed study. Policies regarding aesthetic conditions and wind development on state-owned lands would also be appropriate.
Local and state planning documents that identify valuable scenic, recreational, and cultural assets. Defining particular landscape attributes or other public values that contribute to the resources is helpful when making decisions concerning proposed landscape development proposals. 2 In addition, insofar as a “comprehensive plan” is voted on by the local governing body, the plan may provide guidance to a developer as an expression of the will of the community.
Statewide policies that address the relationship between the development of wind energy and the protection of valuable scenic resources.
Guidelines for Evaluating Cumulative Aesthetic Impacts
While wind-energy development is relatively new in the United States, the potential for cumulative aesthetic impacts resulting either from several new projects in a particular region or from expansion of existing projects is likely to become an issue that may need to be addressed at local, regional, and state levels. The following questions could help to evaluate the potential for undue cumulative aesthetic impacts:
| Clear and reasonably objective guidance is more useful than vague statements such as “the ridgelines in our town are valuable to our rural character and no development is allowed.” A statement that identifies the resource(s), its particular valued attributes, and appropriate and inappropriate development characteristics provides a clear written community standard. Statements that exclude wind development are generally not appropriate unless clear reasons are provided for this exclusion. For example, “the Town of Jonesville is characterized by the Green Range, which is composed of numerous hills and ridges. Several of the hills stand out because of their distinct shapes, including Mount Grant, Morris Mountain, and Jones Peak. Mount Grant is also valued for a popular hiking trail and the spectacular views looking west…” Such statements provide helpful guidance in decision making. In other words, a project located on another ridge but out of the view from the summit of Mount Grant might be acceptable, whereas a wind project located on Mount Grant probably would not be. |
Are projects at scales appropriate to the landscape context?
Are turbine types and sizes uniform within the wind resource area and over time?
How great is the offsite visibility of infrastructure?
Have areas that are inappropriate for wind projects due to terrain or important scenic, cultural, or recreational values been identified and described?
If the project is built as proposed, would each region retain undeveloped scenic vistas?
Would any one region be unduly burdened with wind-energy projects?
Considerations for Improving the Evaluation of Aesthetics and Implementation of Projects
Accurate and detailed information about the visual appearance of all aspects of a proposed project is extremely important. Incomplete or inaccurate information often results in public mistrust.
Generally, an area of 10 miles surrounding the project site is adequate for viewshed mapping and field assessment for turbines of a size currently used in the United States. In some landscapes, a 15- to 20-mile radius may be preferred, especially if highly sensitive viewpoints occur at these distances, the overall scale of the project warrants a broader assessment, or more than one project is proposed in an area.
In evaluating the aesthetic impacts of wind-energy projects, the discussion should focus not on whether people find wind-energy projects attractive but on the characteristics of the landscapes in which the projects will be located; the particular landscape features that contribute to scenic quality; the relative sensitivity of viewing areas; and the degree of degradation that would result to valued scenic resources, especially documented scenic values.
Computerized viewshed analyses provide useful information about potential project visibility but are best used as the basis for conducting field investigations. Within forested areas, views are likely to be minimal at best. The software allows more detailed analysis of numbers of turbines that can be seen from any one point.
Photomontages and photo simulations are essential tools in understanding project visibility, and appearance. Accurate representations involve exact technical requirements, such as precise camera focal lengths, Global Positioning System records of the photo location, and digital elevation (GIS-based) software. The technologies are changing, and it is important that simulations are accurately constructed (Stanton 2005). Local planning boards and the general public should be consulted in determining photo-
montage locations. They should illustrate sensitive or scenic viewpoints as well as “worst-case” situations such good weather conditions and the most scenic perspectives.
An independent assessment of visual impacts by trained professionals can provide more unbiased information than assessments provided on behalf of either developers or other interested and affected parties, and can provide useful comparisons with those assessments.
Meaningful public involvement is essential, and standards for providing information and opportunities for involvement can be helpful (see also Chapter 5 ).
Equally important are perceptions of clear benefits from wind-energy projects. Aesthetic perceptions are linked to our sense of general wellbeing. This has to do both with financial or material benefits (contributions to local taxes, payments for use of property, offsets such as protection of open space) and with making a real difference in terms of reducing pollution and CO 2 levels (Damborg 2002).
Towns, counties, regions, and states can provide helpful guidance to developers and decision makers by identifying landscape resources of value. This process is particularly useful when it is part of formally adopted documents such as comprehensive land-use plans, but it can also be used for developing guidelines.
Wind-energy projects will not necessarily conflict with areas of moderate to high scenic quality, and may even appear more attractive in these settings. Problems can arise when the setting is an important regional focal point, or when a project will be seen close to highly sensitive viewing areas where a natural or intact landscape is important.
The potential for cumulative impacts either from the location of several projects within a region, or from future expansions of existing projects, could become a problem. Cumulative impacts cannot be addressed at the project or local scale, and so a regional or statewide perspective is needed.
Scale is relative. The apparent size of a wind turbine in relation to its surrounding is most relevant. Despite their large sizes, modern wind turbines can fit well in many landscapes. Vertical scale is likely to be an issue primarily if the turbines appear to overwhelm an important ridgeline, focal point, or cultural feature that appears diminished in prominence due to the relative height of the turbines.
The number of turbines or horizontal scale of wind projects will be an important determination of reasonable fit within a region. A project that dominates views throughout a region is more likely to have aesthetic impacts judged unacceptable than one that permits other scenic or natural views to remain unimpaired throughout the region. If residences, especially those not directly benefiting from a proposed project, are surrounded by wind turbines, adverse aesthetic impacts are likely to be reported.
Visual clutter often is adversely perceived and commonly results from the combination of human-made elements in close association that are of differing shapes, colors, forms, patterns, or scales. Generally simple and uniform arrays or groupings of wind turbines are more visually appealing than mixed types and sizes. Screening of associated infrastructure also is important in reducing visual clutter.
Turbines with rotating blades have been shown to be more visually appealing than those that are still. Maintenance or removal of poorly functioning turbines can be important.
Turbine noise usually is most critical within a half-mile of a project. Efforts to reduce potential noise impacts on nearby residents therefore may be most important within that distance.
Decommissioning wind-energy projects appropriately would be considered in initial permit approvals. While some wind-energy projects may have longer life spans than originally anticipated, provisions are needed for removal of site structures that no longer contribute to the project, and for site restoration. Funding provided in escrow for decommissioning is sometimes essential.
Obstruction lighting required on objects more than 200 feet tall often is an extremely important aesthetic concern. Eliminating or reducing major lighting impacts merits a high priority.
CULTURAL IMPACTS
Wind-energy facilities create both positive and negative recreational impacts. On the positive side, many wind-energy projects are listed as tourist sights: some offer tours or provide information areas about the facility and wind energy in general; and several are considering incorporating visitor centers. Some developers allow open access to project sites that may provide additional opportunities for hunting, hiking, snowmobiling, and other activities.
There are two types of potential negative impacts on recreational opportunities: direct and indirect. Direct impacts can result when existing recreational activities are either precluded or require rerouting around a wind-energy facility. Indirect impacts include aesthetic impacts (addressed above) that may affect the recreational experience. These impacts can occur when scenic or natural values are critical to the recreational experience.
Most wind projects to date have been located on or proposed for private land. Policies vary regarding public use around wind turbines on both private and public lands. At project sites, access roads are often gated to
prevent public access along roads, but projects are not usually fenced from public use, although signage may discourage use.
Evaluating Recreational Impacts
In most cases, recreational uses will be identified in state and local documents and often on maps, although there may be times when recreational uses are only locally known. Some developers conduct recreation surveys to determine recreational uses in the study area and attitudes of users toward the development of wind-energy projects. Recreational concerns and interests are often identified in informal meetings and at public hearings. The USFS ranks recreational facilities as shown in Table 4-1 . This provides an example that may need to be adapted by states or local communities in evaluating the impacts of wind-energy facilities.
Most aesthetic and recreational-assessment methods identify relative “sensitivity levels” of recreational uses related to factors such as the amount of use and the expectations of users. A high sensitivity level does not necessarily mean that a wind-energy facility should not be visible, but instead is an indication that further study is needed. The USFS defines the following levels for evaluating impacts on USFS recreational experiences:
Sensitivity Level 1 areas (highly sensitive areas) include all areas seen from primary travel routes, use areas, and water bodies where a minimum of one-fourth of the forest visitors have a major concern for the scenic qualities. Areas specifically considered to be highly sensitive include roads providing access to highly sensitive recreation sites (i.e., sites where a natural environment, non-motorized use, and quiet are characteristic); National Scenic or Recreation Trails; heavily used seasonal trails through areas recognized as scenic attractions; significant recreational streams; water bodies with heavy fishing, boating, swimming, and other uses highly dependent on viewing scenery; wilderness and primitive areas; and observation sites along highly sensitive travelways.
TABLE 4-1 U.S. Forest Service Recreational Facilities Rankings
Primary Use Areas/Travel Routes | Secondary Use Areas/Travel Routes |
National importance | Local importance |
High use volume | Low use volume |
Long use duration | Short use duration |
Large size | Small size |
SOURCE: Adapted from Visual Management System (USFS 1974) and the later Scenery Management System (USFS 1995). |
Sensitivity Level 2 areas (“moderately sensitive locations”) include roads and trails on National Forest recreation maps that are not Level 1 or Level 3 and water bodies receiving low to moderate use.
Sensitivity Level 3 areas (least sensitive areas) include travelways constructed primarily for non-recreation purposes such as timber access roads and utility line clearings, and areas where uses primarily depend little on scenic viewing (e.g., hunting or gathering fuel wood, Christmas trees, or berries).
Historic, Sacred, and Archeological Sites
In analyzing impacts on historic, sacred, and archeological sites, the primary concern is that no permanent harm should be done that would affect the integrity of the site. Archeological inventories are generally required in most states before construction can begin. Some Native American tribes have sacred sites that may not be known to outsiders. Direct impacts (actual removal or physical harm) to historic, sacred, or archeological sites can be easily avoided in most instances.
Some states and localities have designated certain landscapes as having particular historical significance. For example, a proposed wind project in Otsego County, New York, that would have been located within the Lindesay Patent Historic District was later withdrawn. 3 Designation of a historic district provides a reasonable indication of historic value, uniqueness, and public concern for the resource. Whether or not a wind-energy project would damage the resource may depend on the specific nature of the historic resources involved.
The indirect effects on the experience of a historic or sacred site or area resulting from either seeing or hearing a wind-energy project nearby are not as well documented. Most historic sites are assumed to be part of evolving landscape contexts. Concerns generally would arise only when specific aesthetic or landscape attributes of the surrounding area are identified in the documentation of the site’s historic value. A setting where a multisensory experience has been re-created, such as at Plimoth Plantation in Massachusetts, might also warrant consideration. There, the visitor expects not just to see pre-revolutionary structures but to actually experience life at the time of the early settlers. A recent and currently unresolved case in Vermont concerned a historic Civilian Conservation Corps bath-house that was documented as having been sited to take advantage of scenic views down a lake where a proposed wind-energy facility would be visible. Unlike
| The proposed Global Harvest Wind Project was later withdrawn. Another currently proposed project would be visible from sensitive resources within a historic district, but a determination on that project has yet to be made. |
housing developments, wind-energy projects cannot be screened from view, except behind intervening topography and vegetation. Such issues are likely to arise as wind projects are proposed in cultural landscapes, and guidance as to what constitutes an undue impact to historic or sacred sites and areas will be necessary.
Evaluating Impacts on Historic, Sacred, and Archeological Sites
Historic, sacred, and archeological sites and settings must be regarded as sensitive sites. In most states, key historic sites are well documented and rated regarding their local, state, or national significance. State Offices of Historic Preservation, along with local historical societies, provide detailed information on historic sites and properties, and usually are involved in the review of proposed wind-energy projects. State archaeologists generally recommend specific guidelines for archaeological surveys, depending on the site involved. Archeological and sacred sites may be less well known. Documentation of these sites is essential. Good descriptive documentation will identify the particular values involved and the extent to which the context or setting contributes to the structure or landscape and in what way. Generally, the documentation of historic sites offers useful guidance to the value of the surrounding landscape to the interpretation of the resource, although the final determination probably should be done by experts. Most states are only now beginning to develop methods for reviewing onsite and offsite impacts of wind-energy facilities on historic sites (e.g., Vermont Division for Historic Preservation 2007). Siting wind-energy projects in the vicinity of identified and documented historic or sacred landscapes as well as historic, sacred, and archeological sites is likely to “raise red flags.” The impacts of viewing wind facilities from historic or sacred landscapes will require similar kinds of analyses to those noted in Appendix D for aesthetic impacts; however, additional guidance from relevant experts is needed in this area.
IMPACTS ON HUMAN HEALTH AND WELL-BEING
Wind-energy projects can have positive as well as negative impacts on human health and well-being. The positive impacts accrue mainly through improvements in air quality, as discussed previously in this report. These positive impacts (i.e., benefits) to health and well-being are diffuse; they are experienced by people living in areas where conventional methods of electricity generation are used less because wind energy can be substituted in the regional market.
In contrast, to the extent that wind-energy projects create negative impacts on human health and well-being, the impacts are experienced mainly
by people living near wind turbines who are affected by noise and shadow flicker.
As with any machine involving moving parts, wind turbines generate noise during operation. Noise from wind turbines arises mainly from two sources: (1) mechanical noise caused by the gearbox and generator; and (2) aerodynamic noise caused by interaction of the turbine blades with the wind. As described below (see “Noise Levels”), noise of greatest concern can be generally classified as being of one of these three types: broadband, tonal, and low-frequency.
The perception of noise depends in part on the individual—on a person’s hearing acuity and upon his or her subjective tolerance for or dislike of a particular type of noise. For example, a persistent “whoosh” might be a soothing sound to some people even as it annoys others. Nevertheless, it appears that subjective impressions of the noise from wind turbines are not totally idiosyncratic. A 1999 study (Kragh et al. 1999) included a laboratory technique for assessing the subjective unpleasantness of wind-turbine noise. Preliminary findings indicated that noise tonality and noise-fluctuation strength were the parameters best correlated with unpleasantness (Kragh et al. 1999).
Broadband, tonal, and low-frequency noise have all been addressed to some degree in modern upwind horizontal wind turbines, and turbine technologies continue to improve in this regard. With regard to the design of a wind-energy project, one is generally interested in assessing whether the additional noise generated by the wind turbines (relative to the ambient noise) might cause annoyance or a hazard to human health and well-being.
Noise impacts also can result from project construction and maintenance. These are generally of relatively short duration and occurrence but can include equipment operation, blasting, and noise associated with traffic into and out of the facility. These are not addressed in detail in this section. In the following, a brief review of wind-turbine noise and its impacts is presented along with suggested methods for assessing such impacts and mitigation measures.
Noise Levels
Noise from wind turbines, at the location of a receptor, is described in terms of sound pressure levels (relative to a reference value, typically 2 × 10 –5 Pa) and is typically expressed in dB(A), decibels corrected or A-weighted for sensitivity of the human ear. Note that there is a difference between sound power used to describe the source of sound and sound pres-
sure used to describe the effect on a receptor. The sound power level from a single turbine is usually around 90-105 dB(A); such a turbine creates a sound pressure of 50-60 dB(A) at a distance of 40 meters (this is about the same level as conversational speech). Noise (sound-pressure) levels from an onshore wind project are typically in the 35-45 dB(A) range at a distance of about 300 meters (BWEA 2000; Burton et al. 2001). These are relatively low noise or sound-pressure levels compared with other common sources such as a busy office (~60 dB(A)), and with nighttime ambient noise levels in the countryside (~20-40 dB(A)). While turbine noise increases with wind speed, ambient noises—for example, due to the rustling of tree leaves— increase at a higher rate and can mask the turbine noise (BLM 2005a).
In addition to the amplitude of the noise emitted from turbines, its frequency content is also important, as human perception of sounds is different at different frequencies. Broadband noise from a wind turbine typically is a “swishing” or “whooshing” sound resulting from a continuous distribution of sound pressures with frequencies above 100 Hz. Tonal noise typically is a “hum” or “pitch” occurring at distinct frequencies. Low-frequency noise (with frequencies below 100 Hz) includes “infrasound,” which is inaudible or barely audible sound at frequencies below 20 Hz.
Mechanical sounds from a turbine are emitted at “tonal” frequencies associated with the rotating machinery, while aerodynamic sounds are typically broadband in character. Mechanical noise is generated from rotating components in the nacelle, including the generator and gearbox, and to a lesser extent, cooling fans, pumps, compressors, and the yaw system. Aerodynamic noise, produced by the flow of air over blades, is created by blades interacting with eddies created by atmospheric inflow turbulence. This broadband aerodynamic noise is generally the dominant type of wind-turbine noise, and it generally increases with tip speed. Both mechanical and aerodynamic noise often are loud enough to be heard by people.
With older downwind turbines, some infrasound also is emitted each time a rotor blade interacts with the disturbed wind behind the tower, but it is believed that the energy at these low frequencies is insufficient to pose a health hazard (BWEA 2005). Nevertheless, a recent study by van den Berg (2004, 2006) suggests that, especially at night during stable atmospheric conditions, low-frequency modulation (at around 4 Hz) of higher frequency swishing sounds is possible. Note that this is not infrasound, but van den Berg (2006) states that it is not known to what degree this modulated fluctuating sound causes annoyance and deterioration in sleep quality to people living nearby.
Low-frequency vibration and its effects on humans are not well understood. Sensitivity to such vibration resulting from wind-turbine noise is highly variable among humans. Although there are opposing views on the subject, it has recently been stated (Pierpont 2006) that “some people feel disturbing amounts of vibration or pulsation from wind turbines, and can
count in their bodies, especially their chests, the beats of the blades passing the towers, even when they can’t hear or see them.” More needs to be understood regarding the effects of low-frequency noise on humans.
Guidelines for measuring noise produced by wind turbines are provided in the standard, IEC 61400-11: Acoustic Noise Measurement Techniques for Wind Turbines (IEC 2002), which specifies the instrumentation, methods, and locations for noise measurements. Wind-energy developers are required to meet local standards for acceptable sound levels; for example, in Germany, this level is 35 dB(A) for rural nighttime environments. Noise levels in the vicinity of wind-energy projects can be estimated during the design phase using available computational models (DWEA 2003a). Generally, noise levels are only computed at low wind speeds (7-8 m/s), because at higher speeds, noise produced by turbines can be (but is not always) masked by ambient noise.
Noise-emission measurements potentially are subject to problems, however. A 1999 study involving noise-measurement laboratories from seven European countries found, in measuring noise emission from the same 500 kW wind turbine on a flat terrain, that while apparent sound power levels and wind speed dependence could be measured reasonably reliably, tonality measurements were much more variable (Kragh et al. 1999). In addition, methods for assessing noise levels produced by wind turbines located in various terrains, such as mountainous regions, need further development.
Mitigation Measures and Standards
Noise produced by wind turbines generally is not a major concern for humans beyond a half-mile or so because various measures to reduce noise have been implemented in the design of modern turbines. The mechanical sound emanating from rotating machinery can be controlled by sound-isolating techniques. Furthermore, different types of wind turbines have different noise characteristics. As mentioned earlier, modern upwind turbines are less noisy than downwind turbines. Variable-speed turbines (where rotor speeds are lower at low wind speeds) create less noise at lower wind speeds when ambient noise is also low, compared with constant-speed turbines. Direct-drive machines, which have no gearbox or high-speed mechanical components, are much quieter.
Acceptability standards for noise vary by nation, state, and locality. They can also vary depending on time of day—nighttime standards are generally stricter. In the United States, the U.S. Environmental Protection Agency only provides noise guidelines. Many state governments issue their own regulations (e.g., Oregon Department of Environmental Quality
2006), and local governments often enact noise ordinances. Standards of acceptability need to be understood in the context of ambient (background) noise resulting from all other nearby and distant sources.
Shadow Flicker
As the blades of a wind turbine rotate in sunny conditions, they cast moving shadows on the ground resulting in alternating changes in light intensity. This phenomenon is termed shadow flicker. Shadow flicker is different from a related strobe-like phenomenon that is caused by intermittent chopping of the sunlight behind the rotating blades. Shadow flicker intensity is defined as the difference or variation in brightness at a given location in the presence and absence of a shadow. Shadow flicker can be a nuisance to nearby humans, and its effects need to be considered during the design of a wind-energy project.
In the United States, shadow flicker has not been identified as causing even a mild annoyance. In Northern Europe, on the other hand, because of the higher latitude and the lower angle of the sun, especially in winter, shadow flicker can be a problem of concern.
Shadow flicker is a function of several factors, including the location of people relative to the turbine, the wind speed and direction, the diurnal variation of sunlight, the geographic latitude of the location, the local topography, and the presence of any obstructions (Nielsen 2003). Shadow flicker is not important at distant sites (for example, greater than 1,000 feet from a turbine) except during the morning and evening when shadows are long. However, sunlight intensity is also lower during the morning and evening; this tends to reduce the effects of shadows and shadow flicker. The speed of shadow flicker increases with wind-turbine rotor speed.
Shadow flicker may be analytically modeled, and several software packages are commercially available for this purpose (e.g., WindPro and GH WindFarmer). An online tool for simple shadow calculations for flat topography is also available (DWEA 2003b). These software packages generally provide conservative results as they typically ignore the numerous influencing factors listed above and only consider a worst-case scenario (i.e., no shadow or full shadow). Inputs to a shadow-flicker model in WindPro, for example, include a description of the turbine and site, the topography, the joint wind speed and wind direction distribution, and an average or distribution of sunshine hours. Typical output results include the number of shadow-hours per year; these are often represented by iso-lines or contours of equal annual shadow-hours on a topographical map. Daily and
annual shadow variations may also be a part of the result (DWEA 2003b). A typical result might indicate, for example, that a house 300 meters from a 600 kW wind turbine with a rotor diameter of 40 meters will be exposed to moving shadows for approximately 17-18 hours annually, out of a total of 8,760 hours in a year (Andersen 1999).
Shadow flicker can be a nuisance to people living near a wind-energy project. It is sometimes difficult to work in a dwelling if there is shadow flicker on a window. In addition to its intensity, the frequency of the shadow flicker is of importance. Flicker frequency due to a turbine is on the order of the rotor frequency (i.e., 0.6-1.0 Hz), which is harmless to humans. According to the Epilepsy Foundation, only frequencies above 10 Hz are likely to cause epileptic seizures. (For reference, frequencies of strobe lights used in discotheques are higher than 3 Hz but lower than 10 Hz.) If a turbine is close to a highway, the movement of the large rotor blades and possible resulting flicker can distract drivers. Irish guidelines, for example, recommend that turbines be set back from the road at least 300 meters (MSU 2004).
Mitigation Measures
Shadow flicker is not explicitly regulated. When a maximum number of hours of allowed shadow flicker per year is imposed for a neighbor’s property (such as 30 hours/year for one wind-energy project in Germany), this number refers to those hours when the property is actually used by the people there and when they are awake. Denmark has no legislation regarding shadow flicker, but it is generally recommended that there be no more than 10 hours per year when flicker is experienced.
Even in the worst situations, shadow flicker only lasts for a short time each day—rarely more than half an hour. Moreover, flicker is observed only for a few weeks in the winter season. To avoid even limited periods of shadow flicker, a possible solution is to not run the turbines during this time. Obviously, another solution is to site the turbines such that their shadow paths avoid nearby residences.
Since tools for estimation of shadow flicker are readily available, such calculations are routinely done while planning a wind-energy project. One such study was performed for the Wild Horse project in the state of Washington (Nielsen 2003). Using results presented in the form of shadow flicker maps and distributions, one can determine suitable locations for wind turbines. Recently, tools have become available (GH WindFarmer) that not only compute shadow flicker in real time during turbine operation, but also convey information to the turbine control system to enable shutdown if the
shadow flicker at a particular location becomes particularly problematic. However, the committee is unaware if such real-time systems have been implemented at any specific wind-energy project.
LOCAL ECONOMIC AND FISCAL IMPACTS
Wind-energy projects can have a range of economic and fiscal impacts, both positive and negative. Some of those impacts are experienced at the national or regional level, as discussed in Chapter 2 . These involve, for example, tax credits and other monetary incentives to encourage wind-energy production, as well as effects of wind energy on regional energy pricing. In this section, the focus is on the local level: on private economic impacts, positive and negative, as well as on public revenues and costs.
Lease and Easement Arrangements
As discussed in Chapter 5 , most of the onshore wind-energy projects in the United States have been sited on private land. Typically, the developer of a wind-energy project acquires rights to the use of land through negotiations with landowners. Rarely is land purchased in fee simple; instead, the developer purchases leases or easements for a specified duration. While a uniform offer may be made to landowners, contract prices may be negotiated individually and privately. The power of eminent domain is not available to non-government wind-energy developers.
According to the American Wind Energy Association (AWEA 2006f), leasing arrangements can vary greatly, but a reasonable estimate for a lease payment to a landowner from a single utility-scale turbine is currently about $3,000 per year. Lease and easement arrangements can be a financial boon to landowners, providing a steady albeit modest income, but only if the financial and other contractual terms are fair.
A number of guides are now available for landowners who are considering either lease arrangements or granting easements for wind-energy projects. Some of these, such as the guides of the Wind Easement Work Group of Windustry, located in Minnesota, have been prepared by collaborations of wind-energy industry, government, and other partners (Nardi and Daniels 2005a). This work group has provided extensive guidance addressing such questions as:
How much of my land will be tied up and for how long?
What land rights am I giving up? What activities can I continue?
How much will I be paid and how will I receive payments?
Are the proposed payments adequate now and will they be adequate in the future?
Does the proposed method of payment or the agreement itself present adverse tax consequences to me?
Are there firm plans to develop my land, or is the developer just trying to tie it up?
If payments are to be based on revenues generated by the wind turbines, how much information is the developer willing to disclose concerning how the owners’ revenue will be determined?
What rights is the developer able to later sell or transfer without my consent?
Does the developer have adequate liability insurance?
What are the developer’s termination rights?
What are my termination rights?
If the agreement is terminated either voluntarily or involuntarily, what happens to the wind-energy structures and related facilities on my land?
Policies to Protect the Parties Involved
In a companion document, Windustry’s Wind Easement Work Group issued a short set of best practices and policy recommendations regarding easements and leases (Nardi and Daniels 2005b). These included:
Public disclosure of energy production from wind turbines: In order to facilitate transparency for production-based payments, increase public knowledge about the wind resource, and provide information to the state on the economic contribution of wind power.
Public filing of lease documents and public disclosure of terms (or include a “no gag” clause): In order to reduce competition among neighbors, encourage developers to give fair deals, and lower the possibility of a single holdout among landowners.
Limiting easement periods to 30 years and option periods to 5 years: To avoid tying up either the landowners or the developer for unduly long periods.
Property Values
It has been claimed that wind-energy projects do not adversely affect property values (Associated Press 2006). In contrast, it has been asserted that “adverse impacts on environmental, scenic and property values are often overlooked” (Schleede 2003, p. 1).
It is difficult to generalize about the effects of wind-energy projects on property values. A 2003 Renewable Energy Policy Project (REPP) study of the effect of wind development on property values found no statistical effects of changes in property values over time from wind-energy projects (Sterzinger et al. 2003). This study examined changes in property values within 5 miles of 10 wind-energy projects that came online between 1998 and 2001, looking at the three-year period before and after each project came online and using a simple linear-regression analysis. The study found no major pre-post differences, and it also found no major differences when property-value changes in the 5-mile areas around the wind-energy projects were compared with selected “comparable communities.”
The REPP study, however, examined only average price changes. The authors noted that “it would be desirable in future studies to expand the variables incorporated into the analysis and to refine the view shed in order to look at the relationship between property values and the precise distance from development” (Sterzinger et al. 2003, p. 3). A 2006 study (Hoen 2006) more closely examined the effects on property values between 1996 and 2005 within 5 miles of a 20-turbine, 30-MW project in Madison County, New York. This study used a hedonic regression analysis method and found no measurable effects on property values, positive or negative, even on residences within a mile of the facility. In contrast, a 2005 analysis by the Power Plant Research Program of the Maryland Department of Natural Resources concerning a proposed wind energy facility—the Roth Rock facility in Garrett County, Maryland—concluded that the facility would have an uncertain impact on the property values of neighboring properties. It reached this conclusion after reviewing the 2003 REEP study as well as a 2004 study in the United Kingdom by the Royal Institution of Chartered Surveyors (RICS), which found negative impacts, especially on non-farm properties (RICS 2004), and after analyzing the property-value impacts of the Allegheny Heights (Clipper) wind-energy project located north of the Roth Rock project and permitted in 2003 (MDDNR 2006).
Property values are affected by many variables. Thus, empirically isolating the impacts of one variable (a wind-energy project) is extremely difficult unless one or more turbines are located close to a specific property, and even then, there may be confounding factors. Forecasts of property values in prospective host areas that are based on comparisons with existing host areas are of questionable validity, especially if there are significant differences between the areas.
Despite the difficulty of reaching widely generalizable conclusions about the effects of wind-energy projects on property values, it is possible to theo-
rize about important variables. The discussion of aesthetic impacts earlier in this chapter is relevant. On the one hand, to the extent that a property is valuable for a purpose incompatible with wind-energy projects, such as to experience life in a remote and relatively untouched area, a view that includes a wind-energy project—especially one with many turbines—may detract from property values. On the other hand, to the extent that the wind-energy project contributes to the prosperity of an area, it may help to bring in amenities and so may enhance property values.
Because wind installations in the United States are a relatively recent phenomenon and are only now beginning to burgeon, the long-term effects of wind-energy projects on property values also are difficult to assess. While property values may be initially affected by a wind-energy project, the effect may diminish as the project becomes an accepted part of the landscape. On the other hand, the effects on local and regional property values of a few projects with 20 to 50 turbines may be quite different from the effects of numerous projects with 100 to 200 turbines.
When siting facilities that provide a public benefit but may be undesirable as neighbors, one mitigation measure that has been explored, for example, with waste facilities, is to provide property-value guarantees to property owners within a specified distance from the facility when they want to sell their properties (Zeiss and Atwater 1989; Smith and Kunreuther 2001). An issue in this arrangement is the fair level of the guaranteed selling price, as adjusted over time by an inflation factor.
Employment and Secondary Economic Effects
A wind-energy project is a source of jobs throughout its life cycle: for parts manufacturers and for researchers seeking to improve wind-turbine performance; for workers who transport and construct wind turbines and related infrastructure; for workers employed in the operation and maintenance of turbines, transmission lines, etc.; and for workers involved in project decommissioning. The number, skill and pay level, and location of the jobs will vary depending upon the scale, location, and stage of the project. Some of the jobs may be in the area that will host the wind turbines; some may be in a manufacturing plant several states away. At all locations, in addition to direct employment impacts, employment may be indirectly fostered through secondary economic effects, including indirect impacts (e.g., changes in inter-industry purchasing patterns) and induced impacts (e.g., changes in household spending patterns).
In addition, however, it is conceivable that a wind-energy project will
have some adverse impacts on the economy of its host area. While it has been argued that wind-energy facilities can be a tourist attraction (AusWEA 2004), it also has been argued that wind projects are seen by people as undesirable in national forest areas (Grady 2004) and can damage tourism in areas of high scenic beauty (Schleede 2003). It is also possible that, while one or a few wind-energy facilities may be a tourist attraction, a proliferation may have the reverse effect.
According to the AWEA’s “Wind Energy and Economic Development: Building Sustainable Jobs and Communities,” the European Wind Energy Association has estimated that in total, every MW of installed wind capacity directly and indirectly creates about 60 person-years of employment and 15 to 19 jobs. The fact sheet notes that the rate of job creation will decline as the industry grows and is able to take advantage of economies of scale (AWEA 2006f).
Of greatest interest at the local level, however, are not these totals but rather the jobs that become available to local or regional workers because of a wind-energy project in their vicinity. These jobs are likely to involve site preparation and facility construction during the project start-up period; skeleton crews for facility, grounds, and transmission line maintenance during facility operation typically about 20 years; and crews to perform decommissioning and site restoration work when the facility is closed.
The size of crews will vary depending upon the project scale, site characteristics, etc., but estimates of the number of employees, pay scales, skill requirements, and duration of employment can be made with reasonable accuracy. The secondary effects of wind-energy projects on the economy (both positive and negative) are much harder to estimate. On the one hand, a wind-energy project may increase the need for service sector businesses and jobs (gas stations, motels, restaurants, etc.). On the other hand, it may deter economic growth that would otherwise occur in the area (e.g., second homes, recreational facilities, and related amenities).
To estimate the secondary effects of a wind-energy project on a region’s economy, the region first must be geographically defined. Changes in its economic activity generally are then measured in terms of changes in either (1) employment, including part-time and seasonal employment; (2) regional income, i.e., the sum of worker wages and salaries plus business income and profits; or (3) changes in sales or spending. A regional economic multiplier may be used to estimate the secondary economic effects of new money flowing into the region. In conducting the impact analysis, the aim is to estimate the changes that would occur if the project is built versus if it is not built (not just the before/after changes).
While regional economic models have been available for some time, they generally are not well suited to assessing the secondary economic impacts of a single project on a small region or area. Recently, however, an economic model was developed specifically to estimate the economic benefits from a new wind-energy facility. This model, which was developed for the National Renewable Energy Laboratory (NREL), is called JEDI (Jobs and Economic Development Impacts). JEDI is an input-output model that calculates the direct, indirect, and induced economic benefits from new wind-energy facilities. (A new JEDI model, JEDI II, estimates the local economic benefits from new coal and natural gas facilities as well.) JEDI II uses input data such as the size of the project, its plant-construction cost, the length of the construction period, and fixed and variable operation and maintenance costs to estimate impacts (direct, indirect, and induced) in terms of jobs, wage and salary income, and output (economic activity) both during the construction period and during the operating years (Goldberg et al. 2004).
Models such as JEDI can improve understanding of the economic impacts of new energy facilities, especially when those impacts are considered at the macro level. Similarly, assessments of the actual economic impacts of wind-energy facilities, in addition to forecasts of economic impacts, can improve our collective understanding of the economic benefits of wind-energy facilities and how those benefits are distributed. Surveying 13 studies of economic impacts (actual and forecast) of wind facilities on rural economies, one NREL report concluded that these facilities have a large direct impact on the economies of rural communities, especially those with few other supporting industries; however, such communities also see greater “leakage” of secondary economic effects to outside areas. In addition, the report concluded that the number of local construction and operations jobs created by the facility depends on the skills locally available (Pedden 2006).
More studies are needed of the economic impacts of wind facilities, both actual and estimated. The NWCC (2001) provides these guidelines for assessing the economic development impacts of wind energy:
The audience for the study and the objectives to be pursued should receive primary consideration.
The assumptions and scenarios used to analyze economic development impacts should be clearly stated.
The model used to calculate impacts should use regional economic input data. The data should be representative of the study region (country, state, county, reservation, or multiple states and counties).
Both the potential positive and negative (i.e., displacement) economic impacts of wind-energy development should be considered.
The evaluation should consider the ownership, equity and sources of capital, and markets for the project for their relative impacts on the local community, reservation, state, region, or county.
The evaluation should consider the timing and scale of the project in relation to other wind-energy development in the state, region, or country. Pioneering projects in new areas face economic considerations different from those of incremental projects in mature wind-resource areas.
The evaluation should distinguish between short-term and longterm impacts.
The evaluation should consider relative impacts on the economy at a level appropriate to the scope of the study.
For both wind development and the displaced alternative, the evaluation should consider how new labor, material, and services would be supplied.
These guidelines are apt but demanding. From the perspective of the local affected area, it may be best to focus on the jobs that will be directly created by the project—what skills they require, what their pay levels are, what their duration will be, and what the company’s hiring practices are— as well as on reasonably anticipated effects—positive and negative—on the local economy.
Employment Commitments
A developer seeking to be favorably received by a host area may explore with local officials the possibility of a commitment to give hiring preferences to local workers. As Pedden (2006) noted in a report on the economic impacts of wind facilities in rural communities, “some local governments offer incentives to developers in return for the developer agreeing to hire local labor.”
Public Revenues and Costs
Like other industries, a wind-energy project generates tax dollars for the local government. According to the AWEA,“Wind Energy and Economic Development: Building Sustainable Jobs and Communities” (AWEA 2006f):
Alameda County, in California, collected $725,000 in property taxes in 1998 from wind-turbine installations valued at $66 million.
240 MW of wind capacity installed in Iowa in 1998 and 1999 produced $2 million annually in tax payments to counties and school districts.
The director of economic development in Lake Benton, Minnesota, said that each 100 MW of wind development generates about $1 million annually in property-tax revenue.
In addition, as with the private economy, the wind-energy project may indirectly generate taxes for the local government. However, as discussed above with regard to the private economy, an assessment of fiscal benefits in the form of tax revenue should be based on changes that would occur if the project was built versus if it was not built. The project may encourage some forms of economic development that generate taxes, but it may deter others.
A wind-energy facility also may entail public costs. Some of these, such as improvements of local public roads accessing the facility, will be obvious. Others, such as improved community services that may be expected in the wake of the development, will be indirect and less obvious. Taken together, the costs to a small, rural government have the potential to be significant.
Fiscal Commitments
The developer and the local government should have a clear mutual understanding of both the basis for tax revenues and what public expenditures are expected to make the project possible.
ELECTROMAGNETIC INTERFERENCE
Through electromagnetic interference (EMI), wind-energy projects conceivably can have negative impacts on various types of signals important to human activities: television, radio, microwave/radio fixed links, cellular phones, and radar.
EMI is electromagnetic (EM) disturbance that interrupts, obstructs, or otherwise degrades or limits the effective performance of electronics or electrical equipment. It can be induced intentionally, as in some forms of electronic warfare, or unintentionally, as a result of spurious emissions and responses and intermodulation products. In relation to wind turbines, two issues are relevant: (1) possible passive interference of the wind turbines with existing radio or TV stations, and (2) possible electromagnetic emissions produced by the turbines.
There are several ways in which electromagnetic waves can deviate from their intended straight-line communication paths. These include:
Blocking the path with an obstacle, thus creating a “shadow” or area where the intended EM wave will not occur. To a large extent, the
“blocking” depends on the size of the obstacle as a function of the wave-length of the electromagnetic wave.
Refraction of the EM wave. Refraction is the turning or bending of any wave, such as a light or sound wave, when it passes from one medium into another with different refractive properties.
In the context of wind-energy projects, EMI often is discussed in relation to the following telecommunications facilities:
Television broadcast transmissions (approx 50 MHz-1 GHz)
Radio broadcast transmissions (approx 1.5 MHz [AM] and 100 MHz [FM])
Microwave/radio “fixed links” (approx 3-60 GHz)
Mobile phones (approx 1 or 2 GHz)
The main form of interference to TV transmission caused by wind-energy projects is the scattering and reflection of signals by the turbines, mainly the blades. In relation to the components that make up a wind turbine, the tower and nacelle have very little effect on reception (i.e., only a small amount of blocking, reflection, and diffraction occurs). This is backed up by laboratory measurements that show that the tower introduces only a small, localized (up to approximately 100 m) attenuation of the signal (Buckley and Knight Merz 2005).
The British Broadcasting Corporation has issued recommendations based on a simple concept for calculating the geometry associated with reflected signals from wind turbines and how directional receiving aerials can provide rejection of the unwanted signals (BBC 2006).
Typical mitigation requirements include:
Re-orientation of existing aerials to an alternative transmitter
Supply of directional aerials to mildly affected properties
Switch to supply of cable or satellite television (subject to parallel broadcast of terrestrial channels)
Installation of a new repeater station in a location where interference can be avoided (this is more complex for digital but also less likely to be required for digital television)
Available literature indicates that effects of wind projects on both Amplitude Modulated (AM) and Frequency Modulated (FM) radio transmission systems are considered to be negligible and only apply at very small distances from the wind turbine (i.e., within tens of meters). For AM transmissions, this is due to low broadcast frequencies and long (100+ meter) signal wavelengths, which makes distortion difficult even for very large wind turbines. For FM transmissions, this is due to the fact that ordinary FM receivers are susceptible to noise interference only while operating in the threshold regions relative to signal-to-noise ratios. Thus, a distorted audio signal may be superimposed on the desired sound close to a wind turbine, potentially causing interference, only if the primary FM signal is weak.
Fixed Radio Links
Fixed radio links, also known as point-to-point links, are by definition a focused radio transmission directed at a specific receiver. Fixed links are not intended to be picked up by any receivers other than those at which they are directed. They typically rely on the use of a parabolic reflector antenna (like satellite dishes) to transmit a direct narrow beam of radio waves to a receiving antenna. A direct line of sight is required between the transmitter and receiver, and any obstructions within the line of sight may degrade the performance or result in the loss of the link.
A wind turbine may degrade the performance of a fixed link, not only if it is within the line of sight of the link but also if it is within a certain lateral distance of the link, known as the “Fresnel Zone.”
Cellular Phones
Mobile-phone reception depends greatly on the position of the mobile receiver. Therefore, the movement of the receiver and the topography—including both natural and unnatural obstacles—have a major impact on the quality of the signal. The mere movement of the receiver can ensure that wind turbines will have a very minimal effect, if any, on communication quality.
The potential for interference of wind turbines with radar is only partially understood. If there is such interference, it would primarily af-
fect military and civilian air-traffic control. In addition, National Weather Service weather radars might be affected.
Two recent reports treated the problems in some detail. The first is a report by the U.S. Department of Defense to the U.S. Congressional Defense Committees (DOD 2006). The second is a British report on the impacts of wind-energy projects on aviation radar (Poupart 2003).
The DOD report concludes that “[w]ind farms located within radar line of sight of air defense radar have the potential to degrade the ability of the radar to perform its intended function. The magnitude of the impact will depend upon the number and locations of the turbines. Should the impact prove sufficient to degrade the ability of the radar to unambiguously detect objects of interest by primary radar alone this will negatively influence the ability of U.S. military forces to defend the nation.” It concludes further that “[t]he Department has initiated research and development efforts to develop additional mitigation approaches that in the future could enable wind turbines to be within radar line of sight of air defense radars without impacting their performance.”
The U.K. report focused on the development and validation of a computer model that can be used to predict the radar reflection characteristics, which are a function of the complex interaction between radar energy and turbines. These effects are described by the Radar Cross Section (RCS). The report concludes that the model enables a much more detailed quantification of the complex interaction between wind turbines and radar systems than was previously available. Among the findings are:
Wind-turbine towers and nacelles can be designed to have a small RCS.
Blade RCS returns can be effectively controlled only through the use of absorbing materials (stealth technology).
The key factors influencing the effect of wind-energy facilities on radar are spacing of wind turbines within a facility, which needs to be considered in the context of the radar cross-range and down-range resolutions.
No optimal layout or format can be prescribed, because each wind-energy facility will have its own specific requirements that depend on many factors.
The report concludes that the model has a large potential for further use, such as the following:
It can generate the detailed data required for sophisticated initial screening of potential facility sites.
It can support the development of mitigation and solutions, in-
cluding siting optimization, control of wind-turbine RCS, and the development of enhanced radar filters that are able to remove returns from wind turbines.
It is clear that as of late 2006, the interference of wind turbines with radars is a problem as yet unsolved. Research and larger-scale investigations are currently under way in several countries; they may eventually lead to standardization and certification procedures.
CONCLUSIONS AND RECOMMENDATIONS
Aesthetic impacts.
Wind-energy facilities often are highly visible. Responses to proposed wind projects based on aesthetics are among the most common reasons for strong reactions to them. Reactions to the alteration of places that contribute to the beauty of our surroundings are natural and should be acknowledged. Excellent methods exist for identifying the scenic resource values of a site and its surroundings, and they should be the basis for visual impact assessments of proposed projects. Tools are available for understanding project visibility and appearance as well as the landscape characteristics that contribute to scenic quality. Lists of potential mitigation measures are also readily available. Nevertheless, the difficult step of determining under what circumstances and why a project may be found to have undue visual impacts is still poorly handled by many reviewing boards. The reasons include a lack of understanding of visual methods for landscape analysis and a lack of clear guidelines for decision making.
Current Best Practices
Information concerning best practices in the United States is found through the NWCC and its sponsored proceedings and links. Europe and Canada generally have done a more thorough job in providing definitive best-practice guidelines. The integration of local, regional, and national planning and review efforts in those countries contributes to the success of their review processes. Funding in those nations for planning and more extensive surveys of public perceptions of wind energy is also far ahead of that in the United States. Here, standards for best practices are evolving as communities and states recognize the need for a more systematic approach to evaluating visual impacts. There is considerable variability in the review of proposed projects.
Information Needs
Processes for evaluating the aesthetic impacts of wind-energy projects should be developed with a better understanding of the aesthetic principles that influence people’s experience of scenery. Comparative studies are needed of wind-energy projects that have relatively widespread acceptance of their aesthetic impacts and those that do not. These studies could provide useful information about a range of factors that contribute to acceptability within different landscape types. These studies should take into account that sites and projects vary dramatically in the types of scenic resources involved; the proximity and sensitivity of views; and the particular project characteristics, including scale.
The tradeoffs between placing wind-energy projects close to population centers where they are closer to electricity users but visible to more people, and placing them in remote areas where they are less visible but where the wilderness, remote, and undeveloped qualities of the landscape may hold value need discussion as well as a clear understanding of the tradeoffs involved. These issues need to be addressed broadly, not only singling out aesthetic concerns.
Impacts on Recreational, Historic, Sacred, and Archeological Sites
Wind-energy projects can be compatible with many recreational activities, but concerns may arise when they are close to recreational activities for which the enjoyment of natural scenery is an important part of the experience. Historic, sacred, and archeological resources can be harmed by direct impacts that affect the integrity of the resource or future opportunities for research and appreciation. The experience of certain historic or sacred sites or landscapes can also be indirectly affected by wind-energy projects, especially if particular qualities of the surrounding landscape have been documented as important to the experience, interpretation, and significance of the proximate historic or sacred site. Greater clarity is needed about how such situations should be evaluated. For example, the importance and special qualities of the experience must be assessed within the context of the relative visibility and prominence of the proposed wind-energy project.
Useful methods exist for evaluating both the relative sensitivity of recreational areas and recreational users, and for determining valuable scenic resources. Siting to avoid impacts on highly sensitive recreational uses, and project design to mitigate both direct and indirect impacts can be important. Mitigation techniques can include relocation of project design
elements, relocation of recreational activities (such as a trail), and enhancement of existing recreational activities.
State Historic Preservation Offices (SHPOs) generally identify all known historic sites of state and national significance. Local historical societies or comprehensive plans may identify additional sites of local significance. The SHPO typically requires a Class II survey to determine the existence of unknown resources in areas where such surveys are lacking. Guidelines for evaluating direct impacts on historic sites and structures often are available, and many states require archeological surveys for certain sites. Few guidelines currently exist, however, for evaluating indirect impacts of wind-energy projects on historic or sacred sites and landscapes.
Research examining the perceptions of recreational users toward wind-energy projects that are located near dispersed and concentrated recreational activities would provide useful data for decision makers. However, aesthetic impacts are very site-specific, so the results of such research likely will be able to guide site-specific assessments but not substitute for them.
Guidelines are needed concerning distances at which recreational activities can occur safely around wind turbines.
Policy makers and decision makers need better guidance from historic-preservation experts and others concerning the methods for evaluating the effects of wind-energy projects on historic, sacred, and archeological resources.
Noise and Shadow Flicker
Noise can be monitored by various measurement techniques. However, an important issue to consider, especially when studying noise, is that its perception and the degree to which it is considered objectionable depend on individuals exposed to it.
Shadow flicker caused by wind turbines can be an annoyance, and its effects need to be considered during the design of a wind-energy project. In the United States, shadow flicker has not been identified as even a mild annoyance. In Northern Europe, because of the higher latitude and the lower angle of the sun, especially in winter, shadow flicker has, in some cases, been noted as a cause for concern.
Best (or Good) Practices
Good practices for dealing with the potential impacts of noise from a wind-energy project could include the following:
Analysis of the noise should be made based on the operating characteristics of the specific wind turbines, the terrain in which the project will be located, and the distance to nearby residences.
Pre-construction noise surveys should be conducted to determine pre-project background noise levels and to determine later on what, if any, changes the wind project brought about.
If regulatory threshold levels of noise are in place, a minimum distance between any of the wind turbines in the project and the nearest residence should be maintained so as to reduce the sound to the prescribed threshold.
To have a process for resolving potential noise complaints, a telephone number should be provided through which a permitting agency can be notified of any noise concern by any member of the public. Then, agency staff can work with the project owner and concerned citizens to resolve the issue. This process can also include a technical assessment of the noise complaint to ensure its legitimacy.
Shadow flicker is reasonably well understood. With a little careful planning and the use of available software, the potential for shadow flicker can be assessed at any site, and appropriate strategies can be adopted to minimize the time when it might be an annoyance to residents nearby.
Recent research studies regarding noise from wind-energy projects suggest that the industry standards (such as the IEC 61400-11 guidelines) for assessing and documenting noise levels emitted may not be adequate for nighttime conditions and projects in mountainous terrain. This work on understanding the effect of atmospheric stability conditions and on sitespecific terrain conditions and their effects on noise needs to be accounted for in noise standards. In addition, studies on human sensitivity to very low frequencies are recommended.
Computational tools have become available that not only compute shadow flicker in real time during turbine operation, but also convey information to the turbine-control system to allow shutdown if the shadow flicker at a particular location becomes particularly problematic. Hence, the development and implementation of a real-time system at a wind-en-
ergy project to take such actions when shadow flicker is indicated might be useful.
Local Economic and Fiscal Impacts
When assessing the economic and fiscal impacts of a wind-energy project, the main issues that arise include (1) fair treatment of both landowners who lease land for the project and other affected but uncompensated owners and occupants; (2) a fine-grained understanding of how wind-energy facilities may affect property values; (3) a realistic appraisal of the net economic effects of the wind-energy facility, during its construction and over its lifetime; and (4) a similarly realistic assessment of the revenues the local government can expect and the costs it will have to assume.
The guidelines referred to in the text—of Windustry, regarding leasing and easement arrangements; and of the NWCC, regarding assessments of economic development impacts of wind power—contain good advice and are examples of current standards for best practices. In addition, best practices include:
Gathering as much “hard” information as possible: the terms of the lease and easement arrangements; the type, pay scale, and duration of jobs that are likely to be generated for local workers; the taxes that the project will directly generate; and the known public costs that it will entail.
Qualitatively taking into account other, less tangible economic factors: opportunity costs that may arise from the project; the duration of benefits from the project; and the likelihood of an uneven distribution of benefits (e.g., one landowner may realize income by leasing land for a turbine while another may be within close range of the turbine but receive no income).
Adopting guarantees and mitigation measures that are tailored to the facility, the surrounding community members, and the local government and are fair to all involved.
Large wind-energy facilities are fairly new in the United States. Many current analyses of their economic impacts are fueled by enthusiasm or skepticism. There is a need for systematic collection and analysis of economic data on a facility-by-facility and region-by-region basis. These data should take into account the type of facility, including the number of tur-
bines at the facility and elsewhere in the region. The data should cover the following types of information:
Leasing arrangements
Jobs directly created (including skill and pay levels, duration, hiring policies)
Local government revenue and costs
Economic mitigation and enhancement measures
More studies also are needed of public attitudes toward specific wind-energy facilities and how they affect economic behavior (e.g., property values, tourism, new residential development). To allow for cross-facility and longitudinal comparisons, the methods of data collection and analysis used in these studies should be replicable.
Electromagnetic Interference
With the exception of radar, the main EMI effects of wind-energy projects are well understood. Wind turbines have the potential to cause interference to television broadcasts, while the audio parts of TV broad-casts are less susceptible to interference. The data available are adequate to predict interference effects and areas and to minimize interference at the planning stage or propose suitable mitigation requirements.
Regarding radar, more research is needed to understand the conditions under which wind turbines can interfere with radar systems and to develop appropriate mitigation measures.
In addition, while EMI is not an issue in all countries (e.g., it is not an issue in Denmark), EMI issues should be given sufficient coverage in environmental impact statements and assessments to provide adequate evaluation of wind-energy project applications.
GENERAL CONCLUSIONS AND RECOMMENDATIONS
Well-established methods are available for assessing the positive and negative impacts of wind-energy projects on humans; these methods enable better-informed and more-enlightened decision making by regulators, developers, and the public. They include systematic methods for assessing aesthetic impacts, which often are among the most-vocalized concerns expressed about wind-energy projects.
Because relatively little research has been done on the human impacts of wind-energy projects, when wind-energy projects are undertaken, routine documentation should be made of processes for local interactions and impacts that arise during the lifetime of the project, from proposal through decommissioning. Such documentation will facilitate future research and therefore help future siting decisions to be made.
The impacts discussed in this chapter should be taken within the context of both the environmental impacts discussed in Chapter 3 and the broader contextual analysis of wind energy—including its electricity production benefits and limitations—presented in Chapter 2 . Moreover, the conclusions and recommendations presented by topic here should not be taken in isolation; instead, they should be treated as part of a process. Chapter 5 elaborates on processes for planning and evaluating wind-energy projects and for public involvement in these processes.
Finally, the text of this chapter describes many specific questions to be asked and issues to be considered in assessing various aspects of the effects of wind-energy projects on humans, especially concerning aesthetic impacts, and those questions and issues should be covered in assessments and regulatory reviews of wind-energy projects.
The generation of electricity by wind energy has the potential to reduce environmental impacts caused by the use of fossil fuels. Although the use of wind energy to generate electricity is increasing rapidly in the United States, government guidance to help communities and developers evaluate and plan proposed wind-energy projects is lacking. Environmental Impacts of Wind-Energy Projects offers an analysis of the environmental benefits and drawbacks of wind energy, along with an evaluation guide to aid decision-making about projects. It includes a case study of the mid-Atlantic highlands, a mountainous area that spans parts of West Virginia, Virginia, Maryland, and Pennsylvania. This book will inform policy makers at the federal, state, and local levels.
READ FREE ONLINE
Welcome to OpenBook!
You're looking at OpenBook, NAP.edu's online reading room since 1999. Based on feedback from you, our users, we've made some improvements that make it easier than ever to read thousands of publications on our website.
Do you want to take a quick tour of the OpenBook's features?
Show this book's table of contents , where you can jump to any chapter by name.
...or use these buttons to go back to the previous chapter or skip to the next one.
Jump up to the previous page or down to the next one. Also, you can type in a page number and press Enter to go directly to that page in the book.
Switch between the Original Pages , where you can read the report as it appeared in print, and Text Pages for the web version, where you can highlight and search the text.
To search the entire text of this book, type in your search term here and press Enter .
Share a link to this book page on your preferred social network or via email.
View our suggested citation for this chapter.
Ready to take your reading offline? Click here to buy this book in print or download it as a free PDF, if available.
Get Email Updates
Do you enjoy reading reports from the Academies online for free ? Sign up for email notifications and we'll let you know about new publications in your areas of interest when they're released.

COMMENTS
Miller and Keith repeated the calculation for solar power and found that its climate impacts were about 10 times smaller than wind's. "The direct climate impacts of wind power are instant, while the benefits of reduced emissions accumulate slowly," said Keith. "If your perspective is the next 10 years, wind power actually has — in ...
Wind energy is a small but fast-growing fraction of electricity production. It accounts for 5 percent of global electricity production and 8 percent of the U.S. electricity supply. Globally, wind energy capacity surpasses 743 gigawatts, which is more than is available from grid-connected solar energy and about half as much as hydropower can ...
For solar energy, the average power density (measured in watts per meter squared) is 10 times higher than wind power, but also much lower than estimates by leading energy experts. This research suggests that not only will wind farms require more land to hit the proposed renewable energy targets but also, at such a large scale, would become an ...
Wind energy in the United States helps avoid 336 million metric tons of carbon dioxide emissions annually. (link is external) —equivalent to the emissions from 73 million cars. Wind power benefits local communities. Wind projects deliver an estimated $2 billion. (link is external) in state and local tax payments and land-lease payments each year.
3. Wind power has a low operating cost. Because wind power is a renewable energy source, there is no ongoing expense to acquire fuel. Once the wind turbine is installed, the only real cost is maintenance. As the world decarbonizes electricity generation in the future, wind is a clean, renewable, and low-cost option. 4.
Harnessing power from the wind is one of the cleanest and most sustainable ways to generate electricity as it produces no toxic pollution or global warming emissions. Wind is also abundant, inexhaustible, and affordable, which makes it a viable and large-scale alternative to fossil fuels. Despite its vast potential, there are a variety of ...
1. Introduction. The increased interest in the effects of the generation of energy, together with the acknowledgement that our planet is an environment in a fragile balance, has focused on the search for sustainable energy technologies (Wang and Wang Citation 2015; Wang et al. Citation 2015).The burning of non-renewable energy sources is accepted as one of the primary sources of global warming ...
In two papers — published today in Environmental Research Letters and Joule — Harvard University researchers find that the transition to wind or solar power in the United States would require five to 20 times more land area than previously thought, and if such large-scale wind farms were built, would warm average surface temperatures over the continental United States by 0.24 degrees Celsius.
Wind energy is a form of renewable energy, typically powered by the movement of wind across enormous fan-shaped structures called wind turbines.Once built, these turbines create no climate-warming greenhouse gas emissions, making this a "carbon-free" energy source that can provide electricity without making climate change worse.Wind energy is the third-largest source of carbon-free ...
Wind explained: Wind energy and the environment
Open Document. Wind Energy and its Environmental Effects. Wind energy can play a critical role in saving our planet from the negative effects of energy powered by fossil fuels. Wind turbines work effectively at reducing carbon dioxide emissions. For instance, a single utility scale wind turbine can prevent the emission of 5,000 tons of CO2 into ...
Editor's note: The following essay is reprinted with permission from The Conversation, an online publication covering the latest research.. Wind turbines take energy from the atmosphere and turn ...
Researchers say that in many groups, misinformation is raising doubts about renewable energy and slowing or derailing projects. The opposition comes at a time when climate scientists say the world ...
Studies that project the impacts of wind power and/or other types of renewable energy on air quality and health often rely on reduced-complexity air quality approaches that simplify the relationship between emissions and the formation of atmospheric fine particulate matters (PM 2.5) and ozone (O 3).Millstein et al. used reduced-complexity atmospheric chemistry models to estimate cumulative air ...
The major advantage of wind turbines is that, taking into account their entire lifespan, they generate very low emissions compared with fossil fuels. Researchers often calculate greenhouse gas ...
The global benchmark levelized cost of electricity has been dropping for onshore wind energy, which was $44 per MWh in 2020, dropping 9 % from 2019 (Eckhouse, 2020).In addition, the global benchmark levelized cost of electricity for offshore wind energy dropped to $78 per MWh in 2020 from $90 per MWh in 2019 (Eckhouse, 2020).One of the drivers of wind energy generation is the energy policies.
Wind energy is a virtually carbon-free and pollution-free electricity source, with global wind resources greatly exceeding electricity demand. Accordingly, the installed capacity of wind turbines ...
Wind power offers a sustainable option in the pursuit of renewable energy. Wind is the movement of air from an area of high pressure to an area of low pressure. In fact, wind exists because the ...
The world faces two energy problems: most of our energy still produces greenhouse gas emissions, and hundreds of millions lack access to energy. The world lacks safe, low-carbon, and cheap large-scale energy alternatives to fossil fuels. Until we scale up those alternatives the world will continue to face the two energy problems of today.
The total electricity generated through the renewable energy sources was 244 terawatt-hours, which is under 40% of the total. Only 0.1 petawatt-hours or 21% of the aggregate electricity was produced from wind energy (British Petroleum, 2020). Hence, this has made the power sector to be a key source of the country's emissions.
Wind Energy - National Geographic Society ... Wind Energy
The global wind energy industry has been growing. Since 2005, the total installed capacity of global wind energy shows a 14% annualized growth rate for Asia, Europe and North America. Global wind energy electricity production expanded from 104 terawatt-hours (one trillion watts for one hour) in 2005 to 1,273 terawatt-hours in 2018, the paper said.
There are a number of reasons why proposed wind-energy projects evoke strong emotional reactions. Modern wind turbines are relatively new to the United States. Some of the early projects were built in remote areas, but increasingly, they are being built in or proposed for areas that are close to residential and recreational uses, and often in ...